Abstract
Graft-versus-host disease (GVHD) is a fatal complication that occurs after allogeneic hematopoietic stem cell transplantation. To understand the dynamics of CD4 and CD8 T cell production of IFN-γ and IL-17 during GVHD progression, we established a GVHD model by transplanting T cell-depleted bone marrow (TCD-BM) and purified T cells from B6 mice into irradiated BALB.B, creating an MHC-matched but minor histocompatibility (H) antigen-mismatched transplantation (B6 → BALB.B GVHD). Transplantation-induced GVHD was confirmed by the presence of the appropriate compositional changes in the T cell compartments and innate immune cells in the blood and the systemic secretion of inflammatory cytokines. Using this B6 → BALB.B GVHD model, we showed that the production of IFN-γ and IL-17 by CD4 T cells preceded that by CD8 T cells in the spleen, mesenteric lymph node, liver, and lung in the BALB.B GVHD host, and Th1 differentiation predated Th17 differentiation in all organs during GVHD progression. Such changes in cytokine production were based on changes in cytokine gene expression by the T cells at different time points during GVHD development. These results demonstrate that both IFN-γ and IL-17 are produced by CD4 and CD8 T cells but with different kinetics during GVHD progression.
Graft-versus-host disease (GVHD) is a fatal complication that occurs after allogeneic hematopoietic stem cell transplantation (HSCT) (1,2,3). GVHD is initiated by the activation of donor CD4 and CD8 T cells inoculated into the recipient together with hematopoietic stem cells. The activated CD4 and CD8 T cells proliferate and subsequently infiltrate the GVHD target organs such as the skin, lung, liver, and intestines, which causes tissue damage by inducing organ-specific GVHD pathology in the host (4,5). The degree of histocompatibility disparity between the donor and host, the conditioning regimens, and the presence of inflammation affect the extent of alloreactive T cell responses and subsequent GVHD manifestations (6).
Activated CD4 T cells differentiate into different types of helper cells (Th1, Th2, or Th17) with distinct functional characteristics. Among these types, Th1 and Th17 cells contribute considerably to GVHD pathology (7). Donor CD4 T cells differentiate predominantly into Th1 cells and mediate GVHD tissue damage in the gut and liver in an MHC-mismatched GVHD model (8). However, rather than alleviating GVHD, IFN-γ-deficiency in the donor T cells exacerbate acute GVHD, augmenting Th2 and Th17 differentiation and damage in the lung and skin (8,9). IFN-γ and IL-4 deficiencies in donor T cells augment Th17 differentiation and tissue damage in the skin (8). Th17 cells that secrete cytokines such as IL-17A, IL-17F, IL-21, IL-22, and IL-26 (10) are implicated in the rejection of allogeneic tissues (11,12) and in acute GVHD (13). Transplantation of Th17 cells differentiated in vitro mediates skin and pulmonary GVHD (14), and hosts of IL-17-deficient CD4 T cells show delayed onset of GVHD with decreased numbers of IFN-γ-secreting cells (15). Transplantation of IL-17-deficient T cells augments Th1 differentiation and exacerbates acute GVHD (16), indicating a reciprocal influence between Th1 and Th17 cells on GVHD induction or severity. IFN-γ is a major effector molecule produced by activated CD8 T cells. IL-17-secreting CD8 T cells (Tc17) may represent a subset that is distinct from IFN-γ-secreting Tc1 cells, and they might have a therapeutic role in treating infectious disease and cancer (17). A recent report suggested that Tc17 cells are involved in GVHD induction (18). Although these previous studies suggest the involvement of IFN-γ- or IL-17-producing CD4 and CD8 T cells in mediating acute GVHD, cytokine production during GVHD pathogenesis remains unclear.
To understand the dynamics of CD4 and CD8 T cell production of IFN-γ and IL-17 during GVHD development, we performed a longitudinal analysis of the T cells using the B6 → BALB.B system. Transplantation of unfractionated cells from the bone marrow (BM) and spleen from B6 mice into lethally irradiated BALB.B mice (B6 → BALB.B) has been shown to induce acute GVHD with severe weight loss and more than 70% mortality (19). Within 7 to 10 days after transplantation, CD8 T cells specific for several dominant minor H antigens are detected in the blood, spleen, liver, and lung of the host with significant frequencies, suggesting the infiltration of minor H antigen-specific CD8 T cells into the target organs (19,20). Previously, we reported the production of IL-17 by CD11b+ and Gr-1+ innate immune cells after GVHD induction using the B6 → BALB.B model (21). To focus specifically on the cytokine production by mature donor CD4 and CD8 T cells after transplantation and rule out the possible influences of mature donor innate cells in the spleen on T cell differentiation during GVHD development, we established a B6 → BALB.B GVHD model by transplanting T cell-depleted BM (TCD-BM) cells and T cells purified from B6 mouse splenocytes into irradiated BALB.B mice, and assessed the dynamics of CD4 and CD8 T cell expansion and production of IFN-γ and IL-17. The results revealed different kinetics in cytokine production by donor CD4 and CD8 T cells in the allogeneic GVHD hosts at several time points during disease progression.
