Abstract
Lung fibrosis is a life-threatening disease caused by overt or insidious inflammatory responses. However, the mechanism of tissue injury-induced inflammation and subsequent fibrogenesis remains unclear. Recently, we and other groups reported that Th17 responses play a role in amplification of the inflammatory phase in a murine model induced by bleomycin (BLM). Osteopontin (OPN) is a cytokine and extracellular-matrix-associated signaling molecule. However, whether tissue injury causes inflammation and consequent fibrosis through OPN should be determined. In this study, we observed that BLM-induced lung inflammation and subsequent fibrosis was ameliorated in OPN-deficient mice. OPN was expressed ubiquitously in the lung parenchymal and bone-marrow-derived components and OPN from both components contributed to pathogenesis following BLM intratracheal instillation. Th17 differentiation of CD4+ αβ T cells and IL-17-producing γδ T cells was significantly reduced in OPN-deficient mice compared to WT mice. In addition, Th1 differentiation of CD4+ αβ T cells and the percentage of IFN-γ-producing γδ T cells increased. T helper cell differentiation in vitro revealed that OPN was preferentially upregulated in CD4+ T cells under Th17 differentiation conditions. OPN expressed in both parenchymal and bone marrow cell components and contributed to BLM-induced lung inflammation and fibrosis by affecting the ratio of pathogenic IL-17/protective IFN-γ T cells.
Fibrosis is the result of inappropriate wound-healing processes, characterized as an excessive deposition of extracellular matrix proteins in place of parenchymal cells (12). Fibrotic tissue remodeling causes the destruction of normal architecture, leading to organ malfunction. The persistent activation of inflammatory pathways following tissue injury is associated with the development of fibrotic diseases. The mechanism involved in triggering the transition from acute to chronic inflammation is under investigation (3). Recently, several groups have shown that Th17 differentiation in the lung connects the initial innate responses to the adaptive arms of immune responses in bleomycin-treated pulmonary fibrosis murine models (4567).
Osteopontin (OPN) is a glycosylated protein expressed in various tissues, and is implicated in many biological processes including bone remodeling, stress response, inflammation, and cancer progression (891011). The OPN interaction with extracellular adhesion molecules has been well-characterized. Integrins including αVβ3, αVβ5, α9β1, and CD44 variants (CD44v) are receptors for OPN (12131415), and interactions between these receptors and OPN mediate the survival, migration, and adhesion of many cell types (16). In addition to its role in adhesion, OPN functions as a cytokine or intracellular signaling molecule during the development of various innate and adaptive immune cells (171819).
Numerous studies have shown the important role of OPN in wound healing and fibrosis (20). OPN is strongly expressed during fibrosis of the heart, lung, liver, and kidney (2122). In animal experiments, blockage of OPN expression during wound healing decreases the formation of granulation tissue and scarring (23). OPN is known to regulate cell-extracellular matrix interactions and modulate TGF-β1 and matrix metalloproteinase expression (24). For pulmonary fibrosis, OPN was found to distinguish idiopathic pulmonary fibrosis (IPF) from normal lungs in humans based on oligonucleotide arrays (2526). OPN produced by alveolar macrophages functions as a fibrogenic cytokine that promotes migration, adhesion, and proliferation of fibroblasts during the development of BLM-induced lung fibrosis (27). OPN expression is associated with important fibrogenic signals in the lung and a significant decrease in levels of active TGF-β1 and MMP2 in OPN-deficient mice. The epithelium may be an important source of osteopontin during lung fibrosis (28).
