Abstract
GV1001 is a peptide derived from the human telomerase reverse transcriptase (hTERT) sequence that is reported to have anti-cancer and anti-inflammatory effects. Enolase1 (ENO1) is a glycolytic enzyme, and stimulation of this enzyme induces high levels of pro-inflammatory cytokines from concanavalin A (Con A)-activated peripheral blood mononuclear cells (PBMCs) and ENO1-expressing monocytes in healthy subjects, as well as from macrophages in rheumatoid arthritis (RA) patients. Therefore, this study investigated whether GV1001 downregulates ENO1-induced pro-inflammatory cytokines as an anti-inflammatory peptide. The results showed that GV1001 does not affect the expression of ENO1 in either Con A-activated PBMCs or RA PBMCs. However, ENO1 stimulation increased the production of pro-inflammatory cytokines such as tumor necrosis factor (TNF)-α, interleukin (IL)-1β, and IL-6, and these cytokines were downregulated by pretreatment with GV1001. Moreover, p38 mitogen-activated protein kinase (MAPK) and nuclear factor (NF)-κB were activated when ENO1, on the surface of Con A-activated PBMCs and RA PBMCs, was stimulated, and they were successfully suppressed by pre-treatment with GV1001. These results suggest that GV1001 may be an effective anti-inflammatory peptide that downregulates the production of pro-inflammatory cytokines through the suppression of p38 MAPK and NF-κB activation following ENO1 stimulation.
GV1001 is a 16-amino acid peptide vaccine derived from the human telomerase reverse transcriptase (hTERT) sequence. It was developed as an anti-cancer agent to treat advanced pancreatic cancer, non-small lung cancer, melanoma, and other cancers (12345). Recent studies have reported that GV1001 penetrates cell membranes by binding with heat shock proteins (HSPs) and accumulates in the cytoplasm (6). Additionally, this peptide protects against renal ischemia reperfusion injury (IRI) in mice by reducing acute inflammatory responses and decreasing the proportion of apoptotic cells that can cause renal injury (7). Although GV1001 has been shown to be safe in several phase I/II clinical trials in cancer patients (8), the mechanism of action as an anti-inflammatory agent has not been completely elucidated.
Enolase (ENO) is a glycolytic enzyme that degrades 2-phosphoglycerate to 2-phosphoenolpyruvate. It consists of three isoforms depending on its location; α-enolase (ENO1) is expressed in most tissues while β-enolase (ENO3) is found in muscles and γ-enolase (ENO2) is found in the brain. ENO1 is ubiquitous in the cytosol in normal conditions; however, it is expressed on cell surfaces in pathological conditions like inflammation and cancer. Surface ENO1 acts as a plasminogen-binding receptor (91011), which promotes plasminogen-mediated recruitment of monocytes that induce acute inflammation in the lung. Pneumonia patients exhibit elevated levels of ENO1 on the surface of PBMCs and have intense ENO1 staining of mononuclear cells in the alveolar compartment (12). Many studies have reported that ENO1 expression increases on cell surfaces in response to various stimuli, and that ENO1 is involved in many aspects of an inflammatory response. Lipopolysaccharide (LPS) stimulation increased the translocation of ENO1 from the cytosol to the cell surface in primary monocytes and the human monocytic cell line U937 (12). The stimulation of ENO1 on hematopoietic cells, such as neutrophils, lymphocytes, and monocytes, with phorbol myristate acetate (PMA) increased the cells' capacities to generate plasmin (1314). In our previous study, Con A stimulation increased the expression of ENO1 on the surface of PBMCs and consequently increased the production of pro-inflammatory cytokines (15). The inflammatory response in Con A-activated PBMCs by ENO1 stimulation is recognized as similar to the response in PBMCs from rheumatoid arthritis (RA) patients. There are many reports that ENO1 antibodies play pathogenic roles in a variety of autoimmune and inflammatory diseases, such as systemic lupus erythematous, systemic sclerosis, Behcet's disease, ulcerative colitis, Crohn's disease, retinopathy, and RA (161718). RA is a representative autoimmune disease that includes synovial inflammation, pannus formation, and subsequent bone destruction (19202122232425). Many cell types, such as monocytes and macrophages, infiltrate into the inflamed sub-synovium, and play key pathophysiological roles in RA (262728). Furthermore, these cells lead to continuous inflammation and exacerbate symptoms by upregulating the production of prostaglandin E2 (PGE2) and several cytokines, such as TNF-α, IL-1, IL-6, and interferon (IFN)-γ (1529). This means that ENO1 exacerbates RA pathology (9). The expression of ENO1 on the surface of monocytes and macrophages in RA patients is higher than the expression in healthy controls. Higher ENO1 expression on RA PBMCs is correlated with the production of pro-inflammatory cytokines, mediated by the p38 MAPK and NF-κB pathways (15).
In the present study, we investigated whether GV1001 has an anti-inflammatory effect on an ENO1-induced inflammatory response, in terms of the down-regulation of pro-inflammatory cytokine production and its related mechanisms. We found that GV1001 down-regulates ENO1-induced TNF-α, IL-1, and IL-6 production from Con Aactivated PBMCs and PBMCs from the patients with rheumatoid arthritis (RA) via the regulation of p38 MAPK and NF-κB activation.
Anti-ENO1 mAb was separated and purified from the ascites of mice inoculated with F6 (a hybridoma-producing anti-ENO1 mAb) using a protein A column (Pierce, Grand Island, NY, USA). Ascitic fluid was diluted 1:1 with Protein A IgG Binding Buffer (Pierce, Grand Island, NY, USA) and centrifuged at 10,000 g for 20 min. After filtration with a 0.22-µm syringe filter, the diluted ascetic fluid was applied to the column and allowed to flow completely into the resin bed. Then, the column was flushed with 15 ml of binding buffer and 5 ml of elution buffer (Pierce, Grand Island, NY, USA) to elute antibodies. The concentration of separated antibodies was measured by bicinchoninic acid (BCA) assay. For the flow cytometric analysis, anti-ENO1 mAb was labeled with fluorescein isothiocyanate (FITC) using a FluroTag™ FITC conjugation kit (Sigma-Aldrich, St. Louis, MO, USA), and then FITC-conjugated anti-ENO1 mAb was diluted with PBS (1 mg/ml) and stored at 4℃.
