Abstract
TGF-β induces IgA class switching by B cells. We previously reported that Smad3 and Smad4, pivotal TGF-β signal-transducing transcription factors, mediate germline (GL) α transcription induced by TGF-β1, resulting in IgA switching by mouse B cells. Post-translational sumoylation of Smad3 and Smad4 regulates TGF-β-induced transcriptional activation in certain cell types. In the present study, we investigated the effect of sumoylation on TGF-β1-induced, Smad3/4-mediated GLα transcription and IgA switching by mouse B cell line, CH12F3-2A. Overexpression of small ubiquitin-like modifier (SUMO)-1, SUMO-2 or SUMO-3 did not affect TGF-β1-induced, Smad3/4-mediated GLα promoter activity, expression of endogenous GLα transcripts, surface IgA expression, and IgA production. Next, we tested the effect of the E3 ligase PIASy on TGF-β1-induced, Smad3/4-mediated GLα promoter activity. We found that PIASy overexpression suppresses the GLα promoter activity in cooperation with histone deacetylase 1. Taken together, these results suggest that SUMO itself does not affect regulation of GLα transcription and IgA switching induced by TGF-β1/Smad3/4, while PIASy acts as a repressor.
Under the influence of antigens and cytokines, Ig class switch recombination (CSR) on the heavy chain locus in IgM+ mature B cells allows the cells to produce other Ig isotypes (IgG, IgA, and IgE). IL-4, IFN-γ, and TGF-β1 induce IgG1/IgE, IgG2a/IgG3, and IgA/IgG2b class switching by mouse B cells, respectively (1). Each transcription of germline transcripts (GLTs) in the switch regions is a prerequisite for each Ig CSR process (2). For instance, selective induction of GLα transcription by TGF-β1 initiates IgA CSR by increasing the accessibility of activation-induced cytidine deaminase, which is an essential enzyme for Ig CSR (3), to a non-transcribed DNA strand of the switch region. TGF-β1 induces IgA class switching through Smad3/4-mediated GLα transcription (4). We previously reported that many proteins such as Runx3, p300, Smurf, Arkadia, Tiul1, TGIF, and Stat1 are involved in the regulation of TGF-β1-induced, Smad3/4-mediated GLα transcription by B cells (5,6,7,8).
The Smad proteins are key intracellular effectors of TGF-β signaling pathways that encompass a diverse set of cellular responses, including cell proliferation and differentiation. Post-translational modifications of Smad proteins regulate their function. Phosphorylation of receptor-regulated Smads (Smad2 and Smad3) induces hetero-oligomeric formation. Ubiquitination and acetylation of Smads are also required for Smads-dependent gene regulation. Another type of posttranslational modification, sumoylation, has been shown to be important for TGF-β signaling pathways (9). Sumoylation is a process in which a small ubiquitin-like modifier (SUMO), a ubiquitin-related polypeptide, covalently binds to lysine residues of the target proteins and thereby regulates the function of proteins engaged in diverse processes. Three SUMO proteins, SUMO-1, SUMO-2, and SUMO-3, are expressed in higher eukaryotes. SUMO-2 and SUMO-3 share 97% similarity with each other, but only 50% similarity with the most studied SUMO-1 protein (10). Sumoylation is mediated through a process involving an E2 ubiquitin carrier protein (Ubc9) and five protein inhibitor of activated STAT (PIAS) family E3 ubiquitin ligases (PIAS1, PIAS3, PIASxα, PIASxβ, and PIASy). This post-translational modification can affect target protein interaction, localization, and function (11).
SUMO-1 induces Smad4 sumoylation through TGF-β-induced p38 MAP kinase pathway activation and facilitates Smaddependent transcriptional activation, and PIAS1/PIASxβ-mediated sumoylation of Smad4 is also regulated by the p38 MAP kinase pathway (12). Smad4 is sumoylated in a process involving Ubc9 and PIASy (13,14,15), PIAS1 (12,16), and PIASxβ (12). The sumoylation of Smad4 alters its subnuclear localization, enhances its stability, and increases Smad4-mediated transcriptional activation (13,14,15). Furthermore, the interaction between PIAS1 and Smad4 is positively regulated by TGF-β, thus creating a positive feedback loop (16). Association of Smad3 and Smad7 with PIASy leads to increased sumoylation of Smad3 (17). PIASy suppresses TGF-β/Smad3-mediated signaling by stimulating sumoylation and nuclear export of Smad3 (18). PIASy also can inhibit TGF-β/Smad-mediated transcriptional responses by interacting with Smads and histone deacetylase 1 (HDAC1) (19). On the other hand, PIAS3 activates Smad transcriptional activity through its interaction with Smads and p300 (20). In addition, sumoylation of type I TGF-β receptor enhances receptor function by facilitating the recruitment and phosphorylation of Smad3, consequently regulating TGF-β-induced transcription (21).