Since OPN is implicated in type I IFN production and Th17 differentiation (2930), we evaluated its role in a BLM-induced pulmonary fibrosis model focusing on fibrogenic T-helper cell differentiation following BLM administration. We also evaluated the effect of OPN expression in parenchymal cells (including lung epithelial cells) on pathogenesis using bone marrow chimeras (7). BLM-induced pulmonary inflammation and subsequent fibrosis was ameliorated in OPN-/- mice, and OPN was expressed in both lung parenchymal and bone-marrow-derived components, which contributed to pathogenesis following BLM intratracheal instillation. Reduced Th17 differentiation of CD4+ αβ T cells and IL-17-producing γδ T cells increased Th1 differentiation of CD4+ αβ T cells and increased the percentage of IFN-γ-producing T cells; thus, the IFN-γ/IL-17 ratio was increased in OPN-/- mice compared with wild-type (WT) mice. Moreover, OPN was preferentially upregulated in CD4+ T cells under Th17 differentiation conditions in vitro. Thus, OPN is expressed in both parenchymal and bone marrow cell components, and contributed to BLM-induced lung inflammation and fibrosis by affecting the pathogenic IL-17/protective IFN-γ T cell ratios.
C57BL/6 (B6) and OPN-/- mice were obtained from the Jackson Laboratory (Bar Harbor, ME). Male, 8-12-week-old mice were used for experiments, and all mice, including wild-type B6, were bred and maintained at the animal facility of Seoul National University College of Medicine. All animal experiments were performed with the approval of the Institutional Animal Care and Use Committee at Seoul National University (authorization no. SNU05050203).
Pulmonary fibrosis was induced by intratracheal instillation with bleomycin (BLM, 1.5 mg/kg, Nippon Kayaku, Japan) in 50 µl phosphate-buffered saline (PBS).
Recipient mice were lethally irradiated 950 rad whole body irradiation in two split doses) and injected intravenously with T cell-depleted bone marrow cells (3×106/mouse). Reconstituted mice were used in the experiments 8 weeks after bone marrow cell transfer.
Lung tissues were fixed in 4% paraformaldehyde, processed, and embedded in paraffin. Sections were stained with H&E for histopathological analysis.
Broncheoalveolar lavage was performed with five 1.0-ml aliquots of PBS through a tracheal cannula. To evaluate cytokine production, BALF cells were harvested and restimulated with 50 ng/ml PMA and 1 µg/ml ionomycin (Sigma-Aldrich) for 4 h. For intracellular staining, brefeldin A (BD-Pharmingen, San Diego, CA) was added during the final 2 h of stimulation. Cells were fixed with 4% paraformaldehyde, permeabilized with 0.5% Triton X-100, and incubated with anti-CD4, anti-γδTCR, anti-IL-17, and anti-IFN-γ antibodies (eBioscience). Intracellular cytokine levels were assayed by flow cytometry.
BALF cells were incubated with mAbs to mouse CD4, CD8α, B220, CD11c, γδTCR, and βTCR that were conjugated to fluorescein, phycoerythrin, PerCP-Cy5.5, or allophycocyanin (eBioscience). Cells were analyzed using a FACSCalibur (BD Biosciences, San Jose, CA) and FlowJo software (Tree Star, Ashland, OR).
Lymphocytes were isolated from lymph nodes and labeled with anti-CD4 or anti-CD8 antibodies. CD4+ or CD8+ T cells were purified using a FACS Aria cell sorter (BD). Purified T cells were stimulated with plate bound anti-CD3 and anti-CD28 (1 µg/ml each, BD-Pharmingen). For Th1 cell differentiation, recombinant IL-12 (10 ng/ml) and anti-IL-4 (10 µg/ml) were added during the stimulation. For Th2 cell differentiation, recombinant IL-4 (10 ng/ml), anti-IL-12 (10 µg/ml), and anti-IFN-γ (10 µg/ml) were added during the stimulation. For Th17 cell differentiation, recombinant IL-6 (10 ng/ml), TGF-β1 (5 ng/ml), anti-IL-4 (10 µg/ml), and anti-IFN-γ (10 µg/ml) were added during the stimulation.
Total RNA was isolated from lung tissues using Trizol (Invitrogen). cDNA was generated from 1 µg of total RNA by Superscript II (Invitrogen). The following primer pairs were used for semi-quantitative PCR: OPN, 5'-TCTGATGAG ACCGTCACTGC-3'/5'-TCTCCTGGCTCT CTTTGGAA-3'; GAPDH, 5'-CCCACTA ACATCAAATG GGG-3'/5'-ATCCACAGTCTTCTGGGTGG-3'. PCR products were analyzed by resolution on a 2% agarose gel followed by densitometric analysis (Labworks 4.6, UVP Bio-Imaging Systems, Cambridge, UK).