Male HSCT host mice [C.B10-H2b/LiMcdJ (BALB.B)], and female HSCT donors of bone marrow and spleen cells [C57BL/6 (B6)] were obtained from the Jackson Laboratory (Bar Harbor, ME, USA). The mice were housed under specific pathogen-free conditions at the Biomedical Center for Animal Resource Development of Seoul National University College of Medicine in Korea. All experiments were performed under the approval from The Seoul National University Institutional Animal Care and Use Committee (IACUC).
BM cells were prepared by flushing the femurs and tibiae from female B6 mice with 1× PBS. Splenocytes from female B6 mice were prepared by grinding the spleens through a steel mesh. Host male BALB.B mice were irradiated with 900 cGy 137Cs with split dosages at 5-h intervals. At 5 h after the second irradiation, the preconditioned host mice were injected in their lateral tail veins with a 300µl volume mixture of B6 MACS-depleted TCD-BM (5×106) and MACS-enriched T cells (3×106 or 6×106) from the spleens of B6 mice according to the manufacturer's protocol (Miltenyi Biotec, Auburn, CA, USA).
To deplete circulating PBLs, BALB.B GVHD hosts were perfused with PBS/heparin (75 U/ml; Sigma-Aldrich, St. Louis, MO, USA) before sacrifice. Leukocytes in the lymphoid organs were obtained by grinding the spleens and mesenteric lymph nodes (mLNs) through steel and cotton mesh, respectively. Leukocytes infiltrating the liver and lung target organs were prepared as described previously (19). In brief, the livers were pressed through stainless steel mesh and suspended in 5% FBS-DMEM (HyClone, Logan, Utah, USA). The cell suspensions were mixed with 33% Percoll (GE Healthcare, Piscataway, NJ, USA) containing 100 U/mL heparin and then centrifuged at 2000 rpm for 15 min at room temperature. The cell suspensions were applied to Percoll gradients, subjected to RBC lysis, and then resuspended in 5% FBS-DMEM. Lungs were cut into small pieces and treated with collagenase at 37℃ for 45 min after washing with HBSS/10 mM HEPES (Gibco Life Technologies, Carlsbad, CA, USA). After washing twice with 5% FBS-DMEM, the cells were applied to Percoll gradients. Cells were resuspended in 5% FBS-DMEM after washing with 1× PBS.
The fresh PBLs, splenocytes, or lymphocytes that infiltrated the liver and lung target organs in GVHD hosts were incubated at 4℃ for 30 min in FACS buffer (1× PBS with 0.1% bovine calf serum and 0.05% sodium azide) containing FITC- and APC-conjugated anti-CD4 and anti-CD8 (eBioscience, San Diego, CA, USA) monoclonal antibodies, respectively. Other monoclonal antibodies used were PE- or APC-conjugated anti-CD11b (M1/70, eBioscience), FITC-conjugated anti-Gr-1 (RB6-8C5, eBioscience), and PE-conjugated anti-CD4 (GK1.5, eBioscience). For intracellular cytokine analysis, reactivated cultures were stimulated with 20 ng/ml PMA and 1µM ionomycin (Sigma-Aldrich) plus Brefeldin A (Biolegend, San Diego, CA, USA) for 6 h. Cells were stained with anti-CD4 or anti-CD8 antibodies, washed with 1× PBS, and then fixed with 4% paraformaldehyde at room temperature for 20 min. After washing with 1× PBS, the cells were permeabilized with saponin and stained with APC-conjugated anti-IFN-γ (XMG1.2, eBioscience) or PE-conjugated IL-17 (IL-17A; TC11-18H10, BD Bioscience, San Diego, CA, USA) antibodies at room temperature for 1 h. The stained cells were analyzed using FACS LSRII (BD Bioscience) and FlowJo software (TreeStar, Ashland, OR, USA).
Serum samples were collected from intraocular blood and stored at -70℃ after centrifugation (2,500×g for 10 min at 4℃) until analysis. The serum concentrations of IL-6, IL-17, and IFN-γ were measured using a commercial ELISA kit (Biolegend); 100µl serum sample and assay diluent were placed in each well of a 96-well plate coated with a monoclonal mouse IgG against IL-6, IL-17, and IFN-γ. The mixtures were incubated for 2 h at 4℃, followed by aspiration and three washes with washing buffer. Subsequently, 200µl conjugate solution was placed into each well and incubated for 2 h at room temperature. After washing each well four times with washing buffer, 200µl substrate solution prepared with equal amounts of stabilized hydrogen peroxide and tetramethylbenzidine was added for a 20 min reaction in the dark. The reaction was quenched by adding 50µl stop solution (2N H2SO4). Within 30 min, the optical density was measured at a wavelength of 450 nm using the Power Wave Xs (BioTek, Winooski, VT, USA). Serum concentrations of IL-6, IL-17 and IFN-γ were determined based on a standard concentration curve. The correlation coefficient (r) of the standard concentration curve was over 0.990.
Single-cell suspensions were prepared from the spleens of the GVHD hosts sacrificed on day 7 or 21. Cells were washed twice and filtered through a fine cotton mesh. Then the samples were enriched for either CD4 T cells with anti-CD4 biotin and anti-biotin magnetic beads or CD8 T cells with anti-CD8 biotin and anti-biotin magnetic beads using the MidiMACS® system (Miltenyi Biotec).