Heparinized peripheral blood was obtained from healthy volunteers and RA patients under a protocol (#1506-029-678) approved by the Institutional Review Board (IRB) of Seoul National University Hospital. Blood was mixed with an equal volume of phosphate buffered saline (PBS), and then PBMCs were isolated by density gradient centrifuge using Ficoll-Paque PLUS (Amersham Pharmacia Biotech, Piscataway, NJ, USA). After centrifugation, the buffy coat was collected and washed twice with PBS. Red blood cells (RBCs) were lysed with RBC lysis buffer (Sigma, St. Louis, MO, USA) by shaking for 5 min at 37℃. After cells were washed with PBS twice, the cells were counted and cell purity was examined by flow cytometry. To induce ENO1 expression on the surface of PBMCs, isolated PBMCs were stimulated with Con A (2 µg/ml) and then cultured in complete RPMI1640 (Welgene, Korea) media containing 10% heat inactivated fetal bovine serum (GE Health care Hyclone, UT, USA) and antibiotics (100 U/ml of penicillin and 100 µg/ml streptomycin; Welgene, Korea) for 24 h. After Con A stimulation, PBMCs were washed with PBS twice and re-suspended in complete RPMI1640.
GV1001 was provided by KAEL-GemVax (Seongnam, Korea). GV1001 was dissolved in PBS for a 10 mM final concentration. After Con A-activated PBMCs and RA PBMCs (4×106/ml) were pre-treated with GV1001 (100 µM) for 1 h at room temperature with gentle rotation, cells were stimulated with anti-ENO1 mAb (1 mg/ml) for 1 h at room temperature with gentle rotation. MOPC21 (1 mg/ml; Sigma-Aldrich) was used as an isotype control. After ENO1 stimulation, cells were seeded onto 24-well plates and incubated at 37℃ in a humidified incubator with 5% CO2.
PBMCs (1×105 cells/each group) were re-suspended in PBS containing 0.5% BSA and 0.1% sodium azide (FACS buffer). After PBMCs were stained with FITC-conjugated anti-ENO1 mAb or the isotype control (BD Bioscience, San Jose, CA, USA) for 30 min at 4℃, cells were also stained with PE-conjugated anti-human CD69, CD25, CD3, CD14 antibodies (BD Biosciences) or the isotype control (Sigma-Aldrich). After washing with FACS buffer, ENO1 surface expression was analyzed by a FACS Calibur flow cytometer and FlowJo software (BD Biosciences). At least 10,000 cells were analyzed per condition.
After GV1001 pre-treatment and ENO1 stimulation, Con A-activated PBMCs were incubated for 48 h and RA PBMCs were incubated for 12 h. To identify the GV1001-related mechanism of cytokine expression, the specific inhibitors for p38 mitogen-activated protein kinase (MAPK) and nuclear factor κB (NF-κB) inhibitors, SB203580 and Bay11-7082, respectably, were purchased from Sigma. SB203580 (40 µM), Bay11-7082 (2.5 µM), GV1001 (100 µM), and dimethylsulfoxide(DMSO; vehicle control) were used to pre-treat PBMCs for 1 h, and then PBMCs were stimulated with anti-ENO1 mAb for 1 h. These cells were incubated for an additional 48 h and then supernatant was collected for ELISA. The levels of TNF-α, IL-1β (Biolegend, San Diego, CA, USA) and IL-6 (R&D systems, Minneapolis, MN, USA) in the supernatant were measured using specific ELISA kits according to the manufacturer's instructions. The relative absorbances were measured at 450 nm using SoftmaxPro software (Molecular Devices, Sunnyvale, CA, USA).
Cells (1×106) were lysed with lysis buffer containing 50 mM Tris-HCl (pH 7.4), 1% NP-40, 0.25% sodium deoxycholate, 150 mM NaCl, 1 mM EDTA, a protease inhibitor cocktail, and a phosphatase inhibitor cocktail (Sigma-Aldrich). The protein concentration was measured by BCA assay. Protein (10 µg/sample) from the lysed cells was separated by 10% SDS-PAGE for 4 h, and transferred onto a nitrocellulose membrane for 1 h. After blocking with 5% nonfat milk in PBS containing 0.05% Tween 20 (PBS-T) for 1 h at room temperature, the membrane was incubated with primary Ab at 4℃ overnight. Primary Abs for phospho-p38 MAPK (1:500; Cell Signaling, Danvers, MA, USA), p38 MAPK (1:1000; Santa Cruz, Dallas, TX, USA), phospho-p65 (1:500; Santa Cruz), p65 (1:1000; Santa Cruz), and β-actin (1:5000; Sigma) were diluted with 1% nonfat milk in PBS-T. After washing 3 times (5 min/each) with PBS-T, the membrane was incubated with horseradish peroxidase (HRP)-conjugated anti-rabbit IgG (1:5000; Cell Signaling) against phospho-p38 MAPK, p38 MAPK, phospho-p65 NF-κB, and p65 NF-κB, and mouse HRP-conjugated anti-mouse IgG (1:5000; Cell Signaling) against β-actin for 1 h at RT. The membrane was then washed 3 times (5 min/each) with PBST and EZ-Western Lumi La (Dogen, Daeill, Korea) solution was loaded to detect the signal. The density of bands was analyzed using ImageJ software (NIH, Bethesda, MD, USA). Results were expressed the relative intensities of each band adjusted to that of p38 MAPK or p65 NF-κB.
ENO1 is rarely expressed on the surface of inactivated PBMCs in healthy volunteers. However, Con A stimulation for 24 h increased ENO1 expression on PBMCs (Fig. 1A). In our previous study, the major immune cell population that expressed ENO1 by Con A stimulation are T cells and monocytes (15). We observed that ENO1 was expressed on T cells and monocytes in Con A-activated PBMCs (data not shown). Next, whether GV1001 could change Con A-induced ENO1 expression on the surface of PBMCs was investigated. After cells were treated with GV1001, before or after Con A stimulation, changes in the expression of ENO1 on cell surfaces were analyzed by flow cytometry. From the results, no remarkable changes in ENO1 expression between pre- and post-treatment with GV1001 (Fig. 1B and C) were found. Therefore, GV1001 does not affect Con A-induced ENO1 expression on the surface of PBMCs.