Thus, sumoylation of Smad3/4 by SUMO-1, Ubc9, and PIAS proteins regulates TGF-β/Smads-mediated transcriptional responses. Nonetheless, it is not known if the sumoylation-related molecules are involved in the regulation of GLα transcription by TGF-β1 in B cells. We report here for the first time the effects of SUMO proteins, Ubc9, and PIASy on TGF-β1-induced, Smad3/4-mediated GLα transcription and IgA switching by a mouse B cell line, CH12F3-2A.
The mouse B lymphoma cell line CH12F3-2A (surface Igµ+) (22) was provided by Dr. T. Honjo (Kyoto University, Kyoto, Japan). The cells were cultured at 37℃ in a humidified CO2 incubator (Forma Scientific, Marietta, OH, USA) in RPMI-1640 medium (WelGENE, Daegu, Korea) supplemented with 10% fetal bovine serum (PAA Laboratories, Etobicoke, ON, Canada). The cells were stimulated with TGF-β1 (0.5 ng/ml, R&D Systems, Minneapolis, MN, USA) and trichostatin A (TSA) (Sigma-Aldrich, St Louis, MO, USA).
Genes encoding Smad3 (23) and Smad4 (24) subcloned into Flag-pcDNA3, were provided by Dr. M. Kawabata (The Cancer Institute, Tokyo, Japan). The expression plasmid for SUMO-1 (pcDNA3-HA-SUMO-1) (12) was obtained from Dr. T. Ohshima (Tokushima Bunri University, Kagawa, Japan). Dr. F. Liu (The State University of New Jersey, NJ, USA) provided pcDNA3-HA-SUMO-2, pcDNA3-HA-SUMO-3, pcDNA3-HA-Ubc9, and pCMV5-FLAG-PIASy (25). The expression plasmid for HDAC1 (pK7-HDAC1-GFP) (26) was obtained from AddGene (Cambridge, MA, USA). The GLα -448 to +72 luciferase promoter was subcloned into pGL3-Basic Vector (Promega, Madison, WI, USA) (27) and was named pGL3-GLα [-448/+72].
CH12F3-2A cells were transfected as above with the appropriate expression vectors. After 24 h incubation, total cell lysates were subjected to SDS-PAGE under reducing conditions, and proteins were transferred to PVDF membranes (Bio-Rad). Specific immunodetection was carried out by incubation of the membranes with an anti-HA antibody (Sigma-Aldrich) followed by peroxidase-conjugated goat anti-mouse IgG antibody (Pierce, Thermo Scientific, Rockford, IL, USA), and visualized by chemiluminescence (Supersignal detection kit, Pierce).
RNA preparation, reverse transcription, and PCR were performed as described previously (4). PCR primers were synthesized by Bioneer (Daejeon, Korea): GLTα (forward 5'-CTACCATAGGGAAGATAGCCT-3', reverse 5'-TAATCGTGAATCAGGCAG-3', product size: 206 bp) and β-actin (forward 5'-CATGTTTGAGACCTTCAACACCCC-3', reverse 5'-GCCATCTCCTGCTCGAAGTCTAG-3', product size, 320 bp). All reagents for RT-PCR were purchased from iNtRON Biotechnology (Seongnam, Korea). PCR for β-actin was performed in parallel to normalize cDNA concentrations within each set of samples. Aliquots of the PCR products were resolved by electrophoresis on 2% agarose gels.
Cultured cells were washed with HBSS (WelGENE) and resuspended in DMEM (WelGENE) containing 5% FBS, 0.1% NaN3 at a density of 1×106 cells/ml. FITC-conjugated goat anti-mouse IgA (eBioscience, San Diego, CA, USA) and PE-conjugated goat anti-mouse IgM (eBioscience) were added to the cell suspension and placed at 4℃ for 30 min. Cells were washed with HBSS three times and resuspended in PBS containing 1% formalin. Cytofluorometric analysis was carried out using a FACSCalibur (Becton Dickinson, Mountain View, CA, USA).
Antibodies produced in B cell cultures were detected by using isotype-specific ELISA. Affinity purified anti-isotype specific antibodies were added at 1.2µg/ml in 0.05 M sodium bicarbonate buffer (pH 9.3) to 96-well U bottomed polyvinyl microplates (Falcon, Becton Dickinson & Co., Oxnard, CA, USA). Plates were washed with PBS containing 0.05% Tween-20 (PBST) followed by overnight incubation at 4℃, and blocked for 1 h with 0.5% BSA solution. After washing, 50µl of standard myeloma proteins and culture supernatants were added to each well and incubated for 1 h at 37℃. Following another washing step, horseradish-peroxidase (HRPO) conjugated anti-isotype specific antibodies (Southern Biotechnology, Birmingham, AL, USA) were added to each well and incubated for 1 h. Plates were then washed and TMB substrate (BD Biosciences) was added. After incubation, 0.05 M sulfuric acid was added to each well and the colorimetric reaction was measured at 450 nm with an Absorbance Microplate Reader.