Total RNA was isolated from CD4 and CD8 T cells enriched from the splenocytes of the GVHD hosts by MACS using the Trizol reagent (Ambion, Carlsbad, CA, USA) according to the manufacturer's protocol, and 1µg total RNA was reverse transcribed using an Omniscript kit (Qiagen, Valencia, CA, USA). Real-time PCR was performed to determine the changes in mRNA expression of the IFN-γ and IL-17A cytokines. The following primer sets were used and validated by PrimerBank (http://pga.mgh.harvard.edu/primerbank/): β-actin (PrimerBank ID: 6671509a1), IFN-γ (PrimerBank ID: 145966741c1), and IL-17A (PrimerBank ID: 6754324a1). The PCR reaction mixtures consisted of 1× SYBR Green PCR premix (Bioneer, Daejeon, Republic of Korea), 10 pM specific primers, and 2µl cDNA in the Bioneer Exicycler Real-Time PCR system (Bioneer). The thermal cycling conditions were 50℃ for 2 min and an initial denaturation step at 95℃ for 10 min followed by 45 cycles at 95℃ for 30 s, 56℃ for 30 s and 72℃ for 30 s. The mean cycle threshold (CT) values from triplicate (n=3) measurements were used to calculate gene expression, with normalization to β-actin as the internal control. The relative quantification in gene expression was determined using the 2-ΔΔCt method.
All data are presented as mean±SD. The unpaired Student's t-test was used for comparisons between groups, with a p-value less than 0.05 considered significant. Animal experiments had 3~5 mice/group repeated two or more times per experiment, and dot plots of flow cytometry are shown as representative data. All figures and statistical analysis were prepared using GraphPad Prism (GraphPad Software Inc., La Jolla, CA, USA).
To characterize the kinetics of alloreactive CD4 and CD8 T cells during GVHD progression, we first determined the number of T cells required to induce mild acute GVHD. We assessed the impact of different T cell numbers on GVHD survival and symptom severity in the B6 → BALB.B GVHD model. High (6×106) and low (3×106) numbers of T cells purified from B6 mice were injected together with B6 TCD-BM cells (5×106) into lethally irradiated (900 cGy) allogeneic BALB.B mice, and the survival and development of symptoms were assessed periodically. The BALB.B mice transplanted with high numbers of T cells showed short median survival (20 days) with steep weight loss and more severe GVHD symptoms than those transplanted with low numbers of T cells, which showed 66.5 days of median survival (Fig. 1A). Therefore, we selected the low T cell number to induce GVHD because dissecting the dynamics of cytokine production of donor CD4 and CD8 T cells during GVHD progression required a survival time of longer than 30 days. High and low numbers of transplanted T cells failed to induce GVHD in syngeneic control transplantation (B6 → B6).
Several cytokines such as TNF-α, IFN-γ, IL-6, and IL-17 are associated with the progression of GVHD (22). To assess the dynamics of the systemic release of these cytokines in BALB.B GVHD hosts transplanted with 3×106 B6 T cells, we measured the serum levels of IFN-γ, IL-6, and IL-17 using ELISA at different time points after the transplantation during GVHD progression. The levels of IFN-γ, IL-6, and IL-17 in the allogeneic GVHD hosts peaked on day 7 post-transplantation (Fig. 1B). Then, the amounts of IFN-γ and IL-6 in allogeneic hosts became comparable with those of the syngeneic hosts, whereas IL-17 levels remained higher in the allogeneic hosts, even though they decreased over time after day 7 post-transplantation.
Next, we investigated the compositional changes in the PBLs of the GVHD hosts during disease progression, because the proportions of CD11b+Gr-1+ innate immune cells are known to increase with GVHD progression (19). Blood was collected on days 7 and 21 post-transplantation from the BALB.B allogeneic or B6 syngeneic hosts of B6 BM and T cell (3×106) transplantation. The profiles were analyzed by flow cytometry for the proportions of CD4 and CD8 T cells and the Gr1+CD11b+ myeloid cells (Fig. 1C). Compared to the syngeneic B6 hosts, allogeneic BALB.B hosts showed significantly higher proportions of CD4 T cells in the blood on day 7 post-transplantation, which decreased on day 21 post-transplantation. In addition, the relative CD4/CD8 ratios decreased in the allogeneic hosts (Fig. 1D). However, increased proportions of Gr-1+CD11b+ myeloid cell populations (both Gr-1hiCD11b+ and Gr-1loCD11b+) in the blood of allogeneic BALB.B hosts were observed on day 21 post-transplantation compared to those of syngeneic hosts (Fig. 1B). These results demonstrate that donor T cells, especially CD4 T cells, proliferated more vigorously than CD8 T cells in the allogeneic hosts early after transplantation. However, as GVHD progressed, the CD4/CD8 ratio reversed (Fig. 1D) due to increased CD8 T cell numbers, and the proportions of Gr-1hiCD11b+ and Gr-1loCD11b+ myeloid cells increased. These changes were consistent with those observed with GVHD progression in BALB.B mice induced with whole BM and total splenocyte injections (19,21).
The injection of 3×106 T cells and 5×106 TCD-BM cells isolated from B6 mice into the irradiated BALB.B hosts induced mild acute GVHD with an appropriate mean survival time. Furthermore, evidence of GVHD disease progression included systemic inflammatory cytokine secretion and expansion of CD4 and CD8 T cells and myeloid cells.
Secondary lymphoid organs such as the mLN, spleen, and Peyer's patches are thought to be the initial sites of acute GVHD, in which the donor T cells are activated to proliferate (23,24) before infiltrating and destroying the target tissue in the host (25).