ENO1 stimulation is known to induce inflammation by increasing the production of pro-inflammatory cytokines (15). A recent report showed that GV1001 administration reduced the infiltration of macrophages in renal IRI in mice, and it suppressed the production of IL-6 and monocyte chemotactic protein (MCP)-1 (7). Thus, we examined whether the level of pro-inflammatory cytokines was increased by ENO1 stimulation with anti-ENO1 mAb, and whether GV1001 could suppress the production of pro-inflammatory cytokines in Con A-activated PBMCs following ENO1 stimulation. PBMCs were pre-treated with GV1001 for 1 h, and then the cells were simulated with anti-ENO1 mAb for another 1 h. After the cells were incubated for another 48 h, the levels of pro-inflammatory cytokines TNF-α, IL-1 β, and IL-6 were measured in the cell culture supernatants. When ENO1-expressing PBMCs were stimulated with anti-ENO1 mAb, the levels of TNF-α (anti-ENO1 Ab (617.6.1±7.7 pg/ml) vs. control (72.4±9.07 pg/ml) (p<0.001), IL-1β (anti-ENO1 Ab (3872.2±75.7 pg/ml) vs. control (3427.0±66.2 pg/ml) (p<0.01), and IL-6 (anti-ENO1 Ab (50.66±1.39 ng/ml) vs. control (32.77±0.72 ng/ml) (p<0.001) were dramatically increased (Fig. 2A-C). However, they were considerably reduced (TNF-α: GV1001+anti-ENO1 Ab (496.2±51.9 pg/ml) vs. anti-ENO1 Ab (617.6±7.7 pg/ml) (p<0.05); IL-1β: GV1001+anti-ENO1 Ab (3578.0±122.0 pg/ml) vs. anti-ENO1 Ab (3872.2±75.7 pg/ml) (p<0.05); and IL-6: GV1001+anti-ENO1 Ab (43.96±1.25 ng/ml) vs. anti-ENO1 Ab (50.66±1.39 ng/ml) (p<0.01) by the prewith GV1001 treatment (Fig. 2A-C). These results suggest that GV1001 suppresses the production of pro-inflammatory cytokines produced from Con A-activated PBMCs following ENO1 stimulation.
Because GV1001 suppressed the ENO1-induced production of TNF-α, IL-1β, and IL-6, as shown in Fig. 2, the signaling mechanism related to this suppression was examined next. Recently, we reported that ENO1 stimulation enhances the production of pro-inflammatory mediators through the activation of the p38 MAPK and NF-κB pathways (15). Therefore, we verified the possibility that GV1001 decreases the production of pro-inflammatory cytokines by disrupting the p38 MAPK and NF-κB signaling pathway. Con A-activated PBMCs were pre-treated with p38 MAPK inhibitor (SB203580), NK-κB inhibitor (Bay11-7082), and GV1001 for 1 h, and the cells were stimulated with anti-ENO1 mAb. After incubation for another 48 h, TNF-α, IL-1β, and IL-6 in the culture supernatants were measured by ELISA. As seen in a previous study, TNF-α, IL-1β, and IL-6 production was decreased by treatment with SB203580 and Bay11-7082. In addition, pre-treatment with GV1001 also suppressed ENO1-induced TNF-α, IL-1β and IL-6 production (Fig. 3A-C). Based on the results regarding the similar suppressive effect of GV1001 on ENO1-induced pro-inflammatory cytokine production the specific inhibitors for p38MAPK and NF-κB, we examined whether GV1001 could suppress the activation of p38MAPK and NF-κB by immunoblotting. As shown in Fig. 4, the phosphorylation of p38 (Fig. 4A and B) and p65 (Fig. 4C and D) was found to increase with ENO1 stimulation, which was suppressed by pre-treatment with GV1001. Therefore, it seems that GV1001 has an anti-inflammatory effect through the suppression of p38 MAPK and NF-κB activation in Con A-activated PBMCs, even though it is partially dependent.
Inflammatory responses in Con A-activated PBMCs induced by ENO1 stimulation are recognized to be similar to the inflammatory responses in RA, which is a systemic inflammatory autoimmune disease characterized by severe inflammation of the joints and destruction of bone and cartilage (272829). The expression level of ENO1 in PBMCs isolated from RA patients (RA PBMCs) was previously reported to be much higher than the level of ENO1 in PBMCs isolated from normal healthy individuals (normal PBMCs). Monocytes and macrophages, which are involved in severe acute inflammation, are major ENO1-positive immune cells (15). Therefore, the anti-inflammatory effect of GV1001 on RA PBMCs was confirmed, as it was in the Con A-stimulated PBMCs. Most RA PBMCs spontaneously upregulated ENO1 on their surfaces (Fig. 5A). Similar to a previous study, which reported that ENO1 is mostly expressed in the monocytes and macrophages of RA patients (15), we confirmed that most CD14+ cells expressed ENO1 on their surfaces (Fig. 5B). Because we observed that GV1001 does not affect Con A-induced ENO1 expression in normal PBMCs (Fig. 1D and E), whether GV1001 changes ENO1 expression on the surface of PBMCs from RA patients was also examined. The results showed that ENO1 expression on the CD14+ cells neither increased nor decreased with GV1001 treatment (Fig. 5B and C). This result shows that treatment with GV1001 does not change ENO1 expression in PBMCs isolated from RA patients. We have already shown that GV1001 decreased ENO1-induecd TNF-α, IL-1β, and IL-6 production from Con A-activated PBMCs (Fig. 2). We also found that GV1001 decreased ENO1-induecd TNF-α, IL-1β, and IL-6 production from RA PBMCs (Fig. 6).
GV1001 suppressed the induction of pro-inflammatory cytokines in Con A-activated PBMCs (Fig. 2). Next, the antiinflammatory effect of GV1001 was verified in RA PBMCs. RA PBMCs were incubated with GV1001 for 1 h and stimulated with anti-ENO1 mAb for 1 h. After another 12-h incubation, the cytokine levels in the culture supernatants were measured by ELISA. The amount of TNF-α (anti-ENO1 Ab (719.3±12.6 pg/ml) vs. control (34.9±21.9 pg/ml) (p<0.001), IL-1β (anti-ENO1 Ab (321.5±17.4 pg/ml) vs. control (36.9±2.7 pg/ml) (p<0.001), and IL-6 (anti-ENO1 Ab (2.45±1.31 ng/ml) vs. control (1.63±2.54 ng/ml) (p<0.001) drastically increased following ENO1 stimulation and diminished with GV1001 pre-treatment (TNF-α: GV1001+anti-ENO1 Ab (341.0±8.1 pg/ml) vs. anti-ENO1 Ab (719.3±12.6 pg/ml) (p<0.001); IL-1β: GV1001+anti-ENO1 Ab (224.3±7.6 pg/ml) vs. anti-ENO1 Ab (321.5±17.4 pg/ml) (p<0.001); and IL-6: GV1001+anti-ENO1 Ab (1.99±1.02 ng/ml) vs. anti-ENO1 Ab (2.45±1.31 ng/ml) (p<0.01). This result shows that GV1001 can alleviate robust inflammation by ENO1 stimulation in RA PBMCs.
