Abstract
Macrophages are the main effector cells of innate immunity and are involved in inflammatory and anti-infective processes. They also have an essential role in maintaining tissue homeostasis, supporting tissue development, and repairing tissue damage. Until few years ago, it was believed that tissue macrophages derived from circulating blood monocytes, which terminally differentiated in the tissue and unable to proliferate. Recent evidence in the biology of tissue macrophages has uncovered a series of immune and ontogenic features that had been neglected for long, despite old observations. These include origin, heterogeneity, proliferative potential (or self-renewal), polarization, and memory. In recent years, the number of publications on tissue resident macrophages has grown rapidly, highlighting the renewed interest of the immunologists for these key players of innate immunity. This mini-review aims to summarizing the new current knowledge in macrophage immunobiology, in order to offer a clear and immediate overview of the field.
Innate immunity is the first line of defense of the body, present throughout evolution from lower (invertebrate) to more complex organisms (vertebrate) (1). In order to rapidly protect the host against infectious or stressful events, the innate immune cells initiate triggers an inflammatory response. When not properly regulated or in excess, inflammation may contribute to many and different pathological conditions, from autoimmune and chronic inflammatory diseases to atherosclerosis and cancer (234567). Monocytes/macrophages are the major effector cells of innate immunity. They play a pivotal role in the immune response to pathogens, by generating and then resolving the inflammatory reaction. But they also have a central role in tissue development (by shaping the tissue architecture), and in surveillance and monitoring of tissue changes (by acting as sentinel and effector cells). Especially, they are important in maintening tissue homeostasis, by clearing apoptotic or senescent cells, and by repairing and remodeling structural and functional integrity of the tissue soon after a damage.
In 1893, Elie Metchnikoff first described the phagocytosis of pathogens by macrophages ("the big eaters" in Greek), observing starfish challenged with rose thorns (8). Macrophages were identified as tissue resident cells able to eat and kill infectious agents. In 1924, macrophages were defined by Aeschoff as cells of the reticulo-endothelial system (RES). This implied that macrophages originate from, and reside and renew within, that tissue, which comprises the Kupffer cells of the liver, the cells lining the sinuses of spleen, lymph nodes, bone marrow, and the endothelial cells of various organs (9). In the late 1960s, Ralph Van Furth proposed the existence of the Mononuclear Phagocyte System. Within this system, all macrophages, including tissue macrophages and inflammatory cells (macrophages recruited during inflammation, also meaning the monocyte-derived macrophages), are terminally differentiated cells derived from blood monocytes (10). However, in 1984 Van Furth together with Diesselhoff-den Dulk (11) suggested that not all macrophages derive from blood monocytes. In the same years it was observed that macrophages were not terminally differentiated (1213) and were persistent in tissues (14). Recent evidence dramatically transformed our knowledge of macrophages biology. It has now become clear that tissue-resident macrophages may arise independently of monocyte input (see below), originating prenatally from c-myb-independent hematopoiesis (15) prior to the establishment of definitive hematopoiesis. Accordingly, there is now a consensus that some macrophages may proliferate, although it is not clear whether this takes place through self-renewal or through the proliferation of local progenitors (1617). Thus, in light of the monocyte-independent origin of macrophages, other aspects of macrophage biology need reassessment, including macrophages heterogeneity, the role of environment in the tissue-specific specialization, and macrophage polarization into different functional phenotypes during inflammation.
In this review we provide an overview of these new insights into tissue macrophages. Several questions are still open on differences, similarities, and development lineage relationship between monocyte-derived macrophages and tissue macrophages. These are discussed in depth elsewhere (18). Finally, we will briefly report on the recently renewed interest for innate immune memory. A graphical representation of the review topic is shown in the Fig. 1.
Tissue macrophages are heterogeneous and versatile cells found in virtually all tissues of adult mammals, where they can represent up to 10~15% of the total cell number in quiescent conditions. This number can increase further in response to inflammatory stimuli (18). The functional specialization of macrophages in unique tissue microenvironments explains their heterogeneity (1920). Thus, macrophages take different names according to their tissue location, such as osteoclasts (bone), alveolar macrophages (lung), microglial cells (brain), histiocytes (connective tissue), Kupffer cells (liver), Langerhans cells (LC) (skin), etc. If on the one hand tissue macrophages acquire specific morphological and functional phenotypes according to the microenvironment in which they reside, on the other hand some functions of macrophages are the same in all tissues, such as the surveillance that maintains tissue homeostasis (recognizing and removing anomalous and senescent cells), and the protective function (reaction to infections and tissue damage by initiating, developing and resolving an inflammatory response). As mentioned above, it recently became evident the existence of a myb-independent hematopoietic stem cell (HSC)-independent lineage of tissue macrophages. Fate-mapping experiments showed that tissue-resident macrophages in multiple organs, including the liver (Kupffer cells), epidermis (LC), and brain (microglia), originate from the yolk sac (YS) or fetal liver (FL) (1521). Overviews of all the experiments demonstrating myb-independent macrophage origin are critically reviewed in (22).