To understand the dynamics of allogeneic donor CD4 and CD8 T cells in the organs of the BALB.B GVHD host, we assessed the fractions and absolute numbers of CD4 and CD8 T cells in the secondary lymphoid organs (spleen and mLN) and the GVHD target organs (liver and lung) on days 7 and 21 post-transplantation. High proportions of CD4 T cells in the organs of allogeneic BALB.B hosts were observed on day 7, which were reduced on day 21 post-transplantation (Fig. 2A) probably due to increased proportions of Gr-1+CD11b+ myeloid cells during GVHD progression. Despite the reduced proportions, increased numbers of donor CD4 T cells were observed in each organ over time, likely because of the increased total cellularity, especially in the mLN, liver, and lung of the allogeneic BALB.B GVHD host. A similar trend was observed with CD8 T cells, indicating increased infiltration of donor CD4 and CD8 T cells into the peripheral target organs during the GVHD progression. In addition, decreased relative ratios of CD4/CD8 were observed in each organ on day 21 compared to those on day 7 post-transplantation in the BALB.B GVHD hosts (Fig. 2C). Such decreases in CD4/CD8 ratio values in BALB.B allogeneic hosts over time contrasted with the high values maintained in the syngeneic hosts, implying that more vigorous CD8 T cell expansion was associated with GVHD progression.
To understand the dynamics of IFN-γ production by CD4 and CD8 T cells in GVHD progression, we investigated and compared the IFN-γ production by donor CD4 and CD8 T cells in the organs of the allogeneic and syngeneic hosts. Leukocytes infiltrating the spleen, mLN, liver, and lung were prepared from four BALB.B or B6 hosts on days 7 and 21 post-transplantation. The harvested cells were pooled and activated with PMA and ionomycin for 6 h in vitro and analyzed by flow cytometry for their production of IFN-γ. The flow cytometric analyses showed that although the donor CD4 T cells produced IFN-γ in the organs of both the allogeneic and syngeneic hosts, higher proportions of IFN-γ-producing CD4 T cells were found in the organs of allogeneic hosts than in those of the syngeneic hosts, which decreased over time during GVHD progression from 7 to 21 days post-transplantation. However, no appreciable changes in IFN-γ-producing CD4 T cell numbers were observed between days 7 and 21 post-transplantation. We noted that the number of mLNs in the allogeneic hosts continued to increase (Fig. 3A), and the numbers of IFN-γ-producing CD4 T cells in the mLNs increased during the period.
The proportions of IFN-γ-producing CD8 T cells showed similar trends to those of CD4 T cells. However, the numbers of IFN-γ-producing CD8 T cells increased over time in all organs of allogeneic hosts (Fig. 3B). Therefore, GVHD progression in the allogeneic hosts was associated with decreased relative T cell ratios of CD4/CD8 cells in IFN-γ-producing T cells (Fig. 3C).
Flow cytometric analyses of IL-17 production by CD4 and CD8 T cells during GVHD progression showed that proportions and numbers of IL-17-producing CD4 T cells increased in all organs of the allogeneic hosts during GVHD progression from 7 to 21 days post-transplantation (Fig. 4A), and higher proportions and numbers of IL-17-producing CD4 T cells were observed in the allogeneic hosts than the syngeneic hosts. We found increased numbers of IL-17-producing CD4 T cells in the spleen of syngeneic hosts on day 21 post-transplantation, which was due to increased CD4 and CD8 T cell reconstitution in the spleen of syngeneic hosts at later time points post-transplantation. The increased proportions and numbers of IL-17-producing CD4 T cells in allogeneic hosts indicated that CD4 T cell differentiation tended to shift from Th1 to Th17 cells during GVHD progression, especially in the liver and lung target organs.
Although we observed increased proportions and numbers of CD8 T cells over time during GVHD progression, the changes in IL-17-producing cells were not statistically significant (Fig. 4B). Therefore, the relative CD4/CD8 T cell ratios in IL-17-producing cells were always higher than 1 at both time points after transplantation (Fig. 4C). Due to the significant increase in the number of IL-17-producing CD4 T cells in the liver and lung of the allogeneic hosts during GVHD progression, the relative CD4/CD8 T cell ratios in IL-17-producing cells increased in the target organs over time. No significant production of IL-17 by CD4 and CD8 T cells was observed in the syngeneic hosts.
We suggest that production of IFN-γ by CD4 T cells precedes that of IL-17 during GVHD progression and that the production of cytokines by alloreactive CD4 T cells precedes that by alloreactive CD8 T cells.
Flow cytometric analyses showed that the donor CD4 and CD8 T cells produced IFN-γ and IL-17 in the allogeneic hosts with different kinetics. To confirm these results, we performed quantitative RT-PCR using the CD4 and CD8 T cells purified from the spleen of allogeneic or syngeneic hosts after B6 TCD BM and T cell transplantation. There was a two- to three-fold reduction in the amount of IFN-γ transcripts (Fig. 5A) in the CD4 T cells in the allogeneic hosts as GVHD progressed from days 7 to 21 post-transplantation, and a fourfold enhancement of IL-17 (Fig. 5B) transcripts during the period. The amounts of IFN-γ transcripts (Fig. 5A) remained constant in CD8 T cells during this timeframe, while those of IL-17 (Fig. 5B) increased slightly. In addition, higher amounts of IFN-γ and IL-17 transcripts were observed in the allogeneic case than in the syngeneic case. These results confirmed the different dynamics in the production of the two cytokines by CD4 and CD8 T cells during GVHD progression, demonstrating that Th1, Th17, Tc1, and Tc17 responses develop with different kinetics during GVHD development.