As shown in Fig. 4, the activation of p38 MAPK and NF-κB following ENO1 stimulation was suppressed by GV1001 in Con A-activated PBMCs (Fig. 4). Next, whether GV1001 could downregulate the activation of p38 MAPK and NF-κB in PBMCs isolated from RA patients was examined. RA PBMCs were pre-treated with GV1001 for 1 h and stimulated by anti-ENO1 mAb for another 1 h. Then, phosphorylated p38 MAPK and p65 were examined by immunoblotting (Fig. 7A). A densitometric analysis showed that the increased phosphorylation of p38 MAPK and p65 following ENO1 stimulation was significantly decreased with GV1001 treatment in RA PBMCs (Fig. 7B and C). Therefore, GV1001 effectively disrupts the p38 MAPK and NF-kB signaling pathways and consequently suppresses the production of pro-inflammatory cytokines induced by ENO1 stimulation in not only Con A-activated PBMCs but also in RA PBMCs.
In the present study, we found that GV1001, derived from the human telomerase reverse transcriptase (hTERT) sequence, is an anti-inflammatory peptide that suppresses the production of pro-inflammatory cytokines and the activation of p38 MAPK and NF-κB induced by ENO1 stimulation in Con A-activated PBMCs and RA PBMCs.
RA is an autoimmune disease that affects up to 3% of the population (30). In the past few years, many researchers have tried to elucidate the causes of RA and to develop therapeutic approaches. However, the precise causes and related therapies for RA have not been completely elucidated. There are many factors that can worsen RA, including various cytokines such as TNF-α, IL-1, and IL-6 produced by cells such as monocytes and macrophages that infiltrated inflamed synovial joints (313233). Recently, the involvement of ENO1 in the pathogenesis of RA and its possible role as a therapeutic target were reported (15).
ENO1, an essential enzyme involved in glycolysis, is located in the cytoplasm in normal conditions, but it is translocated to the cell membrane in pathogenic conditions. Recent studies have shown that ENO1 is a putative target for auto-antigen in RA (16). Additionally, an increase in ENO1-positive cells induces the swift migration of inflammatory cells into lung inflammatory lesions (23). ENO1 was previously shown to be overexpressed in monocytes and macrophages from PBMCs and synovial fluid mononuclear cells (SFMCs) of RA patients (15). When the ENO1 translocated from the cytosol to the cell surface is stimulated, the production of PGE2 and pro-inflammatory cytokines such as TNF-α, IL-1α/β, IFN-γ, and IL-18 are increased. All of these cytokines are closely related to RA pathogenesis and promote inflammatory cell recruitment to inflamed synovial tissues. Therefore, investigations of the molecules that regulate the production of pro-inflammatory cytokines can provide clues for effective RA treatments.
GV1001 was first developed as a cancer vaccine (123), and it was subsequently revealed to function as an anti-inflammatory agent (7). In a mouse renal IRI model, this peptide efficiently inhibited the production of IL-6 and MCP-1, which are associated with a decrease in the infiltration of neutrophils and macrophages in the kidney after IRI. Furthermore, GV1001 interacts with various cytoplasmic proteins, including ENO1, for its mode of action (6). Hence, GV1001 showed potential to be used as an effective therapeutic agent for RA by regulating ENO1 activity. Fig. 6 shows the effect of GV1001 on TNF-α, IL-1β, and IL-6 production in RA PBMCs. This result suggests that GV1001 may be not only an effective therapeutic agent but also an adjuvant for conventional RA therapeutic drugs.
Several pro-inflammatory cytokines directly affect the pathogenesis of RA. In the case of TNF-α, an NF-κB activation cascade initiator induces the production of other pro-inflammatory cytokines through the NF-κB signaling pathway (34). NF-κB signaling provides positive regulation that may amplify and maintain RA pathology (34). IL-6 activates the Janus kinase/signal transducer and activator of transcription (JAK/STAT) pathway, and STAT-3 is constitutively activated in RA (3536). Inflammation is usually tightly regulated by mediators that initiate, maintain, and shut down the process. Efforts to develop more effective treatments for RA are based on an understanding of the cytokine network. Pro-inflammatory cytokines has been targeted with therapeutic antibodies or receptor antagonists to limit overproduction. For RA, many therapeutic agents, such as Tocilizumab and Infiximab, have been developed with this goal. These are immunosuppressive drugs, and each attenuates IL-6 and TNF-α-mediated signaling by blocking the cytokines and/or their receptors. Although these are effective inflammatory inhibitors, there are several adverse side effects, such as upper respiratory tract infections and serious infections of multiple other organs. However, GV1001 has been shown to be safe in several phase I/II clinical trials (373839). In the current study, Con A-activated PBMCs and RA PBMCs were shown to produce high levels of pro-inflammatory cytokines after ENO1 stimulation, and these cytokines were significantly decreased by treatment with GV1001 before ENO1 stimulation (Fig. 2 and 6). Therefore, GV1001 is capable of suppressing pro-inflammatory cytokines and a possible therapeutic agent that can reduce ENO1-mediated inflammation.