To evaluate the effect of OPN on the pathogenesis of pulmonary fibrosis, a bleomycin (BLM)-induced lung fibrosis model was induced in WT B6 and B6.OPN-/- (OPN-/-) mice. At day 21 of intratracheal BLM instillation, OPN-/- mice showed significantly reduced lung pathology in terms of cellular infiltration and collagen deposition compared to WT mice (Fig. 1A). Since OPN is expressed ubiquitously and its expression levels increased following BLM administration (28), we evaluated OPN expression in various tissues of normal adult untreated mice using semi-quantitative RT-PCR. High OPN expression was observed in parenchymal organs such as the brain, lung, and liver, but expression was lower in primary and secondary lymphoid organs such as the thymus, spleen, and lymph nodes (Fig. 1B). To evaluate the effect of OPN expressed in parenchymal or bone marrow-derived cells on BLM-induced lung fibrosis, we generated bone marrow chimeras with WT and OPN-/- bone marrow cells transferred into WT and OPN-/- recipients, and used the chimeras for the experiments after 8 weeks. At day 21 of BLM instillation, severe fibrogenic disease was induced in WT chimeras (WT→WT) and little evidence of lung pathology was observed in knockout chimeras (OPN-/-→OPN-/-). The lung pathology of both mixed chimeras, WT→OPN-/- and OPN-/-→WT, was intermediate between WT chimeras and knockout chimeras (Fig. 1C). These results indicated that OPN was expressed in both the parenchymal components (including lung epithelial cells and fibroblasts) and in the bone marrow-derived components (including T cells and dendritic cells), and contributed to the pathogenesis of BLM-induced lung fibrosis.
Immunological characteristics of OPN-/- mice were evaluated using flow cytometric analysis. Compared to WT mice, OPN-/- mice showed a decrease in αβ TCR+ T cells in secondary lymphoid organs such as peripheral lymph nodes, spleen, and liver (Fig. 2A). The percentage of γδ TCR+ T cells also decreased in OPN-/- mice compared to WT mice, but this reduction was not statistically significant (Fig. 2A). Among αβ TCR+ T cells, the percentage of CD4+ T cells was selectively reduced while that of CD8+ T cells was similar to WT mice (Fig. 2B). Dendritic cells, both conventional and B220+ cells, in the lymph nodes, spleen, and liver were similar between WT and OPN-/- mice (Fig. 2C).
To evaluate the immunological parameters during the pathogenesis of BLM-induced lung fibrosis, we analyzed bronchoalveolar lavage (BAL) fluid at day 10 of BLM instillation, which corresponded to the peak inflammatory response. The number of infiltrated γδ TCR+ T cells in BAL fluid was significantly reduced in OPN-/- mice compared to WT mice, and that of CD4+ αβ TCR+ T cells was similar between WT and OPN-/- mice (Fig. 3A, C, D). Intracellular cytokine analysis revealed a significant reduction in IL-17-producing T cells (both αβ TCR+ CD4+ and γδ TCR+ T cells) (Fig. 3B). However, the percentage of IFN-γ-producing T cells (both αβ CD4+ T cells and γδ T cells) increased (Fig. 3B). Because CD4+ αβ T cell numbers were not significantly decreased in OPN-/- mice, there was a net increase in Th1 differentiation and a decrease in Th17 differentiation in OPN-/- mice. Thus, the increased protective IFN-γ/pathogenic IL-17 ratio of pulmonary T cells in OPN-/- mice following BLM intratracheal instillation explained the reduced disease pathogenesis.
In this study, we found that OPN-/- mice showed strongly reduced Th17 differentiation of CD4+ T cells and IL-17-producing γδ T cells. In addition, the percentage of IFN-γ-secreting γδ T cells and Th1 differentiation increased in OPN-/- mice. Since Th17 differentiation of αβ CD4+ T cells plays an important role in the pathogenesis of BLM-induced pulmonary fibrosis, especially during the inflammatory amplification phase (7), we explored whether OPN was specific to the Th17 response. We first measured the expression levels of OPN in purified cell populations and found that OPN expression levels were similar in unactivated naïve CD4+ T cells, CD8+ T cells, CD11c+ dendritic cells, and B cells (Fig. 4A). When we activated CD4+ T and CD8+ T cells with anti-CD3 and anti-CD28 antibodies without additional cytokines, OPN expression levels were similar in these two populations and did not increase following in vitro activation during the initial 48 h (Fig. 4B). When we induced differentiation of CD4+ T cells under defined cytokine conditions, OPN expression specifically increased under Th17 differentiation conditions, but not under Th0, Th1, and Th2 differentiation conditions, during the initial 48 h (Fig. 4C).