Using an MHC-matched but minor H antigen-mismatched model, we investigated the dynamics of CD4 and CD8 T cell production of two major cytokines involved in GVHD pathogenesis, IFN-γ and IL-17. The production of the cytokines by CD4 T cells preceded that by CD8 T cells, and Th1 and Tc1 differentiation occurred prior to Th17 and Tc17 differentiation. These results demonstrate that these cytokines are produced with different kinetics, suggesting an evolution of CD4 and CD8 T cell differentiation during GVHD progression.
Allogeneic minor H antigen-specific CD4 and CD8 T cells are considered to play roles in GVHD pathogenesis (19,26). The results from this study provide direct evidence for enhanced production of IFN-γ and IL-17 by donor CD4 and CD8 T cells in the allogeneic GVHD hosts via ex vivo analysis of T cells isolated from the various organs of the GVHD hosts.
Acute GVHD can be conceptualized as a three-stage process consisting of preconditioned initiation of tissue damage (e.g., total body irradiation), activation and proliferation of donor T cells after allo-antigen recognition, and the effector phase, resulting in tissue damage (27). During the early stage after HSCT, alloreactive T cells produce proinflammatory cytokines that play a critical role in GVHD pathophysiology by eliciting the activation of immune cells and causing direct tissue damage (2,28). However, we found that cytokines were also produced by CD4 and CD8 T cells in the syngeneic hosts, suggesting that cytokines, especially IFN-γ, could be produced by CD4 and CD8 T cells even without activation after antigen-recognition under inflammatory conditions (4,29) which were induced by total body irradiation. Activation of CD4 and CD8 T cells would augment the production of the cytokines in each cell through effector differentiation after activation via recognition of allo-antigens.
Serum levels of IL-17 peaked on day 7 post-transplantation, at which time alloreactive CD4 or CD8 T cells showed little Il-17 gene transcription. Therefore, the IL-17 secreted in blood at day 7 in the allogeneic hosts could be ascribed to the production by innate immune cells originated from transplanted BM cells due to tissue damage by high-dose irradiation (21) and by attack from alloreactive T cells. Then, the relatively higher level of IL-17 secreted in the serum of allogeneic hosts than in syngeneic hosts at later time points (days 14 and 21 post-transplantation) would be ascribed to the IL-17-production by the alloreactive CD4 T cells. However, systemic release to blood would not be distinct at the late time points because IL-17 production was more prominent at local tissue sites.
Th1 and Th2 responses were up-regulated early after BM transplantation, followed by a subsequent up-regulation of Th17 cells during acute and chronic GVHD progression (30). Significant IL-17 production was observed at day 21, especially in the GVHD liver and lung target organs. Therefore, differentiation toward Th17 is associated with local tissue damages such as cutaneous and pulmonary lesions (14) during disease development. In experimental autoimmune encephalomyelitis (EAE), IL-17-producing CD4 T cells are prominent in the brain compared to secondary lymphoid organs (31), consistent with our data. Furthermore, IFN-γ-producing CD4 T cells enter the CNS and modify conditions therein, making it both attractive and accessible to IL-17-producing CD4 T cells, resulting in tissue damage in the EAE model (32). In this respect, the shift of CD4 T cell differentiation from Th1 to Th17 could be related to organ pathology in the progression of GVHD.
Consistent with the previous notion that Th1 and Th17 CD4 T cells and Tc1 and Tc17 CD8 T cells contribute considerably to GVHD pathology (7,33), our data demonstrated cytokine production by the two types of T cells. In addition, the observed production of IFN-γ and IL-17 by CD4 T cells before production by CD8 T cells implies a cytokine-producing helper function of activated CD4 T cells in promoting CD8 T cell effector differentiation. On day 21 post-transplantation, the transcriptional activity for IFN-γ production by CD8 T cells and the numbers of IFN-γ-producing cells remained high, while the IL-17 transcriptional activity and the numbers of IL-17-producing CD4 T cells were enhanced. This implies a possible influence of cytolytic activity of CD8 T cells on a shift to Th17 differentiation of CD4 T cells in target organs, suggesting the possible presence of a reciprocal influence between activated CD4 and CD8 T cells during GVHD progression. As Th1 and Th17 are involved in different stages of tissue pathology (8,13,16,34), further detailed investigations of the dynamic role of CD4 and CD8 T cells in the target organs might expand our understanding of GVHD progression.
In summary, we showed that the dynamics of CD4 and CD8 T cell production of IFN-γ and IL-17 differed during B6 → BALB.B GVHD development. The results from this study provide useful insights regarding the pathophysiology of GVHD.
Figures and Tables
Figure 1