ENO1 is known to induce inflammation through activation of the p38 MAPK and NF-κB signaling pathways in RA (15). These signaling pathways have important roles in RA pathogenesis. NF-κB is a pivotal regulator of inflammation through regulation of transcription. It is closely involved in the development of T helper 1 (Th1) responses, activation of abnormal apoptosis, and proliferation of RA fibroblast-like synovial cells (404142). In addition, the p38 MAPK pathway contributes to the upregulation of proinflammatory cytokines, chemokines, and metalloproteinase in RA (43). As shown in Fig. 3, GV1001 effectively suppressed the ENO1-induced production of TNF-α, IL-1β, and IL-6, similar to p38 MAPK and NF-κB inhibitors in Con A-activated PBMCs. Moreover, increased activation of p38 MAPK and NF-κB, which was represented by the increased expression of phosphorylated p38 and p65, was effectively inhibited by treatment with GV1001 before ENO1 stimulation in both Con A-activated PBMCs and RA PBMCs (Fig. 4 and 7). This result suggests a regulatory role for GV1001 in the inflammation-related activation of p38 MAPK and NF-κB signaling mechanisms. Taken all together, these results suggest that GV1001 may be a useful anti-inflammatory peptide by downregulating pro-inflammatory cytokine production and regulation of p38 MAPK and NF-κB activation induced by ENO1 stimulation.
Figures and Tables
Figure 1
The effect of GV1001 on the increase in ENO1 expression following Con A stimulation. Con A-stimulated PBMCs were stained with (A) FITC-conjugated anti-ENO1 mAb. FITC-conjugated MOPC21 were used as the isotype controls. (B) PBMCs (1×106/ml) were pretreated with GV1001 (100 µM) or vehicle for 1 h and then cultured in the presence or absence of Con A (2 µg/ml) for 24 h. Then, the cells of each group were harvested, washed with PBS, and incubated for another 48 h. (C) PBMCs (1×106/ml) were stimulated with Con A (2 µg/ml) or vehicle for 24 h. Next, the cells were washed with PBS, treated with GV1001 (100 µM) for 1 h, and then incubated for another 48 h. ENO1 expression in these groups was analyzed by flow cytometry.
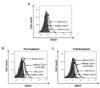
Figure 2
The inhibitory effect of GV1001 on ENO1-induced pro-inflammatory cytokine production in Con A-activated PBMCs. Activated PBMCs were pre-treated with GV1001 (100 µ M) for 1 h and then stimulated with anti-ENO1 mAb (1 µg) for 1 h. MOPC21 was used as an isotype control. Stimulated PBMCs were cultured in a 24-well plate (1×106/ml) for another 48 h. The culture supernatant of the stimulated PBMCs was collected, and (A) TNF-α, (B) IL-1β, and (C) IL-6 levels were measured by ELISA. Data are presented as the means±SD. *p<0.05, **p<0.01, ***p<0.001.
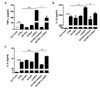
Figure 3
The regulation of p38 MAPK and NF-κ B activation by treatment with GV1001. Con A-activated PBMCs were pre-treated with GV1001 (100 µM), DMSO (vehicle), SB203580 (40 µM), and Bay11-7082 (2.5 µM) for 1 h and then stimulated with anti-ENO1 mAb for 1 h. After incubating for another 48 h, the supernatant was harvested, and pro-inflammatory cytokine levels (A) TNF-α, (B) IL-1β, and (C) IL-6 were determined by ELISA. Data are presented as the means±SD. ***p<0.001.
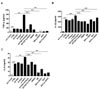
Figure 4
The suppression of p38 MAPK and NF-κB activation by treatment with GV1001. Con A-activated PBMCs were pre-treated with GV1001 (100 µM) for 1 h, and then stimulated with anti-ENO1 mAb for 120 min for p38 MAPK and 5 min for NF-κB. Then, the cells were collected and lysed with lysis buffer to extract proteins for immunoblotting. Levels of (A) the phosphorylated form of p38 (p-p38) and total p38 and (C) the phosphorylated form of p65 (p-p65) and total p65 were examined by immunoblotting. A densitometry analysis was performed and results are represented as the fold increase in the phosphorylated form over the total form of (B) p-p38/p38 and (D) p-p65/p65. Results are representative of three independent experiments. **p< 0.01, ***p<0.001.
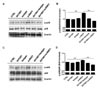
Figure 5
The effect of GV1001 on ENO1 expression in RA PBMCs. (A) PBMCs (1×105) from RA patients (n=10) were stained with anti-ENO1 mAb or the isotype control, and ENO1 expression was examined by flow cytometry. RA PBMCs were incubated in the (B) absence or (C) presence of GV1001 (100 µM) for 48 h. After washing, the cells were double-stained with FITC conjugated anti-ENO1 mAb and PE-conjugated anti-CD14 Ab.
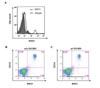
Figure 6
The effect of GV1001 on ENO1-induced pro-inflammatory cytokine production in RA PBMCs. RA PBMCs were pre-treated with GV1001 (100 µM) for 1 h and then incubated with anti-ENO1 mAb (1 µg) or MOPC21 isotype for 1 h. After the stimulated cells were seeded in a 24-well plate, they were further incubated for 12 h. The culture supernatant was collected, and the levels of (A) TNF-α, (B) IL-1β, and (C) IL-6 were measured by ELISA. Data are presented as the means±SD. **p<0.01, ***p<0.001.
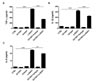
Figure 7
The suppression of p38 MAPK and NF-κB activation in RA PBMCs following treatment with GV1001. RA PBMCs were stimulated with anti-ENO1 mAb for 120 min after pre-treatment with GV1001 (100 µM) for 1 h. (A) Phosphorylation of p38 and p65 was detected by immunoblotting. The relative expression is presented as the fold increase of (B) p-p38/p38 or (C) p-p65/p65 by densitometry analysis. Results are representative of three independent experiments. ***p<0.001.
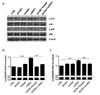
ACKNOWLEDGEMENTS
This work supported by Kael&Gemvax Ltd. (Grant #: 0620144530 (2014-3221)) to JSK and the overseas research program of Seoul National University Hospital to WJS.
References
1. Middleton G, Silcocks P, Cox T, Valle J, Wadsley J, Propper D, Coxon F, Ross P, Madhusudan S, Roques T, Cunningham D, Falk S, Wadd N, Harrison M, Corrie P, Iveson T, Robinson A, McAdam K, Eatock M, Evans J, Archer C, Hickish T, Garcia-Alonso A, Nicolson M, Steward W, Anthoney A, Greenhalf W, Shaw V, Costello E, Naisbitt D, Rawcliffe C, Nanson G, Neoptolemos J. Gemcitabine and capecitabine with or without telomerase peptide vaccine GV1001 in patients with locally advanced or metastatic pancreatic cancer (TeloVac): an open-label, randomised, phase 3 trial. Lancet Oncol. 2014; 15:829–840.