Two main phases of embryonic hematopoiesis have been described in the mouse: 1. primitive hematopoiesis that takes place in YS and gives rise to macrophages without going through a monocytic progenitor (myb-independent); 2. definitive hematopoiesis that takes place in the FL (myb-dependent), which is initially seeded by hematopoietic progenitors from the YS and subsequently by hematopoietic stem cells from endothelium of the aorta-gonads-mesonephros (23). The relative contribution of YS progenitors to the circulating definitive hematopoietic progenitor cell pool that seeds the FL (and that then replenishes macrophages of peripheral organs) remains controversial. YS- and FL-derived macrophages have been considered distinct so far, but a recent work identified an erythro-myeloid progenitor population that originates in the YS and later migrates to the liver (24). Thus, a common origin in the YS for both YS- and FL-derived macrophages is a reasonable hypothesis. During embryogenesis, the FL subsequently becomes the source of definitive hematopoiesis that generates all major hematopoietic lineages, including circulating monocytes. FL monocytes populate most peripheral tissues (e.g., lung, spleen, dermis, liver), except the brain, and give rise to tissue macrophages, which generally coexist with, but can progressively displace, YS-derived tissue macrophages. After birth, upon bone formation, hematopoiesis passes from the FL to the bone marrow. Blood monocyte precursors originate in the bone marrow, and blood monocytes constantly replenish resident macrophages in various organs, such as intestine and dermis (252627). Together with these monocyte-derived tissue resident macrophages, the tissues may encompass monocyte-derived macrophages that developed from inflammatory monocytes recruited during an infection or damage of the tissue (1828).
Macrophages that prenatally reside the tissues then proliferate to repopulate the expanding tissue with tissue resident macrophages. This proliferative potential or self-renewal capacity has been recently observed in microglia, in peritoneal, pleural and alveolar macrophages, in macrophages of adipose tissue and atherosclerotic plaques, and in cardiac macrophages (16). Nevertheless, tissue macrophages of embryonic origin can be replaced by monocytes-derived macrophages after severe inflammation (18), or in the aging heart (29).
Varol et al. (30) have recently reviewed the current knowledge on resident macrophage development and their functional specialization in the tissue, while Dey et al. (31) have examined tissue macrophage ontogenesis and relationship with blood monocytes. We refer the reader to these two reviews for deeper and detailed information on these aspects of macrophage biology.
The macrophage functional specialization/differentiation defines the presence of morphologically distinct macrophages with tissue-specific functions in homeostatic conditions. On the other hand, macrophage polarization describes the capacity of macrophages to modify their function in a plastic manner during the inflammatory reaction. Both tissue macrophages and monocyte-derived macrophages have a high level of plasticity, although their relative contribution to the progression and resolution of inflammation is not fully demonstrated. The relationship between tissue macrophages and monocyte-derived macrophages and their role in steady state conditions and during inflammation has been extensively reviewed in (18) and, with a focus on brain, adipose tissue and liver, in (31).
Macrophage polarization occurs through different activation programs, by which macrophages carry out their defense functions. In this way, macrophages become able to respond with appropriate functions in distinct contexts, and functional diversity becomes the key feature of these cells. Essentially, macrophages can modify their functions from a heal/growth promoting setting (M2 or alternative macrophages), to a killing/inhibitory capacity (M1 or classical macrophages) (32).
In vitro, macrophages are activated towards an M1 functional program by microorganism-related molecules (e.g., the gram-negative lipopolysaccharide, LPS) and by the inflammation-related cytokines TNF-α or IFN-γ, alone or in combination. M1 macrophages are efficient producers of toxic effector molecules (such as reactive oxygen/nitrogen species, and inflammatory cytokines), participate as inducers and effector cells in polarized Th1 responses, and mediate resistance against intracellular parasites and tumors (33).
Conversely, M2-like polarization has been observed in vitro in response to the Th2-related cytokines IL-4 or IL-13, to the concomitant triggering of Fcγ receptors and Toll-like receptors (TLR), to immune complexes, and to anti-inflammatory molecules such as IL-10, TGF-β, and glucocorticoids (3435). High levels of scavenger, mannose and galactose receptors characterize M2 cells in vitro. M2 macrophages take part in polarized Th2 responses, allergy, parasite clearance, dampening of inflammation, tissue remodeling, angiogenesis, immunoregulation, and tumor promotion (36). M2 is the normal "default" program adopted by resident macrophages (37).
In addition to functional differences, M1 and M2 macrophages have distinct features in terms of chemokine production profiles (38) and metabolism (39). The main distinction in terms of metabolism is that in M2 macrophages the arginine metabolism is shifted to ornithine and polyamines, which are important in the would healing process. Conversely, in M1 cells such metabolism is shifted to NO, which plays a key role in the intracellular killing of pathogens (40).