Establishment of minor H antigen-disparate graft-versus-host-disease (GVHD) model using T-cell depleted (TCD) bone marrow (BM) and purified T cells. (A) BALB.B (●) or B6 (○) mice were lethally irradiated and infused with 5×106 TCD BM with 6×106 or 3×106 MACS-enriched splenic T cells from B6 mice; n=10 mice/group. Survival rate is shown. (B) BALB.B (■) or B6 (□) mice were lethally irradiated and infused with 5×106 TCD BM with 3×106 MACS-enriched splenic T cells from B6 mice; n=10 mice/group. Sera were collected by centrifugation of the blood from host mice. Host serum cytokine levels of IFN-γ, IL-6, and IL-17 were measured by ELISA on days 4, 7, 14, and 21 post-transplantation. (C) T cells and myeloid cell fractions in the peripheral blood from allogeneic and syngeneic hosts on days 7 and 21. PBLs were pooled and stained with anti-CD4, CD8, Gr-1, and CD11b antibodies and analyzed by flow cytometry. Data representing more than three independent experiments are shown; n=10 mice/group. (D) PBLs from allogeneic and syngeneic hosts on days 7 and 21 were labeled with anti-CD4 and anti-CD8 antibodies, and the CD4/CD8 ratios were compared. Four mice were analyzed in each group. ***p<0.001, **p<0.01. Error bars represent the mean±SD.
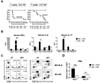
Figure 2
The local dynamics of cellular composition during mild acute GVHD. Leukocytes infiltrating the spleen, mesenteric lymph node (mLN), liver, and lung of the GVHD hosts received TCD-BM cells. A total of 3×106 splenic T cells from B6 mice were prepared on days 7 and 21 post-transplantation and T cell fractions were analyzed using flow cytometry after staining with anti-CD4 and CD8 antibodies. (A) Representative flow cytometry data for CD4+ and CD8+ T cells obtained from allogeneic and syngeneic hosts on days 7 and 21 are shown. (B) Absolute numbers of CD4 and CD8 T cells in the secondary lymphoid organs (spleen and mLN) and target organs (liver and lung) from allogeneic and syngeneic hosts on days 7 and 21 are plotted. Data representing more than three independent experiments are shown. (C) T cells from the spleen, mLN, liver, and lung target organs from allogeneic or syngeneic hosts on day 7 and day 21 were labeled with anti-CD4 and anti-CD8 antibodies, and the CD4/CD8 ratios were compared. Four mice were analyzed in each group. ***p<0.001, **p<0.01, *p<0.05. Error bars represent the mean±SD.
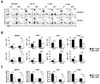
Figure 3
Dynamics of IFN-γ production by CD4 and CD8 T cells infiltrating the organs of GVHD hosts. Lymphocytes were prepared from secondary lymphoid organs (spleen and mLN) and target organs (liver and lung) of BALB.B and B6 host mice that received TCD BM and 3×106 splenic T cells from B6 mice on days 7 and 21 post-transplantation. The lymphocytes were analyzed for cytokine production by flow cytometry after intracytoplasmic staining with anti-IFN-γ in combination with anti-CD4 and anti-CD8 antibodies. (A) IFN-γ and CD4 profiles in CD4 T cells (top) and percentages and absolute numbers of the IFN-γ-producing CD4 T cells (bottom) from the spleen, mLN, liver, and lung target organs on day 7 and 21 post-GVHD progression are plotted. (B) IFN-γ and CD8 profiles in CD8 T cells (top) and percentages and absolute numbers of the IFN-γ-producing CD8 T cells (bottom) from the spleen, mLN, liver, and lung target organs on days 7 and 21 post-GVHD progression are plotted. (C) T cells from spleen, mLN, liver, and lung target organs from allogeneic and syngeneic hosts on days 7 and 21 were labeled with anti-CD4, CD8, and IFN-γ antibodies, and the CD4/CD8 ratios of the IFN-γ+ cells were compared. Four mice were analyzed in each group. The data represent more than three independent experiments. ***p<0.001, **p<0.01, *p<0.05. Error bars represent the mean±SD.
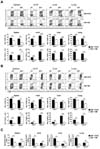
Figure 4
Dynamics of IL-17 production by CD4 and CD8 T cells infiltrating the organs of GVHD hosts. Lymphocytes were prepared as Fig. 3. The lymphocytes were analyzed for cytokine production by flow cytometry after intracytoplasmic staining with anti-IL-17 in combination with anti-CD4 and anti-CD8 antibodies. (A) IL-17 and CD4 profiles of CD4 T cells (top) and percentages and absolute numbers of IL-17-producing CD4 T cells (bottom) from the spleen, mLN, liver, and lung target organs on days 7 and 21 post-GVHD progression are plotted. (B) IL-17 and CD8 profiles of CD8 T cells (top) and percentages and absolute numbers of the IL-17-producing CD8 T cells (bottom) from the spleen, mLN, liver, and lung target organs on days 7 and 21 post-GVHD progression are plotted. (C) T cells from the spleen, mLN, liver, and lung target organs of allogeneic and syngeneic hosts on days 7 and 21 were labeled with anti-CD4, CD8 and IL-17 antibodies, and the CD4/CD8 ratios of IL-17+ cells were compared. Four mice were analyzed in each group. The data represent more than three independent experiments. ***p<0.001, **p<0.01, *p<0.05. Error bars represent the mean±SD.
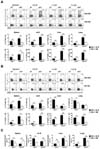
Figure 5
Dynamics of cytokine production in CD4 and CD8 T cells. Levels of IFN-γ (A) and IL-17 (B) mRNA transcripts were determined using quantitative real-time RT-PCR with MACS-enriched splenic CD4 and CD8 T cells from each host on days 7 and 21. Fold changes in IFN-γ and IL-17 mRNA expression levels compared to β-actin as the internal control were calculated using the 2-ΔΔCt method. The data represent more than three independent experiments. **p<0.01, *p<0.05. Data performed in triplicate are presented as the mean±SD; n=6 mice/group.
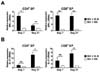
ACKNOWLEDGEMENTS
This work was supported by a grant from the Korea Healthcare Technology R & D Project, Ministry of Health, Welfare, and Family Affairs, Republic of Korea (Grant no. A120273).
References
1. Blazar BR, Murphy WJ, Abedi M. Advances in graft-versus-host disease biology and therapy. Nat Rev Immunol. 2012; 12:443–458.