2. Staff C, Mozaffari F, Frodin JE, Mellstedt H, Liljefors M. Telomerase (GV1001) vaccination together with gemcitabine in advanced pancreatic cancer patients. Int J Oncol. 2014; 45:1293–1303.


3. Annels NE, Shaw VE, Gabitass RF, Billingham L, Corrie P, Eatock M, Valle J, Smith D, Wadsley J, Cunningham D, Pandha H, Neoptolemos JP, Middleton G. The effects of gemcitabine and capecitabine combination chemotherapy and of low-dose adjuvant GM-CSF on the levels of myeloid-derived suppressor cells in patients with advanced pancreatic cancer. Cancer Immunol Immunother. 2014; 63:175–183.


4. Brunsvig PF, Kyte JA, Kersten C, Sundstrom S, Moller M, Nyakas M, Hansen GL, Gaudernack G, Aamdal S. Telomerase peptide vaccination in NSCLC: a phase II trial in stage III patients vaccinated after chemoradiotherapy and an 8-year update on a phase I/II trial. Clin Cancer Res. 2011; 17:6847–6857.


5. Hunger RE, Kernland Lang K, Markowski CJ, Trachsel S, Moller M, Eriksen JA, Rasmussen AM, Braathen LR, Gaudernack G. Vaccination of patients with cutaneous melanoma with telomerase-specific peptides. Cancer Immunol Immunother. 2011; 60:1553–1564.