The M1/M2 classification is useful to understand the plasticity of macrophages but is just a limited attempt to capture the complexity and plasticity of these cells. In fact, this macrophage taxonomy does not fully mirror what really happens in the tissue during inflammation. In vivo the sequence of changing scenarios/signals presumably induces macrophages to adopt a variety of functional phenotypes during the course of an inflammatory reaction. Therefore, the M1/M2 polarization of macrophage functions may be taken as a simplified conceptual framework describing a continuum of diverse functional states, of which M1 and M2 activation states are not ontogenically defined subsets but represent the extremes of the functional continuum (41). As suggested by Martinez and Gordon (42), it is time for reassessing the concept of macrophage activation. Recently, Murray and coworkers attempted a re-classification of polarized macrophage in response to a range of stimuli (43). Furthermore, this issue has been recently addressed by network modeling analyses of hundreds of macrophage transcriptomes triggered by a diverse set of stimuli (44). This analysis revealed at least nine distinct macrophage activation programs, thus extending the dual M1/M2 macrophage polarization to a spectrum model. Moreover, knowing that local environment controls macrophage phenotypes, and given that many experiments with human cells are performed in vitro, we should redefine the in vitro culture conditions to closely match those occurring in a specific tissue microenvironment (4546) or in the course of an inflammatory reaction (47).
An important issue of macrophage polarization is whether polarized macrophage populations can switch one to the other in response to different conditions. Data from in vitro studies demonstrate that human monocytes can acquire the phenotype of polarized M1 macrophages and then mature into M2 repair macrophages upon exposure in culture to sequential changes in the microenvironmental conditions (47). A related open question is whether both tissue-resident macrophages and monocyte-derived macrophages can polarize in M1 or/and M2 functional phenotypes. These questions are abundantly discussed elsewhere (see for examples 18, 31). Here we just want to highlight the major role of microenvironmental signals in the functional phenotype switching of monocytes and macrophages by mentioning two severe pathological conditions: obesity and cancer. The metabolic syndrome can lead to a switch in the phenotype of adipose tissue macrophages from M2/wound healing (as in healthy non-obese humans) to classically activated macrophages (48). In cancer, tumor-infiltrating classically activated macrophages have the potential to contribute to the earliest stages of neoplasia, and then, as the tumor progresses, can progressively differentiate to a regulatory phenotype and eventually become cells that share the characteristics of both regulatory and wound-healing macrophages (49). In both cases, signals present in the surrounding environment at a given time drive the changes in the functional phenotype of adipose tissue macrophages and tumor-associated macrophages at given stage of the disease.
As previously discussed, innate immune cells have two peculiarities, diversity/heterogeneity and plasticity (50). Steady state hematopoietic differentiation programs may generate diverse subsets of monocytes (e.g., CD14++ CD16- or classical monocytes, CD14++CD16+ or intermediate monocytes, CD14+CD16++ or non-classical monocytes) (51), and distinct tissue-resident macrophages (e.g., Kupffer cells, microglia, LC, etc.). Thus, tissue-resident macrophages may be exposed to endogenous signals from the tissue during development or steady state conditions, in order to develop tissue specialization, whereas both tissue-resident macrophages and monocyte subpopulations (once in the tissue, monocyte-derived macrophages) may be subsequently activated by microbial or danger signals during inflammation or tissue damage. Thus, in the presence of diverse microenvironmental stimuli, each subset modifies its activation state in a plastic manner depending on the nature of the stimulus. As already mentioned, this feature has been termed plasticity or polarization. Phenotypic, transcriptional and epigenetics mechanisms underlie both diversity and plasticity, inducing functional specialization in different cells and in the same cells in different microenvironmental contexts.
We want to underline that heterogeneity, maintenance and specific function of resident macrophages are firmly under tissue control during homeostasis and in inflammatory conditions. This control mainly arises from different mechanisms: 1. tissue-selective transcriptional control; 2. environment reprogramming; 3. presence of silencing circuits.
It is evident that the expression of different transcription factor is required for specific functions in macrophages (5253). Several examples of transcription factors that dictate tissue-specific transcription programs in macrophages have been reported. For examples: the heme-induced transcription factor Spic is required for the development of red pulp macrophages (54), and Nr1h3 is needed for the development of all macrophages of the marginal zone (55). GATA6 is a regulator of a tissue-specific gene expression program in peritoneal macrophages, and retinoic acid released in the tissue is a signal that regulates reversibly the induction of GATA6 (46). Induction of PPAR-γ by GM-CSF is critical for the development of alveolar macrophages from fetal monocytes (56). Transcription factors such as STAT1, STAT6, C/EBPb, IRF-4, IRF5, and PPAR-γ have been shown to regulate transcription programs that control M1/M2 macrophage polarization (57).
Two recent studies that have employed RNA-seq have demonstrated the main role of the microenvironment in determining the macrophage phenotype reprogramming (5859). Authors concluded that tissue macrophages shared with other myeloid cells the epigenetic structure and gene expression (regulated by the master transcription factor PU.1), and in addition that each tissue has its own unique gene expression profile controlled by changes in enhancer landscapes. Moreover, tissue-specific reprogramming was evident by transferring macrophages from a tissue to another, showing that transferred cells lost most of the old tissue programming, acquiring a new one according to their new tissue microenvironment). The same was evident upon lethal irradiation of embryonic tissue macrophages and replacement with macrophages derived from transplanted healthy bone marrow.