3. Filipovich AH, Weisdorf D, Pavletic S, Socie G, Wingard JR, Lee SJ, Martin P, Chien J, Przepiorka D, Couriel D, Cowen EW, Dinndorf P, Farrell A, Hartzman R, Henslee-Downey J, Jacobsohn D, McDonald G, Mittleman B, Rizzo JD, Robinson M, Schubert M, Schultz K, Shulman H, Turner M, Vogelsang G, Flowers ME. National Institutes of Health consensus development project on criteria for clinical trials in chronic graft-versus-host disease: I. Diagnosis and staging working group report. Biology of blood and marrow transplantation. Biol Blood Marrow Transplant. 2005; 11:945–956.


4. Socie G, Blazar BR. Acute graft-versus-host disease: from the bench to the bedside. Blood. 2009; 114:4327–4336.


5. Sun Y, Tawara I, Toubai T, Reddy P. Pathophysiology of acute graft-versus-host disease: recent advances. Transl Res. 2007; 150:197–214.


6. Ferrara JL, Levy R, Chao NJ. Pathophysiologic mechanisms of acute graft-vs.-host disease. Biol Blood Marrow Transplant. 1999; 5:347–356.


7. Iclozan C, Yu Y, Liu C, Liang Y, Yi T, Anasetti C, Yu XZ. T helper17 cells are sufficient but not necessary to induce acute graft-versus-host disease. Biol Blood Marrow Transplant. 2010; 16:170–178.


8. Yi T, Chen Y, Wang L, Du G, Huang D, Zhao D, Johnston H, Young J, Todorov I, Umetsu DT, Chen L, Iwakura Y, Kandeel F, Forman S, Zeng D. Reciprocal differentiation and tissue-specific pathogenesis of Th1, Th2, and Th17 cells in graft-versus-host disease. Blood. 2009; 114:3101–3112.


9. Yang YG, Dey BR, Sergio JJ, Pearson DA, Sykes M. Donor-derived interferon gamma is required for inhibition of acute graft-versus-host disease by interleukin 12. J Clin Invest. 1998; 102:2126–2135.


10. Dong C. TH17 cells in development: an updated view of their molecular identity and genetic programming. Nat Rev Immunol. 2008; 8:337–348.


11. Antonysamy MA, Fanslow WC, Fu F, Li W, Qian S, Troutt AB, Thomson AW. Evidence for a role of IL-17 in alloimmunity: a novel IL-17 antagonist promotes heart graft survival. Transplant Proc. 1999; 31:93.


12. Loong CC, Hsieh HG, Lui WY, Chen A, Lin CY. Evidence for the early involvement of interleukin 17 in human and experimental renal allograft rejection. J Pathol. 2002; 197:322–332.


13. Serody JS, Hill GR. The IL-17 differentiation pathway and its role in transplant outcome. Biol Blood Marrow Transplant. 2012; 18:S56–S61.


14. Carlson MJ, West ML, Coghill JM, Panoskaltsis-Mortari A, Blazar BR, Serody JS. In vitro-differentiated TH17 cells mediate lethal acute graft-versus-host disease with severe cutaneous and pulmonary pathologic manifestations. Blood. 2009; 113:1365–1374.


15. Kappel LW, Goldberg GL, King CG, Suh DY, Smith OM, Ligh C, Holland AM, Grubin J, Mark NM, Liu C, Iwakura Y, Heller G, van den Brink MR. IL-17 contributes to CD4-mediated graft-versus-host disease. Blood. 2009; 113:945–952.