6. Lee SA, Kim BR, Kim BK, Kim DW, Shon WJ, Lee NR, Inn KS, Kim BJ. Heat shock protein-mediated cell penetration and cytosolic delivery of macromolecules by a telomerase-derived peptide vaccine. Biomaterials. 2013; 34:7495–7505.


7. Koo TY, Yan JJ, Yang J. Protective effect of peptide GV1001 against renal ischemia-reperfusion injury in mice. Transplant Proc. 2014; 46:1117–1122.


8. Greten TF, Forner A, Korangy F, N'Kontchou G, Barget N, Ayuso C, Ormandy LA, Manns MP, Beaugrand M, Bruix J. A phase II open label trial evaluating safety and efficacy of a telomerase peptide vaccination in patients with advanced hepatocellular carcinoma. BMC Cancer. 2010; 10:209.


9. Miles LA, Dahlberg CM, Plescia J, Felez J, Kato K, Plow EF. Role of cell-surface lysines in plasminogen binding to cells: identification of alpha-enolase as a candidate plasminogen receptor. Biochemistry. 1991; 30:1682–1691.


10. Redlitz A, Fowler BJ, Plow EF, Miles LA. The role of an enolase-related molecule in plasminogen binding to cells. Eur J Biochem. 1995; 227:407–415.


11. Lopez-Alemany R, Longstaff C, Hawley S, Mirshahi M, Fabregas P, Jardi M, Merton E, Miles LA, Felez J. Inhibition of cell surface mediated plasminogen activation by a monoclonal antibody against alpha-Enolase. Am J Hematol. 2003; 72:234–242.
12. Wygrecka M, Marsh LM, Morty RE, Henneke I, Guenther A, Lohmeyer J, Markart P, Preissner KT. Enolase-1 promotes plasminogen-mediated recruitment of monocytes to the acutely inflamed lung. Blood. 2009; 113:5588–5598.


13. Giallongo A, Feo S, Showe LC, Croce CM. Isolation and partial characterization of a 48-kDa protein which is induced in normal lymphocytes upon mitogenic stimulation. Biochem Biophys Res Commun. 1986; 134:1238–1244.


14. Felez J, Miles LA, Plescia J, Plow EF. Regulation of plasminogen receptor expression on human monocytes and monocytoid cell lines. J Cell Biol. 1990; 111:1673–1683.


15. Bae S, Kim H, Lee N, Won C, Kim HR, Hwang YI, Song YW, Kang JS, Lee WJ. alpha-Enolase expressed on the surfaces of monocytes and macrophages induces robust synovial inflammation in rheumatoid arthritis. J Immunol. 2012; 189:365–372.


16. Saulot V, Vittecoq O, Charlionet R, Fardellone P, Lange C, Marvin L, Machour N, Le Loet X, Gilbert D, Tron F. Presence of autoantibodies to the glycolytic enzyme alpha-enolase in sera from patients with early rheumatoid arthritis. Arthritis Rheum. 2002; 46:1196–1201.


17. Mosca M, Chimenti D, Pratesi F, Baldini C, Anzilotti C, Bombardieri S, Migliorini P. Prevalence and clinico-serological correlations of anti-alpha-enolase, anti-C1q, and antidsDNA antibodies in patients with systemic lupus erythematosus. J Rheumatol. 2006; 33:695–697.
18. Wakui H, Imai H, Komatsuda A, Miura AB. Circulating antibodies against alpha-enolase in patients with primary membranous nephropathy (MN). Clin Exp Immunol. 1999; 118:445–450.


19. Sweeney SE, Firestein GS. Rheumatoid arthritis: regulation of synovial inflammation. Int J Biochem Cell Biol. 2004; 36:372–378.


20. Tak PP, van der Lubbe A, Cauli A, Daha MR, Smeets TJ, Kluin PM, Meinders AE, Yanni G, Panayi GS, Breedveld FC. Reduction of synovial inflammation after anti-CD4 monoclonal antibody treatment in early rheumatoid arthritis. Arthritis Rheum. 1995; 38:1457–1465.