In this study, we observed that BLM-induced lung inflammation and subsequent fibrosis were ameliorated in OPN-deficient mice; moreover, OPN was expressed in both the lung parenchymal and bone-marrow-derived components and contributed to pathogenesis following intratracheal BLM instillation. Th17 differentiation of CD4+ αβ T cells and IL-17-producing γδ T cells was significantly reduced in OPN-deficient mice compared to WT mice. In addition, Th1 differentiation of CD4+ αβ T cells and the percentage of IFN-γ-producing γδ T cells increased. T helper cell differentiation in vitro revealed that OPN was preferentially upregulated in CD4+ T cells under Th17 differentiation conditions.
The critical role of IL-17A in the pathogenesis of BLM-induced lung fibrosis has been reported previously (4567). The pathogenic role of IL-17A was more pronounced during the initial inflammatory amplification phase. Blocking IL-17A through IL-17RA-Fc revealed that the greatest reduction in fibrosis occurred when we treated with IL-17RA-Fc during the initial inflammatory phase, and minimal effects were observed when we treated with IL-17RA-Fc during the later fibrosis phase (7). Protective anti-fibrotic activity of IFN-γ in various organs, including BLM-induced lung fibrosis, has been reported previously (313233). Thus, the IL-17/IFN-γ ratio in the pulmonary microenvironment affects the pathogenesis of lung fibrosis.
An immunomodulatory effect of OPN on T helper cell differentiation has been reported. OPN induces Th1 responses by upregulating IL-12 and downregulating IL-10 through αVβ3 integrin and CD44, respectively, expressed on antigen-presenting cells (34). OPN induces IL-17 production through CD4+ T cells via the β3 integrin receptor expressed on T cells, which aggravates the pathogenesis of autoimmune encephalomyelitis (35). In addition, intracellular OPN expressed by conventional dendritic cells enhanced Th17 responses by suppressing IL-27 expression (36). Thus, OPN induces both Th1 and Th17 differentiation. In this respect, preferential Th17 differentiation by OPN in the BLM-induced lung fibrosis model in this study enhanced Th1 differentiation in OPN-deficient mice, which was unexpected. This may reflect that following BLM intratracheal instillation, the local cytokine microenvironment in the lung rapidly changes to favor Th17 differentiation (67), and OPN plays a key role in pathogenic Th17 differentiation. The preferential and critical role of OPN in Th17 differentiation is explained by the rapid kinetics of OPN expression in CD4+ T cells under in vitro Th17 differentiation conditions compared to Th0, Th1, or Th2 differentiation conditions. Meanwhile, in vitro differentiation of Th1 or Th17 cells from OPN-deficient CD4+ T cells was comparable to that of WT CD4+ T cells (data not shown), which indicates that T-cell-intrinsic OPN is not always required for Th1 or Th17 differentiation and that the cytokine microenvironment provided by non-T-cell OPN is important for T-helper cell differentiation. In addition, the Th17 differentiating cytokine microenvironment following BLM intratracheal instillation, which contains TGF-β, inhibits Th1 differentiation (37). Thus, increased Th1 differentiation in the absence of OPN suggests that OPN is indispensable for providing a Th17 differentiating microenvironment but is dispensable for Th1 differentiation in a BLM-induced lung fibrosis model.
In this study we detected decreased numbers of γδ T cells and an increased IFN-γ/IL-17 ratio in γδ T cells. Pulmonary γδ T cells have been implicated in protecting against several pulmonary pathologies, including BLM-induced lung fibrosis, silicosis, hypersensitivity pneumonitis (43839), and IL-22 produced by γδ T cells (40). Since we did not evaluate IL-22 expression in WT and OPN-deficient mice, we cannot accurately determine the effect of γδ T cell changes in OPN-deficient mice. Even with decreased γδ T cell numbers, the increased IFN-γ/IL-17 ratio of these cells may provide protective effects in OPN-deficient mice.