In this study, we used a well-characterized mouse CH12F3-2A B lymphoma cell line to investigate IgA isotype switching (22,28). Smad3 plays a key role in TGF-β1-induced GLα transcription. Therefore, we first tried to address whether SUMO overexpression affects TGF-β1-induced, Smad3-mediated GLα promoter activity in CH12F3-2A cells. As shown in Fig. 1A (left panel), Smad3 overexpression enhanced TGF-β1-induced GLα promoter activity. However, overexpression of SUMO-1, SUMO-2, or SUMO-3 did not alter TGF-β1-induced, Smad3-mediated GLα promoter activity. We confirmed through detection of sumoylated proteins following transfection of SUMO-1 and SUMO-3 expression vectors that overexpression of SUMO proteins results in sumoylation of certain proteins (Fig. 1A, right panel). Smad4 physically interacts with Ubc9, and SUMO-1 overexpression enhances TGF-β-induced transcriptional responses by increasing intranuclear Smad4 level (13,14). Smad4 sumoylation was shown to increase in the presence of Ubc9 and PIASy, which enhances Smad4-mediated transcriptional activation (15). In contrast, it was reported that SUMO-1 and Ubc9 can inhibit a TGF-β-responsive transcriptional activity via sumoylation of Smad4 (25). The net effect of Smad4 sumoylation can therefore be either stimulatory or inhibitory, depending on distinct transcriptional effects of the target promoter that is analyzed. We next determined whether Ubc9 and SUMO-1 overexpression regulates Smad3/4-mediated GLα promoter activity induced by TGF-β1. As shown in Fig. 1B (left panel), Ubc9 hardly affected TGF-β1-induced, Smad3- or Smad4-mediated GLα promoter activity. In addition, Ubc9 and SUMO-1 affected neither the TGF-β1-induced GLα promoter activity nor Smad3/4-mediated promoter activity (Fig. 1B, right panel). Next, we investigated the effect of SUMO-1 overexpression on the expression of endogenous GLTα. As shown in Fig. 2A, Smad3 overexpression enhanced TGF-β1-induced GLTα expression, while SUMO-1 overexpression did not alter the expression. Furthermore, SUMO-1 overexpression had no effect on TGF-β1-induced, Smad3-mediated surface IgA expression and IgA production by CH12F3-2A cells (Fig. 2B and 2C). We further tested the effects of SUMO-2 and SUMO-3 overexpression on TGF-β1/Smad3-mediated GLα transcription and IgA expression, but found no significant effects (data not shown). In summary, these results suggest that SUMO and Ubc9 proteins do not affect on TGF-β1-induced, Smad3/4-mediated GLα transcription and IgA secretion by B cells.
Smad4 sumoylation by the PIASy redistributes Smad4 to subnuclear speckles, which result in enhanced Smad4-mediated transcriptional activation by TGF-β (15). In contrast, PIASy inhibits TGF-β/Smad3-mediated transcriptional response either by stimulating sumoylation of Smad3 and nuclear export of Smad3 (18), or by interacting with Smad3/4 and HDAC1 (19). Here we first tested the effect of PIASy on GLα promoter activity in B cells. Overexpression of PIASy diminished TGF-β1-induced GLα promoter activity in a dose-dependent manner (Fig. 3A, left panel). Overexpression of the Flag-PIASy protein was detected by immunoblot using an anti-Flag antibody (data not shown). In addition, PIASy suppressed TGF-β1-induced, Smad3/4-mediated GLα promoter activity (Fig. 3A, right panel). Thus, PIASy act as a negative regulator of Smad3/4-mediated GLα transcription by TGF-β1 in B cells. Next, we blocked HDAC with the effective inhibitor TSA to determine if the deacetylase is involved in the suppression of the GLα promoter activity. As shown in Fig. 3B (upper panel), TSA dose-dependently increased TGF-β1-induced GLα promoter activity and restored PIASy-mediated suppression of GLα activity. Conversely, PIASy inhibited TSA-mediated fortification of GLα promoter activity. Nevertheless, TSA could still enhance GLα promoter activity even when PIASy was overexpressed, albeit to a lesser degree (Fig. 3B, upper panel). This result suggests that although both PIASy and HDAC can suppress GLα transcription, they can act independently from each other. Namely, PIASy and HDAC may have their own inhibitory mechanisms that do not rely on each other