Within a tissue, macrophages sense the microenvironmental changes through a series of sensors, such as PRR, scavenger receptors, cytokines receptors, and adhesion molecules, and are susceptible to silencing programs dictated by the tissue. Indeed, the tissue sets tissue-specific thresholds for the timing and extent of macrophage activation depending on the type and intensity of the stimulus. The exact nature of these silencing circuits differs between tissues and includes innate inherent suppression, as well as acquired deactivation induced activating stimuli (34). Examples are gut macrophages and microglia. Intestinal gut macrophages express the IL-10 receptor, and in order to prevent severe inflammation they must be exposed to homeostatic T regulatory cell-derived IL-10 (60). On the other hand, microglia is probably silenced by TGF-β (61) and maintained into a down-regulated phenotype by neural-derived CX3CR1 and CD200 (6263). Local tissue-derived signals are thought to control the development of the tissue-specific phenotypes of resident macrophages, proving the critical role of tissue environment. However, for most tissues the identity of these signals remains largely unknown (with some exceptions, see microglia, 61, and bone, 64).
In conclusion, the environment may reprogram macrophages in a tissue-specific manner in order to tailor their functions based on the tissue needs, or may polarize macrophages conferring a differently reactive phenotypes (classical vs. alternative vs. deactivated). Moreover, it is unknown whether functional heterogeneity and polarization are the result of irreversible lineage-specific differentiation or a consequence of continuous but reversible induction of diverse functional programs. Some functional differences may depend also on the diverse origin. It was recently suggested to classify monocyte/macrophage primarily by their ontogeny and only secondarily by their location, function and phenotype (65). However, which is the effect of origin on macrophage function is not yet fully known.
Considering the new insights in macrophage biology, it is worth mentioning the recent reassessment of the innate immune memory, an old concept that is currently raising a renewed interest in the scientific community. Indeed, revisited old knowledge on the repeated stimulation of the innate immune responses (see for instance 6667) has reintroduced the old concept of innate immune memory (68). Evidence in both plants and invertebrates (that do not possess adaptive immunity and classical memory) indicates that phagocytes can respond much better to a challenge if they have been pre-stimulated with the same or with another agent (69). Thus, innate immunity can have a memory, although different from acquired immune memory. A very interesting notion is that the innate memory is apparently at least in part non-specific, which implies that cells pre-challenged with (almost) any kind of agents can attain an improved defensive response to a subsequent challenge. Another very interesting concept is that of the exquisitely local dimension of phagocyte memory. In higher vertebrates such as man, tissues are disseminated with resident macrophages. These phagocytes have the main role of maintaining tissue integrity and function. However, in the case of external challenges (e.g., an infectious agent), they can initiate an immediate defensive inflammatory response. It is very interesting to observe that macrophages, after a challenge, can develop a memory and react to a second challenge in different ways: they become less reactive to some challenges to avoid extensive tissue damage or they display an enhanced response to improve tissue surveillance, e.g., against tumors. These two different responses of innate immune memory are known as "tolerance" and "trained immunity", respectively. LPS activates macrophages into a disruptive inflammatory reaction that also damages the surrounding tissue. Upon a second challenge, macrophages react much less because they aim at avoiding an excessive response that would destroy the tissue integrity. On the other hand, challenge with fungal components (implying a long-term slow infection with tissue debilitation) induces an innate memory that results in enhanced reactivity to subsequent stimuli, necessary for the adequate defense of a weakened tissue.
The molecular mechanisms responsible for shifting macrophages toward a memory status have not yet been elucidated. Putative mechanisms may involve different recruitment of different monocyte/macrophage subpopulation (i.e., CD14+ and CD16-) (68), or changes in the expression of lectin receptors on cell membrane (70), or in the functional phenotype (e.g., phagocytosis or protein production). In any case, all of these changes are probably due to epigenetic reprogramming that regulates gene expression by inducing dynamic alterations in the chromatin structure, through modification of DNA, post-translational modifications of histones (methylation), or microRNA (717273). Another interesting aspects of innate memory are changes in metabolic processes, as already observed in macrophage polarization (3974). Whether monocyte-derived macrophages or tissue macrophages or both retain a memory of past challenge has not been fully demonstrated, as well as their fate in the tissue. We preliminarily observed (unpublished observation) that a mild microbial stimulus could induce different immunological programming in monocyte-derived macrophages vs. tissue macrophages. Indeed, in vitro monocyte-derived macrophages and tissue macrophages primed with microbial agents (e.g., LPS, β-glucan) produce a substantially different profile of inflammatory cytokines upon re-exposure, demonstrating the capacity of adapting their response to an evolving situation (such as multiple or chronic infections). Notably, the type of innate memory induced by pre-challenge may be different (decrease vs. increase vs. no effect) depending on the inflammatory endpoint (e.g., production of TNF-α or IL-1β or IL-8) and the type of mononuclear phagocyte, with inflammatory monocytes being more reactive than tissue macrophages.