Because it acts as an intracellular signaling molecule, a cytokine, a chemokine, and an adhesion molecule, and is expressed ubiquitously, OPN affects or orchestrates diverse aspects of both the innate and adaptive immune responses, which explains the complete protection against BLM-induced fibrosis in OPN-deficient mice. In this study, OPN expression in both lung parenchymal cells and bone-marrow-derived cells contributed to the lung pathology. In this respect, localized expression of OPN in alveolar epithelial cells of IPF patients and the significantly increased level of OPN in bronchoalveolar lavage fluid implies that critical changes in IPF epithelial cells induce OPN as a mediator of pathogenesis (2526). Although the BLM-induced fibrosis model is an inflammation-induced lung fibrosis model, epithelial cells play critical roles in initiating inflammatory responses (7). Epithelial-to-mesenchymal transition (EMT) following pulmonary epithelial cell activation and recruitment and stimulation of local and systemic fibroblasts, which are more relevant to IPF patient pathogenesis (12), may be involved in the pathogenesis, especially during the later fibrosis phase. Mixed bone marrow chimeras (both WT→OPN-/- and OPN-/-→WT) revealed substantial levels of pulmonary pathology, although the maximal disease score was observed in WT mice, indicating that OPN in the lung parenchymal cells or bone-marrow-derived cells was sufficient to induce lung inflammation and fibrosis. Unlike the BLM-induced lung fibrosis model, IPF patients showed less evidence of inflammation; thus, OPN in the IPF lung is likely involved in pathogenic fibrogenesis such as EMT and increased myofibroblast differentiation and proliferation.
Figures and Tables
Figure 1
OPN deficiency reduces BLM-induced pulmonary inflammation and fibrosis. (A) Representative photographs of lungs from WT and OPN-/- mice 21 days after intratracheal instillation of BLM (1.5 mg/kg). Sections were stained with H&E. (B) OPN expression levels in diverse tissues from WT B6 mice were analyzed using RT-PCR. (C) BM chimeras were prepared by irradiation of WT or OPN-/- mice, followed by T-cell-depleted BM cell reconstitution (BMWT→WT, BMWT→ OPN-/-, BMOPN-/-→WT, and BMOPN-/-→OPN-/-). Two months after BM cell reconstitution, pulmonary fibrosis was induced with BLM. Representative photographs of lungs 21 days after intratracheal instillation with BLM (1.5 mg/kg).
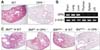
Figure 2
Lymphocytes of spleens, lymph nodes, and liver from WT and OPN-/- mice were harvested and stained with antibodies for αβTCR, γδTCR, CD4, CD8, CD11c, and B220 molecules. Cell populations were detected using flow cytometric analysis.
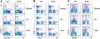
Figure 3
WT and OPN-/- mice that received an intratracheal instillation of BLM (1.5 mg/kg) were sacrificed to harvest BALF on 10 days. Cells from BALF were stimulated with PMA, and ionomycin, surface markers and intracellular cytokines were detected using flow cytometric analysis. (A) The percentages of CD4+ or γδTCR+ cells in BALF were detected using flow cytometric analysis. (B) The percentage of CD4+ or γδTCR+ cells producing IL-17 or IFN-γ in BALF of BLM-exposed WT and OPN-/- mice. (C) The number of CD4+ T cells in BALF. (D) The number of γδ T cells in BALF.
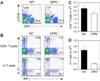
Figure 4
(A) Naïve CD4+ T cells, CD8+ T cells, CD11c+ dendritic cells, and B220+ B cells from lymph nodes and spleens were purified. OPN mRNA expression was determined using RT-PCR. (B) Purified CD4+ or CD8+ T cells were stimulated with anti-CD3 and anti-CD28 antibodies for 24 and 48 h. (C) Purified CD4+ T cells were stimulated with anti-CD3 and anti-CD28 antibodies under the Th0 (no additive), Th1 (10 ng/ml IL-12, 10 µg/ml anti-IL-4), Th2 (10 ng/ml IL-4, 10 µg/ml anti-IL-12, 10 µg/ml anti-IFN-γ), and Th17 (10 ng/ml IL-6, 5 ng/ml TGF-β, 10 µg/ml anti-IL-4, 10 µg/ml anti-IFN-γ) cell generation conditions. Representative data from three independent determinations are shown.
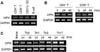
ACKNOWLEDGEMENTS
This work was supported by grants from the National R&D Program for Cancer Control, Ministry of Health & Welfare (1220200) and the National Research Foundation of Korea (NRF) grant funded by the Korea government (MEST) (2012014152).
References
1. Wynn TA, Ramalingam TR. Mechanisms of fibrosis: therapeutic translation for fibrotic disease. Nat Med. 2012; 18:1028–1040.