16. Yi T, Zhao D, Lin CL, Zhang C, Chen Y, Todorov I, LeBon T, Kandeel F, Forman S, Zeng D. Absence of donor Th17 leads to augmented Th1 differentiation and exacerbated acute graft-versus-host disease. Blood. 2008; 112:2101–2110.


17. Yen HR, Harris TJ, Wada S, Grosso JF, Getnet D, Goldberg MV, Liang KL, Bruno TC, Pyle KJ, Chan SL, Anders RA, Trimble CL, Adler AJ, Lin TY, Pardoll DM, Huang CT, Drake CG. Tc17 CD8 T cells: functional plasticity and subset diversity. J Immunol. 2009; 183:7161–7168.


18. Lai HY, Chou TY, Tzeng CH, Lee OK. Cytokine profiles in various graft-versus-host disease target organs following hematopoietic stem cell transplantation. Cell Transplant. 2012; 21:2033–2045.


19. Choi EY, Christianson GJ, Yoshimura Y, Jung N, Sproule TJ, Malarkannan S, Joyce S, Roopenian DC. Real-time T-cell profiling identifies H60 as a major minor histocompatibility antigen in murine graft-versus-host disease. Blood. 2002; 100:4259–4265.


20. Song MG, Kang B, Jeon JY, Chang J, Lee S, Min CK, Youn H, Choi EY. In Vivo imaging of differences in early donor cell proliferation in graft-versus-host disease hosts with different pre-conditioning doses. Mol Cells. 2012; 33:79–86.


21. Choi JH, Yoon H, Min CK, Choi EY. Effects of pre-conditioning dose on the immune kinetics and cytokine production in the leukocytes infiltrating GVHD tissues after MHC-matched transplantation. Immune Netw. 2011; 11:68–78.


22. Toubai T, Tanaka J, Paczesny S, Shono Y, Reddy P, Imamura M. Role of cytokines in the pathophysiology of acute graft-versus-host disease (GVHD): are erum/plasma cytokines potential biomarkers for diagnosis of acute GVHD following allogeneic hematopoietic cell transplantation (Allo-HCT)? Curr Stem Cell Res Ther. 2012; 7:229–239.


23. Murai M, Yoneyama H, Ezaki T, Suematsu M, Terashima Y, Harada A, Hamada H, Asakura H, Ishikawa H, Matsushima K. Peyer's patch is the essential site in initiating murine acute and lethal graft-versus-host reaction. Nat Immunol. 2003; 4:154–160.


24. Panoskaltsis-Mortari A, Price A, Hermanson JR, Taras E, Lees C, Serody JS, Blazar BR. In vivo imaging of graft-versus-host-disease in mice. Blood. 2004; 103:3590–3598.


25. Beilhack A, Schulz S, Baker J, Beilhack GF, Wieland CB, Herman EI, Baker EM, Cao YA, Contag CH, Negrin RS. In vivo analyses of early events in acute graft-versus-host disease reveal sequential infiltration of T-cell subsets. Blood. 2005; 106:1113–1122.


26. Koyama M, Kuns RD, Olver SD, Raffelt NC, Wilson YA, Don AL, Lineburg KE, Cheong M, Robb RJ, Markey KA, Varelias A, Malissen B, Hämmerling GJ, Clouston AD, Engwerda CR, Bhat P, MacDonald KP, Hill GR. Recipient nonhematopoietic antigen-presenting cells are sufficient to induce lethal acute graft-versus-host disease. Nat Med. 2011; 18:135–142.


27. Goker H, Haznedaroglu IC, Chao NJ. Acute graft-vs-host disease: pathobiology and management. Exp Hematol. 2001; 29:259–277.


28. Visentainer JE, Lieber SR, Persoli LB, Vigorito AC, Aranha FJ, de Brito Eid KA, Oliveira GB, Miranda EC, de Souza CA. Serum cytokine levels and acute graft-versus-host disease after HLA-identical hematopoietic stem cell transplantation. Exp Hematol. 2003; 31:1044–1050.


29. Hill GR, Crawford JM, Cooke KR, Brinson YS, Pan L, Ferrara JL. Total body irradiation and acute graft-versus-host disease: the role of gastrointestinal damage and inflammatory cytokines. Blood. 1997; 90:3204–3213.


30. Nishimori H, Maeda Y, Teshima T, Sugiyama H, Kobayashi K, Yamasuji Y, Kadohisa S, Uryu H, Takeuchi K, Tanaka T, Yoshino T, Iwakura Y, Tanimoto M. Synthetic retinoid Am80 ameliorates chronic graft-versus-host disease by down-regulating Th1 and Th17. Blood. 2012; 119:285–295.


31. Park H, Li Z, Yang XO, Chang SH, Nurieva R, Wang Y-H, Wang Y, Hood L, Zhu Z, Tian Q, Dong C. A distinct lineage of CD4 T cells regulates tissue inflammation by producing interleukin 17. Nat Immunol. 2005; 6:1133–1141.


32. O'Connor RA, Prendergast CT, Sabatos CA, Lau CW, Leech MD, Wraith DC, Anderton SM. Cutting edge: Th1 cells facilitate the entry of Th17 cells to the central nervous system during experimental autoimmune encephalomyelitis. J Immunol. 2008; 181:3750–3754.