The increased understanding of the properties of innate memory is changing our awareness of host defense and immune memory, and could lead to defining new classes of vaccines and adjuvants. Efficacy of many vaccines probably implies the induction of non-specific macrophage memory that contributes to the increased resistance to infections. Research in the field of memory macrophages needs a thorough reassessment of a large body of old evidence accumulated in the past decades in the areas of macrophage activation and of adjuvanticity.
Given the important role of macrophages in acute and chronic inflammation and in other severe diseases (artherosclerosis, neurodegeneration and cancer), it is not surprising the growing interest for their proprieties and for their potential clinical applications. In a recent review, Martinez and Gordon address the issue of translating the new knowledge of macrophage biology into clinical practice (75). They describe a selection of the potential functional targets in macrophages, such as macrophage recruitment, activation, and memory, which are being considered for potential therapeutic/clinical application by using ad hoc inhibitors. Moreover they indicate new application areas that are likely to develop in the near future, thanks to our new understanding of macrophage biology, including cell therapy, diagnosis and prognosis.
We agree with Martinez and Gordon especially on the following key needs: more studies on humans to close the gap between the bench and the clinic; and use of standardized and improved methods to investigate macrophages heterogeneity and to measure genes, proteins and metabolites in situ.
The common view is that macrophage diversity and plasticity are driven by cues in the tissue microenvironment, which can include cytokines, growth factors and microorganism-associated molecular patterns. These signals are believed to dictate a transcriptional response that shapes the phenotype and function of macrophages based on the physiological or pathological context. Progress has been made in defining the molecular mechanism underlying macrophage biology, but the data are still incomplete and far from being systematic. Thus, we need to increase our knowledge of the mechanistic basis of macrophage heterogeneity/diversity and plasticity, and how to pharmacologically manipulate them. Considering that various in vitro models currently used do not adequately reflect the heterogeneity and plasticity of tissue macrophages, we conclude by suggesting that future studies should be conducted on macrophages isolated directly from tissues in different homeostatic and pathological conditions (in steady state condition and in response to a changing environment). Single-cell transcriptomic and epigenetic analysis (76) might help to molecularly identify the macrophage functional states and their changes driven by changing tissue conditions.
Figures and Tables
Figure 1
New insights in macrophages. Origin. During embryogenesis, tissue macrophages derive from yolk sac (YS) and fetal liver (FL) progenitors, by primitive and definitive hematopoiesis, respectively. It is possible that FL progenitors may derive from YS progenitors (orange arrow). After birth and in adulthood, monocyte-derived macrophages come from bone marrow (BM) progenitors by definitive hematopoiesis. Heterogeneity/Diversity. Macrophage heterogeneity depends on tissue microenvironment and origin. In the tissue, macrophages can differentiate and acquire a functional specialization depending on tissue-specific signals (▴•♦▪), thereby becoming microglia in the brain, Langerhans cells in the dermis, Kupffer cells in the liver, and gut macrophages (Mϕ) in the intestine. The differentiation in the tissue is both phenotypical and functional, but it is not end-stage, since tissue macrophages retain proliferative/self-renewal capacity and functional plasticity (see below). The yellow and blue bold arrows indicate different origin of these macrophages, being microglia totally yolk sac-derived, while gut macrophages are mostly monocyte-derived macrophages. Thin brown arrows indicate that a fraction of macrophages from FL is already present in tissues such as liver and dermis (taken as examples), but also in peritoneum, lung, spleen, pancreas, and kidney. Polarization/Plasticity. During the different phases of inflammation, the microenviromental changes can polarize macrophages in different functional phenotypes, from an inflammatory (M1) to an alternative/deactivated phenotype (M2), responsible of induction and resolution of inflammation, respectively. These two phenotypes are the extreme of a spectrum of multiple phenotypes (here signed as MX), as many as the microenvironmental scenarios could be. Memory. Macrophages can react to a second challenge reducing (tolerance) or enhancing (trained immunity) their own immune response in terms of cytokine production. Different mechanisms underlie this macrophage capacity, such as epigenetic and metabolic reprogramming or activation of different signaling pathways. In the picture, the memory phenotype referred to as "mixed memory phenotype" indicates that the type of innate memory induced by pre-challenge can be different (increased or decreased cytokine production) and coexist in the same cells depending on the inflammatory cytokines measured and the type of mononuclear phagocyte (see text).
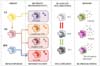
ACKNOWLEDGEMENTS
This work was supported by BioCog (FP7-HEALTH-2013-INNOVATION-1 GA n. 602461), HUMUNITY (FP7-PEOPLE-INT-2012 GA n. 316383), the grant 2011-2114 of Fondazione Cariplo (Milano, Italy), and a grant within the Executive Protocol Italy-South Korea 2013, financed by the Ministry of Foreign Affairs of Italy and the National Research Foundation (0652-20130012) of Korea.
References
1. Buchmann K. Evolution of Innate Immunity Clues from Invertebrates via Fish to Mammals. Front Immunol. 2014; 5:459.