3. Wick G, Grundtman C, Mayerl C, Wimpissinger TF, Feichtinger J, Zelger B, Sgonc R, Wolfram D. The immunology of fibrosis. Annu Rev Immunol. 2013; 31:107–135.


4. Lo Re S, Dumoutier L, Couillin I, Van Vyve C, Yakoub Y, Uwambayinema F, Marien B, van den Brûle S, Van Snick J, Uyttenhove C, Ryffel B, Renauld JC, Lison D, Huaux F. IL-17A-producing gammadelta T and Th17 lymphocytes mediate lung inflammation but not fibrosis in experimental silicosis. J Immunol. 2010; 184:6367–6377.


5. Sonnenberg GF, Nair MG, Kirn TJ, Zaph C, Fouser LA, Artis D. Pathological versus protective functions of IL-22 in airway inflammation are regulated by IL-17A. J Exp Med. 2010; 207:1293–1305.


6. Wilson MS, Madala SK, Ramalingam TR, Gochuico BR, Rosas IO, Cheever AW, Wynn TA. Bleomycin and IL-1beta-mediated pulmonary fibrosis is IL-17A dependent. J Exp Med. 2010; 207:535–552.
7. Oh K, Park HB, Byoun OJ, Shin DM, Jeong EM, Kim YW, Kim YS, Melino G, Kim IG, Lee DS. Epithelial transglutaminase 2 is needed for T cell interleukin-17 production and subsequent pulmonary inflammation and fibrosis in bleomycin-treated mice. J Exp Med. 2011; 208:1707–1719.