rather than interrelated common mechanism. In addition, overexpression of HDAC1 further decreased PIASy-mediated suppression of GLα promoter activity (Fig. 3B, lower left panel). Overexpression of the HDAC1-GFP protein was measured by flow cytometric detection of GFP (data not shown). Furthermore, TSA recovered suppression of GLα promoter activity by PIASy in the presence of TGF-β1 (Fig. 3B, lower right panel). Thus, PIASy can cooperate with HDAC1 to suppress TGF-β1-induced, Smad3/4-mediated GLα transcription in mouse B cells. These suppressions by PIASy and HDAC1 may be due to 1) repression of GLα transcriptional activity by PIASy-mediated SUMO modification of certain proteins including Smad3/4, since SUMO modification often represses transcriptional activity of a number of transcription factors, such as Sp3, c-Jun, c-Myb, AP2, Elk-1, and p300 (29), and 2) recruitment of transcriptional repressor HDAC1 into the Smad3/4-PIASy complex, because PIASy can suppress Smadmediated transcriptional responses by interacting with Smads and HDAC (19). However, these possibilities remain to be tested. In addition, we are currently exploring the effects of PIASy and HDAC1 on the endogenous expression of GLα transcripts and IgA expression induced by TGF-β1/Smad3/4 in B cells.
Overall, our novel findings provide new basic evidence that SUMO and Ubc9 are unlikely to affect TGF-β/Smad3/4-mediated GLα transcription by B cells, while PIASy and HDAC1 act as repressor molecules in the transcription.
Figures and Tables
Figure 1
Effects of SUMO and Ubc9 on TGF-β1-induced, Smad3/4-mediated GLα promoter activity. (A) CH12F3-2A cells were transfected with GLα promoter reporter construct (pGL3-GLα [-448/+72], 10µg) and the indicated expression plasmids (5µg each). TGF-β1 (0.5 ng/ml) was added, and luciferase activity was determined 16 h later. Transfection efficiency was normalized to β-gal activities. Data shown are average luciferase activities of three independent transfections with SEM (bars) (left panel). Sumoylated proteins were detected by immunoblotting analysis for HA from extracts of CH12F3-2A cells transfected with SUMO-1 and SUMO-3 (right panel). (B) CH12F3-2A cells were transfected with pGL3-GLα [-448/+72] (10µg) and the indicated expression plasmids (5µg each) including Ubc9 (5µg). Induction and analysis were performed as shown in (A). *p<0.05, **p<0.01.
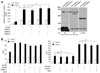
Figure 2
SUMO-1 is not involved in TGF-β/Smad3-induced GLα transcription and IgA isotype switching. CH12F3-2A cells were transfected with Smad3 (5µg) and SUMO-1 (5µg), and the cells were stimulated with TGF-β1 (0.5 ng/ml). (A) After 36 h of culture, RNA was isolated, and levels of GLTα and β-actin mRNA were measured by RT-PCR. (B) Following 3 days incubation, cells were stained with FITC-labeled anti-mouse IgA and PE-labeled anti-mouse IgM and analyzed by flow cytometry. (C) Culture supernatants were harvested after incubation for 4 days, and IgA levels were determined by ELISA. Data are means±SEM of three cultures.
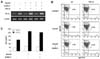
Figure 3
Effects of PIASy and HDAC1 on TGF-β1-induced, Smad3/4-mediated GLα promoter activity. (A) CH12F3-2A cells were transfected with pGL3-GLα [-448/+72] (10µg) and the indicated doses of PIASy alone (left panel) or PIASy (30µg) and Smad3/4 (5µg each) (right panel). Induction and analysis were performed as shown in Fig. 1A. (B) CH12F3-2A cells were transfected with pGL3-GLα [-448/+72] (10µg) and empty vector (pcDNA3, 30µg) or PIASy (30µg). TGF-β1 (0.5 ng/ml) and the indicated doses of TSA were added (upper panel). PIASy (30µg) and HDAC1 (15µg) were transfected along with the GLα promoter reporter (10µg) into CH12F3-2A cells, and cells were treated with TGF-β1 (lower left panel). The GLα promoter reporter (10µg), Smad3/4 (5µg each), and PIASy (30µg) were transfected into CH12F3-2A cells, and TGF-β1 (0.5 ng/ml) and TSA (5 ng/ml) were added (lower right panel). Luciferase activity was determined 16 h later. *p<0.05, **p<0.01.
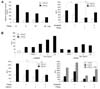
ACKNOWLEDGEMENTS
This research was supported by Basic Science Research Program through the National Research Foundation of Korea (NRF) funded by the Ministry of Education, Science and Technology (MEST) (NRF-2010-0025648).
References
2. Stavnezer J. Molecular processes that regulate class switching. Curr Top Microbiol Immunol. 2000; 245:127–168.