2. Wong BW, Meredith A, Lin D, McManus BM. The biological role of inflammation in atherosclerosis. Can J Cardiol. 2012; 28:631–641.


3. Viola J, Soehnlein O. Atherosclerosis - A matter of unresolved inflammation. Semin Immunol. 2015; 27:184–193.


4. Mantovani A, Allavena P, Sica A, Balkwill F. Cancer-related inflammation. Nature. 2008; 454:436–444.


5. Simon A, van der Meer JW. Pathogenesis of familial periodic fever syndromes or hereditary autoinflammatory syndromes. Am J Physiol Regul Integr Comp Physiol. 2007; 292:R86–R98.


6. Masters SL, Simon A, Aksentijevich I, Kastner DL. Horror autoinflammaticus: the molecular pathophysiology of autoinflammatory disease (*). Annu Rev Immunol. 2009; 27:621–668.


7. Dinarello CA, van der Meer JW. Treating inflammation by blocking interleukin-1 in humans. Semin Immunol. 2013; 25:469–484.


8. Cavaillon JM. The historical milestones in the understanding of leukocyte biology initiated by Elie Metchnikoff. J Leukoc Biol. 2011; 90:413–424.


9. Aschoff J. Das reticuloendothelial system. Erg Inn Med Kinderheilk. 1924; 26:1–119.
10. van Furth R, Cohn ZA. The origin and kinetics of mononuclear phagocytes. J Exp Med. 1968; 128:415–435.


11. van Furth R, Diesselhoff-den Dulk MM. Dual origin of mouse spleen macrophages. J Exp Med. 1984; 160:1273–1283.


12. Parwaresch MR, Wacker HH. Origin and kinetics of resident tissue macrophages. Parabiosis studies with radiolabelled leucocytes. Cell Tissue Kinet. 1984; 17:25–39.
13. Czernielewski JM, Demarchez M. Further evidence for the self-reproducing capacity of Langerhans cells in human skin. J Invest Dermatol. 1987; 88:17–20.


14. Melnicoff MJ, Horan PK, Breslin EW, Morahan PS. Maintenance of peritoneal macrophages in the steady state. J Leukoc Biol. 1988; 44:367–375.


15. Schulz C, Gomez PE, Chorro L, Szabo-Rogers H, Cagnard N, Kierdorf K, Prinz M, Wu B, Jacobsen SE, Pollard JW, Frampton J, Liu KJ, Geissmann F. A lineage of myeloid cells independent of Myb and hematopoietic stem cells. Science. 2012; 336:86–90.


16. Gentek R, Molawi K, Sieweke MH. Tissue macrophage identity and self-renewal. Immunol Rev. 2014; 262:56–73.


17. Ley K, Miller YI, Hedrick CC. Monocyte and macrophage dynamics during atherogenesis. Arterioscler Thromb Vasc Biol. 2011; 31:1506–1516.


18. Italiani P, Boraschi D. From Monocytes to M1/M2 Macrophages: Phenotypical vs Functional Differentiation. Front Immunol. 2014; 5:514.


19. Epelman S, Lavine KJ, Randolph GJ. Origin and functions of tissue macrophages. Immunity. 2014; 41:21–35.


20. Haldar M, Murphy KM. Origin, development, and homeostasis of tissue-resident macrophages. Immunol Rev. 2014; 262:25–35.


21. Yona S, Kim KW, Wolf Y, Mildner A, Varol D, Breker M, Strauss-Ayali D, Viukov S, Guilliams M, Misharin A, Hume DA, Perlman H, Malissen B, Zelzer E, Jung S. Fate mapping reveals origins and dynamics of monocytes and tissue macrophages under homeostasis. Immunity. 2013; 38:79–91.


22. Gomez Perdiguero E, Geissmann F. Myb-independent macrophages: a family of cells that develops with their tissue of residence and is involved in its homeostasis. Cold Spring Harb Symp Quant Biol. 2013; 78:91–100.


24. Gomez Perdiguero E, Klapproth K, Schulz C, Busch K, Azzoni E, Crozet L, Garner H, Trouillet C, de Bruijn MF, Geissmann F, Rodewald HR. Tissue-resident macrophages originate from yolk-sac-derived erythro-myeloid progenitors. Nature. 2015; 518:547–551.


25. Tamoutounour S, Guilliams M, Montanana SF, Liu H, Terhorst D, Malosse C, Pollet E, Ardouin L, Luche H, Sanchez C, Dalod M, Malissen B, Henri S. Origins and functional specialization of macrophages and of conventional and monocyte-derived dendritic cells in mouse skin. Immunity. 2013; 39:925–938.


26. Zigmond E, Jung S. Intestinal macrophages: well educated exceptions from the rule. Trends Immunol. 2013; 34:162–168.


27. Bain CC, Bravo-Blas A, Scott CL, Gomez PE, Geissmann F, Henri S, Malissen B, Osborne LC, Artis D, Mowat AM. Constant replenishment from circulating monocytes maintains the macrophage pool in the intestine of adult mice. Nat Immunol. 2014; 15:929–937.


28. Davies LC, Rosas M, Jenkins SJ, Liao CT, Scurr MJ, Brombacher F, Fraser DJ, Allen JE, Jones SA, Taylor PR. Distinct bone marrow-derived and tissue-resident macrophage lineages proliferate at key stages during inflammation. Nat Commun. 2013; 4:1886.


29. Molawi K, Wolf Y, Kandalla PK, Favret J, Hagemeyer N, Frenzel K, Pinto AR, Klapproth K, Henri S, Malissen B, Rodewald HR, Rosenthal NA, Bajenoff M, Prinz M, Jung S, Sieweke MH. Progressive replacement of embryo-derived cardiac macrophages with age. J Exp Med. 2014; 211:2151–2158.