8. Ishijima M, Rittling SR, Yamashita T, Tsuji K, Kurosawa H, Nifuji A, Denhardt DT, Noda M. Enhancement of osteoclastic bone resorption and suppression of osteoblastic bone formation in response to reduced mechanical stress do not occur in the absence of osteopontin. J Exp Med. 2001; 193:399–404.


9. Wang KX, Denhardt DT. Osteopontin: role in immune regulation and stress responses. Cytokine Growth Factor Rev. 2008; 19:333–345.


10. Chakraborty G, Jain S, Kundu GC. Osteopontin promotes vascular endothelial growth factor-dependent breast tumor growth and angiogenesis via autocrine and paracrine mechanisms. Cancer Res. 2008; 68:152–161.


11. Ahmed M, Behera R, Chakraborty G, Jain S, Kumar V, Sharma P, Bulbule A, Kale S, Kumar S, Mishra R, Raja R, Saraswati S, Kaur R, Soundararajan G, Kumar D, Thorat D, Sanyal M, Ramdasi A, Ghosh P, Kundu GC. Osteopontin: a potentially important therapeutic target in cancer. Expert Opin Ther Targets. 2011; 15:1113–1126.


12. Yamamoto N, Sakai F, Kon S, Morimoto J, Kimura C, Yamazaki H, Okazaki I, Seki N, Fujii T, Uede T. Essential role of the cryptic epitope SLAYGLR within osteopontin in a murine model of rheumatoid arthritis. J Clin Invest. 2003; 112:181–188.


13. Weber GF, Ashkar S, Glimcher MJ, Cantor H. Receptor-ligand interaction between CD44 and osteopontin (Eta-1). Science. 1996; 271:509–512.


14. Katagiri YU, Sleeman J, Fujii H, Herrlich P, Hotta H, Tanaka K, Chikuma S, Yagita H, Okumura K, Murakami M, Saiki I, Chambers AF, Uede T. CD44 variants but not CD44s cooperate with beta1-containing integrins to permit cells to bind to osteopontin independently of arginine-glycine-aspartic acid, thereby stimulating cell motility and chemotaxis. Cancer Res. 1999; 59:219–226.
15. Kanayama M, Kurotaki D, Morimoto J, Asano T, Matsui Y, Nakayama Y, Saito Y, Ito K, Kimura C, Iwasaki N, Suzuki K, Harada T, Li HM, Uehara J, Miyazaki T, Minami A, Kon S, Uede T. Alpha9 integrin and its ligands constitute critical joint microenvironments for development of autoimmune arthritis. J Immunol. 2009; 182:8015–8025.


16. Morimoto J, Kon S, Matsui Y, Uede T. Osteopontin; as a target molecule for the treatment of inflammatory diseases. Curr Drug Targets. 2010; 11:494–505.


17. Diao H, Kon S, Iwabuchi K, Kimura C, Morimoto J, Ito D, Segawa T, Maeda M, Hamuro J, Nakayama T, Taniguchi M, Yagita H, Van Kaer LK, Onóe K, Denhardt D, Rittling S, Uede T. Osteopontin as a mediator of NKT cell function in T cell-mediated liver diseases. Immunity. 2004; 21:539–550.


18. Chung JW, Kim MS, Piao ZH, Jeong M, Yoon SR, Shin N, Kim SY, Hwang ES, Yang Y, Lee YH, Kim YS, Choi I. Osteopontin promotes the development of natural killer cells from hematopoietic stem cells. Stem Cells. 2008; 26:2114–2123.


19. Diao H, Iwabuchi K, Li L, Onoe K, Van Kaer L, Kon S, Saito Y, Morimoto J, Denhardt DT, Rittling S, Uede T. Osteopontin regulates development and function of invariant natural killer T cells. Proc Natl Acad Sci U S A. 2008; 105:15884–15889.


20. Weber CE, Li NY, Wai PY, Kuo PC. Epithelial-mesenchymal transition, TGF-β, and osteopontin in wound healing and tissue remodeling after injury. J Burn Care Res. 2012; 33:311–318.


21. Brown LF, Berse B, Van de Water L, Papadopoulos-Sergiou A, Perruzzi CA, Manseau EJ, Dvorak HF, Senger DR. Expression and distribution of osteopontin in human tissues: widespread association with luminal epithelial surfaces. Mol Biol Cell. 1992; 3:1169–1180.


22. Ashizawa N, Graf K, Do YS, Nunohiro T, Giachelli CM, Meehan WP, Tuan TL, Hsueh WA. Osteopontin is produced by rat cardiac fibroblasts and mediates A(II)-induced DNA synthesis and collagen gel contraction. J Clin Invest. 1996; 98:2218–2227.


23. Liaw L, Birk DE, Ballas CB, Whitsitt JS, Davidson JM, Hogan BL. Altered wound healing in mice lacking a functional osteopontin gene (spp1). J Clin Invest. 1998; 101:1468–1478.


24. Konno S, Kurokawa M, Uede T, Nishimura M, Huang SK. Role of osteopontin, a multifunctional protein, in allergy and asthma. Clin Exp Allergy. 2011; 41:1360–1366.