3. Park SR. Activation-induced cytidine deaminase in B cell immunity and cancers. Immune Netw. 2012; 12:230–239.


4. Park SR, Lee JH, Kim PH. Smad3 and Smad4 mediate transforming growth factor-beta1-induced IgA expression in murine B lymphocytes. Eur J Immunol. 2001; 31:1706–1715.


5. Park SR, Lee EK, Kim BC, Kim PH. p300 cooperates with Smad3/4 and Runx3 in TGFbeta1-induced IgA isotype expression. Eur J Immunol. 2003; 33:3386–3392.


6. Choi SH, Seo GY, Nam EH, Jeon SH, Kim HA, Park JB, Kim PH. Opposing effects of Arkadia and Smurf on TGFbeta1-induced IgA isotype expression. Mol Cells. 2007; 24:283–287.
7. Park KH, Nam EH, Seo GY, Seo SR, Kim PH. Tiul1 and TGIF are involved in downregulation of TGFbeta1-induced IgA isotype expression. Immune Netw. 2009; 9:248–254.


8. Park SR, Jung MH, Jeon SH, Park MH, Park KH, Lee MR, Kim PH. IFN-gamma down-regulates TGF-beta1-induced IgA expression through Stat1 and p300 signaling. Mol Cells. 2010; 29:57–62.


9. Miyazono K, Kamiya Y, Miyazawa K. SUMO amplifies TGF-beta signalling. Nat Cell Biol. 2008; 10:635–637.
11. Welchman RL, Gordon C, Mayer RJ. Ubiquitin and ubiquitin-like proteins as multifunctional signals. Nat Rev Mol Cell Biol. 2005; 6:599–609.