30. Varol C, Mildner A, Jung S. Macrophages: development and tissue specialization. Annu Rev Immunol. 2015; 33:643–675.


31. Dey A, Allen J, Hankey-Giblin PA. Ontogeny and polarization of macrophages in inflammation: blood monocytes versus tissue macrophages. Front Immunol. 2014; 5:683.


32. Mills CD. M1 and M2 Macrophages: Oracles of Health and Disease. Crit Rev Immunol. 2012; 32:463–488.


35. Martinez FO, Sica A, Mantovani A, Locati M. Macrophage activation and polarization. Front Biosci. 2008; 13:453–461.


36. Sica A, Mantovani A. Macrophage plasticity and polarization: in vivo veritas. J Clin Invest. 2012; 122:787–795.
37. Murray PJ, Wynn TA. Protective and pathogenic functions of macrophage subsets. Nat Rev Immunol. 2011; 11:723–737.


38. Mantovani A, Sica A, Sozzani S, Allavena P, Vecchi A, Locati M. The chemokine system in diverse forms of macrophage activation and polarization. Trends Immunol. 2004; 25:677–686.


39. Galvan-Pena S, O'Neill LA. Metabolic reprograming in macrophage polarization. Front Immunol. 2014; 5:420.
40. Rath M, Muller I, Kropf P, Closs EI, Munder M. Metabolism via arginase or nitric oxide synthase: Two competing arginine pathways in macrophages. Front Immunol. 2014; 5:532.


41. Mosser DM, Edwards JP. Exploring the full spectrum of macrophage activation. Nat Rev Immunol. 2008; 8:958–969.


42. Martinez FO, Gordon S. The M1 and M2 paradigm of macrophage activation: time for reassessment. F1000Prime Rep. 2014; 6:13.


43. Murray PJ, Allen JE, Biswas SK, Fisher EA, Gilroy DW, Goerdt S, Gordon S, Hamilton JA, Ivashkiv LB, Lawrence T, Locati M, Mantovani A, Martinez FO, Mege JL, Mosser DM, Natoli G, Saeij JP, Schultze JL, Shirey KA, Sica A, Suttles J, Udalova I, van Ginderachter JA, Vogel SN, Wynn TA. Macrophage activation and polarization: nomenclature and experimental guidelines. Immunity. 2014; 41:14–20.


44. Xue J, Schmidt SV, Sander J, Draffehn A, Krebs W, Quester I, De ND, Gohel TD, Emde M, Schmidleithner L, Ganesan H, Nino-Castro A, Mallmann MR, Labzin L, Theis H, Kraut M, Beyer M, Latz E, Freeman TC, Ulas T, Schultze JL. Transcriptome-based network analysis reveals a spectrum model of human macrophage activation. Immunity. 2014; 40:274–288.


45. Butovsky O, Jedrychowski MP, Moore CS, Cialic R, Lanser AJ, Gabriely G, Koeglsperger T, Dake B, Wu PM, Doykan CE, Fanek Z, Liu L, Chen Z, Rothstein JD, Ransohoff RM, Gygi SP, Antel JP, Weiner HL. Identification of a unique TGF-beta-dependent molecular and functional signature in microglia. Nat Neurosci. 2014; 17:131–143.


46. Okabe Y, Medzhitov R. Tissue-specific signals control reversible program of localization and functional polarization of macrophages. Cell. 2014; 157:832–844.


47. Italiani P, Mazza EM, Lucchesi D, Cifola I, Gemelli C, Grande A, Battaglia C, Bicciato S, Boraschi D. Transcriptomic profiling of the development of the inflammatory response in human monocytes in vitro. PLoS One. 2014; 9:e87680.
48. Kraakman MJ, Murphy AJ, Jandeleit-Dahm K, Kammoun HL. Macrophage polarization in obesity and type 2 diabetes: weighing down our understanding of macrophage function? Front Immunol. 2014; 5:470.


49. Biswas SK, Mantovani A. Macrophage plasticity and interaction with lymphocyte subsets: cancer as a paradigm. Nat Immunol. 2010; 11:889–896.


50. Soumelis V, Pattarini L, Michea P, Cappuccio A. Systems approaches to unravel innate immune cell diversity, environmental plasticity and functional specialization. Curr Opin Immunol. 2015; 32:42–47.


51. Ziegler-Heitbrock L, Hofer TP. Toward a refined definition of monocyte subsets. Front Immunol. 2013; 4:23.


52. Molawi K, Sieweke MH. Transcriptional control of macrophage identity, self-renewal, and function. Adv Immunol. 2013; 120:269–300.


53. Gautier EL, Yvan-Charvet L. Understanding macrophage diversity at the ontogenic and transcriptomic levels. Immunol Rev. 2014; 262:85–95.


54. Kohyama M, Ise W, Edelson BT, Wilker PR, Hildner K, Mejia C, Frazier WA, Murphy TL, Murphy KM. Role for Spi-C in the development of red pulp macrophages and splenic iron homeostasis. Nature. 2009; 457:318–321.