25. Pardo A, Gibson K, Cisneros J, Richards TJ, Yang Y, Becerril C, Yousem S, Herrera I, Ruiz V, Selman M, Kaminski N. Up-regulation and profibrotic role of osteopontin in human idiopathic pulmonary fibrosis. PLoS Med. 2005; 2:e251.


26. Kelly MM, Leigh R, Gilpin SE, Cheng E, Martin GE, Radford K, Cox G, Gauldie J. Cell-specific gene expression in patients with usual interstitial pneumonia. Am J Respir Crit Care Med. 2006; 174:557–565.


27. Takahashi F, Takahashi K, Okazaki T, Maeda K, Ienaga H, Maeda M, Kon S, Uede T, Fukuchi Y. Role of osteopontin in the pathogenesis of bleomycin-induced pulmonary fibrosis. Am J Respir Cell Mol Biol. 2001; 24:264–271.


28. Berman JS, Serlin D, Li X, Whitley G, Hayes J, Rishikof DC, Ricupero DA, Liaw L, Goetschkes M, O'Regan AW. Altered bleomycin-induced lung fibrosis in osteopontin-deficient mice. Am J Physiol Lung Cell Mol Physiol. 2004; 286:L1311–L1318.


29. Shinohara ML, Lu L, Bu J, Werneck MB, Kobayashi KS, Glimcher LH, Cantor H. Osteopontin expression is essential for interferon-alpha production by plasmacytoid dendritic cells. Nat Immunol. 2006; 7:498–506.


30. Cantor H, Shinohara ML. Regulation of T-helper-cell lineage development by osteopontin: the inside story. Nat Rev Immunol. 2009; 9:137–141.


31. Baroni GS, D'Ambrosio L, Curto P, Casini A, Mancini R, Jezequel AM, Benedetti A. Interferon gamma decreases hepatic stellate cell activation and extracellular matrix deposition in rat liver fibrosis. Hepatology. 1996; 23:1189–1199.


32. Giri SN, Hyde DM, Marafino BJ Jr. Ameliorating effect of murine interferon gamma on bleomycin-induced lung collagen fibrosis in mice. Biochem Med Metab Biol. 1986; 36:194–197.


33. Oldroyd SD, Thomas GL, Gabbiani G, EI Nahas AM. Interferon-gamma inhibits experimental renal fibrosis. Kidney Int. 1996; 56:2116–2127.
34. Ashkar S, Weber GF, Panoutsakopoulou V, Sanchirico ME, Jansson M, Zawaideh S, Rittling SR, Denhardt DT, Glimcher MJ, Cantor H. Eta-1 (osteopontin): an early component of type-1 (cell-mediated) immunity. Science. 2000; 287:860–864.


35. Murugaiyan G, Mittal A, Weiner HL. Identification of an IL-27/osteopontin axis in dendritic cells and its modulation by IFN-gamma limits IL-17-mediated autoimmune inflammation. Proc Natl Acad Sci U S A. 2010; 107:11495–11500.


36. Shinohara ML, Kim JH, Garcia VA, Cantor H. Engagement of the type I interferon receptor on dendritic cells inhibits T helper 17 cell development: role of intracellular osteopontin. Immunity. 2008; 29:68–78.


38. Braun RK, Ferrick C, Neubauer P, Sjoding M, Sterner-Kock A, Kock M, Putney L, Ferrick DA, Hyde DM, Love RB. IL-17 producing gammadelta T cells are required for a controlled inflammatory response after bleomycin-induced lung injury. Inflammation. 2008; 31:167–179.


39. Simonian PL, Roark CL, Diaz del Valle F, Palmer BE, Douglas IS, Ikuta K, Born WK, O'Brien RL, Fontenot AP. Regulatory role of gammadelta T cells in the recruitment of CD4+ and CD8+ T cells to lung and subsequent pulmonary fibrosis. J Immunol. 2006; 177:4436–4443.


40. Simonian PL, Wehrmann F, Roark CL, Born WK, O'Brien RL, Fontenot AP. γδT cells protect against lung fibrosis via IL-22. J Exp Med. 2010; 207:2239–2253.