21. Ishikawa H, Hirata S, Andoh Y, Kubo H, Nakagawa N, Nishibayashi Y, Mizuno K. An immunohistochemical and immunoelectron microscopic study of adhesion molecules in synovial pannus formation in rheumatoid arthritis. Rheumatol Int. 1996; 16:53–60.


22. Furuzawa-Carballeda J, Macip-Rodriguez PM, Cabral AR. Osteoarthritis and rheumatoid arthritis pannus have similar qualitative metabolic characteristics and pro-inflammatory cytokine response. Clin Exp Rheumatol. 2008; 26:554–560.
23. Xue C, Takahashi M, Hasunuma T, Aono H, Yamamoto K, Yoshino S, Sumida T, Nishioka K. Characterisation of fibroblast-like cells in pannus lesions of patients with rheumatoid arthritis sharing properties of fibroblasts and chondrocytes. Ann Rheum Dis. 1997; 56:262–267.


24. Robinson DR, Tashjian AH Jr., Levine L. Prostaglandin-stimulated bone resorption by rheumatoid synovia. A possible mechanism for bone destruction in rheumatoid arthritis. J Clin Invest. 1975; 56:1181–1188.


25. Kotake S, Udagawa N, Hakoda M, Mogi M, Yano K, Tsuda E, Takahashi K, Furuya T, Ishiyama S, Kim KJ, Saito S, Nishikawa T, Takahashi N, Togari A, Tomatsu T, Suda T, Kamatani N. Activated human T cells directly induce osteoclastogenesis from human monocytes: possible role of T cells in bone destruction in rheumatoid arthritis patients. Arthritis Rheum. 2001; 44:1003–1012.


26. Van Boxel JA, Paget SA. Predominantly T-cell infiltrate in rheumatoid synovial membranes. N Engl J Med. 1975; 293:517–520.


27. Slauenwhite D, Gebremeskel S, Doucette CD, Hoskin DW, Johnston B. Regulation of cytokine polarization and T cell recruitment to inflamed paws in mouse collagen-induced arthritis by the chemokine receptor CXCR6. Arthritis Rheumatol. 2014; 66:3001–3012.


28. Brennan F, Beech J. Update on cytokines in rheumatoid arthritis. Curr Opin Rheumatol. 2007; 19:296–301.


29. Firestein GS. Starving the synovium: angiogenesis and inflammation in rheumatoid arthritis. J Clin Invest. 1999; 103:3–4.


30. Moore TL, Gillian BE, Crespo-Pagnussat S, Feller L, Chauhan AK. Measurement and evaluation of isotypes of anti-citrullinated fibrinogen and anti-citrullinated alpha-enolase antibodies in juvenile idiopathic arthritis. Clin Exp Rheumatol. 2014; 32:740–746.
31. Myasoedova E, Crowson CS, Kremers HM, Therneau TM, Gabriel SE. Is the incidence of rheumatoid arthritis rising?: results from Olmsted County, Minnesota, 1955-2007. Arthritis Rheum. 2010; 62:1576–1582.


32. Miossec P. An update on the cytokine network in rheumatoid arthritis. Curr Opin Rheumatol. 2004; 16:218–222.


33. Matsukawa A, Yoshimura T, Miyamoto K, Ohkawara S, Yoshinaga M. Analysis of the inflammatory cytokine network among TNF alpha, IL-1 beta, IL-1 receptor antagonist, and IL-8 in LPS-induced rabbit arthritis. Lab Invest. 1997; 76:629–638.
34. McInnes IB, Schett G. Cytokines in the pathogenesis of rheumatoid arthritis. Nat Rev Immunol. 2007; 7:429–442.


35. Jue DM, Jeon KI, Jeong JY. Nuclear factor kappaB (NF-kappaB) pathway as a therapeutic target in rheumatoid arthritis. J Korean Med Sci. 1999; 14:231–238.


36. Ivashkiv LB, Hu X. The JAK/STAT pathway in rheumatoid arthritis: pathogenic or protective? Arthritis Rheum. 2003; 48:2092–2096.
37. Isomaki P, Junttila I, Vidqvist KL, Korpela M, Silvennoinen O. The activity of JAK-STAT pathways in rheumatoid arthritis: constitutive activation of STAT3 correlates with interleukin 6 levels. Rheumatology (Oxford). 2015; 54:1103–1113.


38. Campbell L, Chen C, Bhagat SS, Parker RA, Ostor AJ. Risk of adverse events including serious infections in rheumatoid arthritis patients treated with tocilizumab: a systematic literature review and meta-analysis of randomized controlled trials. Rheumatology (Oxford). 2011; 50:552–562.


39. Nishimoto N, Kishimoto T. Humanized antihuman IL-6 receptor antibody, tocilizumab. Handb Exp Pharmacol. 2008; 151–160.


40. Cohen G, Courvoisier N, Cohen JD, Zaltni S, Sany J, Combe B. The efficiency of switching from infliximab to etanercept and vice-versa in patients with rheumatoid arthritis. Clin Exp Rheumatol. 2005; 23:795–800.
41. Makarov SS. NF-kappa B in rheumatoid arthritis: a pivotal regulator of inflammation, hyperplasia, and tissue destruction. Arthritis Res. 2001; 3:200–206.