12. Ohshima T, Shimotohno K. Transforming growth factor-beta-mediated signaling via the p38 MAP kinase pathway activates Smad-dependent transcription through SUMO-1 modification of Smad4. J Biol Chem. 2003; 278:50833–50842.


13. Lin X, Liang M, Liang YY, Brunicardi FC, Feng XH. SUMO-1/Ubc9 promotes nuclear accumulation and metabolic stability of tumor suppressor Smad4. J Biol Chem. 2003; 278:31043–31048.


14. Lin X, Liang M, Liang YY, Brunicardi FC, Melchior F, Feng XH. Activation of transforming growth factor-beta signaling by SUMO-1 modification of tumor suppressor Smad4/DPC4. J Biol Chem. 2003; 278:18714–18719.


15. Lee PS, Chang C, Liu D, Derynck R. Sumoylation of Smad4, the common Smad mediator of transforming growth factor-beta family signaling. J Biol Chem. 2003; 278:27853–27863.


16. Liang M, Melchior F, Feng XH, Lin X. Regulation of Smad4 sumoylation and transforming growth factor-beta signaling by protein inhibitor of activated STAT1. J Biol Chem. 2004; 279:22857–22865.


17. Imoto S, Sugiyama K, Muromoto R, Sato N, Yamamoto T, Matsuda T. Regulation of transforming growth factor-beta signaling by protein inhibitor of activated STAT, PIASy through Smad3. J Biol Chem. 2003; 278:34253–34258.


18. Imoto S, Ohbayashi N, Ikeda O, Kamitani S, Muromoto R, Sekine Y, Matsuda T. Sumoylation of Smad3 stimulates its nuclear export during PIASy-mediated suppression of TGF-beta signaling. Biochem Biophys Res Commun. 2008; 370:359–365.


19. Long J, Matsuura I, He D, Wang G, Shuai K, Liu F. Repression of Smad transcriptional activity by PIASy, an inhibitor of activated STAT. Proc Natl Acad Sci U S A. 2003; 100:9791–9796.


20. Long J, Wang G, Matsuura I, He D, Liu F. Activation of Smad transcriptional activity by protein inhibitor of activated STAT3 (PIAS3). Proc Natl Acad Sci U S A. 2004; 101:99–104.


21. Kang JS, Saunier EF, Akhurst RJ, Derynck R. The type I TGF-beta receptor is covalently modified and regulated by sumoylation. Nat Cell Biol. 2008; 10:654–664.


22. Nakamura M, Kondo S, Sugai M, Nazarea M, Imamura S, Honjo T. High frequency class switching of an IgM+ B lymphoma clone CH12F3 to IgA+ cells. Int Immunol. 1996; 8:193–201.


23. Zhang Y, Feng X, We R, Derynck R. Receptor-associated Mad homologues synergize as effectors of the TGF-beta response. Nature. 1996; 383:168–172.


24. Hahn SA, Schutte M, Hoque AT, Moskaluk CA, da Costa LT, Rozenblum E, Weinstein CL, Fischer A, Yeo CJ, Hruban RH, Kern SE. DPC4, a candidate tumor suppressor gene at human chromosome 18q21.1. Science. 1996; 271:350–353.


25. Long J, Wang G, He D, Liu F. Repression of Smad4 transcriptional activity by SUMO modification. Biochem J. 2004; 379:23–29.


26. Tou L, Liu Q, Shivdasani RA. Regulation of mammalian epithelial differentiation and intestine development by class I histone deacetylases. Mol Cell Biol. 2004; 24:3132–3139.


27. Park MH, Park SR, Park MR, Kim YH, Kim PH. Retinoic acid induces expression of Ig germ line α transcript, an IgA isotype switching indicative, through retinoic acid receptor. Genes Genomics. 2011; 33:83–88.


28. Jang YS, Choi SH, Park SR, Kim HA, Park JB, Kim PH. Characterization of mouse B lymphoma cells (CH12F3-2A) for the study of IgA isotype switching. Immune Netw. 2004; 4:216–223.


29. Verger A, Perdomo J, Crossley M. Modification with SUMO. A role in transcriptional regulation. EMBO Rep. 2003; 4:137–142.