55. A-Gonzalez N, Guillen JA, Gallardo G, Diaz M, de la Rosa JV, Hernandez IH, Casanova-Acebes M, Lopez F, Tabraue C, Beceiro S, Hong C, Lara PC, Andujar M, Arai S, Miyazaki T, Li S, Corbi AL, Tontonoz P, Hidalgo A, Castrillo A. The nuclear receptor LXRalpha controls the functional specialization of splenic macrophages. Nat Immunol. 2013; 14:831–839.


56. Schneider C, Nobs SP, Kurrer M, Rehrauer H, Thiele C, Kopf M. Induction of the nuclear receptor PPAR-gamma by the cytokine GM-CSF is critical for the differentiation of fetal monocytes into alveolar macrophages. Nat Immunol. 2014; 15:1026–1037.


57. Lawrence T, Natoli G. Transcriptional regulation of macrophage polarization: enabling diversity with identity. Nat Rev Immunol. 2011; 11:750–761.


58. Gosselin D, Link VM, Romanoski CE, Fonseca GJ, Eichenfield DZ, Spann NJ, Stender JD, Chun HB, Garner H, Geissmann F, Glass CK. Environment drives selection and function of enhancers controlling tissue-specific macrophage identities. Cell. 2014; 159:1327–1340.


59. Lavin Y, Winter D, Blecher-Gonen R, David E, Keren-Shaul H, Merad M, Jung S, Amit I. Tissue-resident macrophage enhancer landscapes are shaped by the local microenvironment. Cell. 2014; 159:1312–1326.


60. Zigmond E, Bernshtein B, Friedlander G, Walker CR, Yona S, Kim KW, Brenner O, Krauthgamer R, Varol C, Muller W, Jung S. Macrophage-restricted interleukin-10 receptor deficiency, but not IL-10 deficiency, causes severe spontaneous colitis. Immunity. 2014; 40:720–733.


61. Butovsky O, Jedrychowski MP, Moore CS, Cialic R, Lanser AJ, Gabriely G, Koeglsperger T, Dake B, Wu PM, Doykan CE, Fanek Z, Liu L, Chen Z, Rothstein JD, Ransohoff RM, Gygi SP, Antel JP, Weiner HL. Identification of a unique TGF-beta-dependent molecular and functional signature in microglia. Nat Neurosci. 2014; 17:131–143.


62. Perry VH, Teeling J. Microglia and macrophages of the central nervous system: the contribution of microglia priming and systemic inflammation to chronic neurodegeneration. Semin Immunopathol. 2013; 35:601–612.


63. Wolf Y, Yona S, Kim KW, Jung S. Microglia, seen from the CX3CR1 angle. Front Cell Neurosci. 2013; 7:26.


64. Boyle WJ, Simonet WS, Lacey DL. Osteoclast differentiation and activation. Nature. 2003; 423:337–342.


65. Guilliams M, Ginhoux F, Jakubzick C, Naik SH, Onai N, Schraml BU, Segura E, Tussiwand R, Yona S. Dendritic cells, monocytes and macrophages: a unified nomenclature based on ontogeny. Nat Rev Immunol. 2014; 14:571–578.


66. Boraschi D, Meltzer MS. Defective tumoricidal capacity of macrophages from A/J mice II Comparison of the macrophage cytotoxic defect of A/J mice with that of lipid A-unresponsive C3H/HeJ mice. J Immunol. 1979; 122:1592–1597.
67. Tagliabue A, Boraschi D, McCoy JL. Development of cell-mediated antiviral immunity and macrophage activation in C3H/HeN mice infected with mouse mammary tumor virus. J Immunol. 1980; 124:2203–2208.
68. Netea MG, Quintin J, van der Meer JW. Trained immunity: a memory for innate host defense. Cell Host Microbe. 2011; 9:355–361.


70. Bowdish DM, Loffredo MS, Mukhopadhyay S, Mantovani A, Gordon S. Macrophage receptors implicated in the "adaptive" form of innate immunity. Microbes Infect. 2007; 9:1680–1687.


71. Quintin J, Saeed S, Martens JH, Giamarellos-Bourboulis EJ, Ifrim DC, Logie C, Jacobs L, Jansen T, Kullberg BJ, Wijmenga C, Joosten LA, Xavier RJ, van der, Stunnenberg HG, Netea MG. Candida albicans infection affords protection against reinfection via functional reprogramming of monocytes. Cell Host Microbe. 2012; 12:223–232.


72. Saeed S, Quintin J, Kerstens HH, Rao NA, Aghajanirefah A, Matarese F, Cheng SC, Ratter J, Berentsen K, van der Ent MA, Sharifi N, Janssen-Megens EM, Ter HM, Mandoli A, van ST, Ng A, Burden F, Downes K, Frontini M, Kumar V, Giamarellos-Bourboulis EJ, Ouwehand WH, van der Meer JW, Joosten LA, Wijmenga C, Martens JH, Xavier RJ, Logie C, Netea MG, Stunnenberg HG. Epigenetic programming of monocyte-to-macrophage differentiation and trained innate immunity. Science. 2014; 345:1251086.


73. Foster SL, Hargreaves DC, Medzhitov R. Gene-specific control of inflammation by TLR-induced chromatin modifications. Nature. 2007; 447:972–978.


74. Biswas SK, Mantovani A. Orchestration of metabolism by macrophages. Cell Metab. 2012; 15:432–437.

