Abstract
Mycobacterium scrofulaceum is an environmental and slow-growing atypical mycobacterium. Emerging evidence suggests that M. scrofulaceum infection is associated with cervical lymphadenitis in children and pulmonary or systemic infections in immunocompromised adults. However, the nature of host innate immune responses to M. scrofulaceum remains unclear. In this study, we examined the innate immune responses in murine bone marrow-derived macrophages (BMDMs) infected with different M. scrofulaceum strains including ATCC type strains and two clinically isolated strains (rough and smooth types). All three strains resulted in the production of proinflammatory cytokines in BMDMs mediated through toll-like receptor-2 and the adaptor MyD88. Activation of MAPKs (extracellular signal-regulated kinase 1/2, and p38, and c-Jun N-terminal kinase) and nuclear receptor (NF)-κB together with intracellular reactive oxygen species generation were required for the expression of proinflammatory cytokines in BMDMs. In addition, the rough morphotypes of M. scrofulaceum clinical strains induced higher levels of proinflammatory cytokines, MAPK and NF-κB activation, and ROS production than other strains. When mice were infected with different M. scrofulaceum strains, those infected with the rough strain showed the greatest hepatosplenomegaly, granulomatous lesions, and immune cell infiltration in the lungs. Notably, the bacterial load was higher in mice infected with rough colonies than in mice infected with ATCC or smooth strains. Collectively, these data indicate that rough M. scrofulaceum induces higher inflammatory responses and virulence than ATCC or smooth strains.
Mycobacterium scrofulaceum is a slow-growing environmental and opportunistic atypical mycobacterium that causes cervical lymphadenopathy in children and disseminated infection in adult patients with immunodeficiency (1). In addition, an extensively disseminated M. scrofulaceum infection was reported in an immunocompetent host with miliary lung lesions, lymphadenitis, granulomatous hepatitis, osteomyelitis, and subcutaneous abscesses (2). In Korea, M. scrofulaceum causes one of the most prevalent nontuberculous mycobacterial (NTM) pulmonary diseases, often found in immunocompetent patients (3). M. scrofulaceum is often classified as an MAIS organism (with M. avium and M. intracellulare). MAIS organisms are isolated from all parts of the environmental, especially drinking water and municipal water systems. Because MAIS organisms are generally resistant to most antimicrobial drugs, surgical excision often is used for treatment of lymphadenitis associated with atypical mycobacterial infection (4,5). However, the nature of the host immune response and pathogenic mechanisms that control the immune system in M. scrofulaceum infection remain unclear.
Growing evidence suggests that the innate immune system is an essential sentinel to protect the host as a primary and immediate defense during mycobacterial infection (6). Toll-like receptor is one of the most characteristic innate immune receptors and plays a crucial role in the initiation of inflammatory cytokine production and intracellular signaling to induce nuclear factor-κB activation in various cells infected with diverse mycobacterial strains (7,8,9). The activation of innate immune signaling induced by mycobacteria or their distinct components was shown to result in the production of proinflammatory cytokines and antimicrobial proteins in macrophages, promoting early control of mycobacterial infection (7,10). Triggering of innate immune receptors by mycobacterial ligands or mycobacteria leads to an activation of the mitogen-activated protein kinase (MAPK) pathway through TGF-β-activated kinase 1 (TAK1)-TAK1-binding protein (TAB) complex activation (11,12). In addition, the induction and activation of innate immune responses are crucial in shaping adaptive immune responses (13). In addition, mycobacterial infection triggers oxidative stress to increase the generation of intracellular reactive oxygen species, playing essential roles in signaling to mediate a variety of physiological functions in addition to pathogen killing in phagocytes (14,15). However, M. scrofulaceum-induced host innate immune responses and their regulatory mechanisms have not been fully elucidated, compared to those induced by M. tuberculosis or other NTM infection.
In this study, we examined not only the intracellular signaling pathways induced by M. scrofulaceum infection and underlying molecular mechanism, but also essential role of M. scrofulaceum morphotypes for regulating innate immune responses using ATCC type strains and clinically isolated two strains.
Wild-type 6-to-8-week-old female C57BL/6 mice were purchased form SAMTAKO BIO KOREA (Gyeonggi-do, Korea) and TLR2-, MyD88-, and TRIF-knockout (KO) mice on a C57BL/6 background were kindly provided by Dr. S. Akira (Osaka University, Japan). All animal procedures were approved by the Institutional Animal Care and Use Committee of Chungnam National University. Primary BMDMs were isolated from femurs of mice and then differentiated for 5~7 days in DMEM (Life Technologies BRL) containing macrophage colony stimulating factor (M-CSF, 25 ng/ml) (R&D systems, Minneapolis, MN, USA), 4 mM glutamine, and 10% FBS, as described previously (16).
All reagents and chemicals were purchased from Sigma-Aldrich except those noted below, which were obtained from the indicated suppliers. Specific Abs against phospho-ERK1/2 (Thr202/Tyr204), phospho-p38 (Thr180/Tyr182), and phospho-JNK (Thr183/Tyr185) were purchased from Cell signaling Technology (Cell signaling, Beverly, MA, USA). Specific inhibitors of MAPKs [U0126 (MEK inhibitor), SB203580 (p38 inhibitor), and SP600125 (JNK inhibitor)], the antioxidant N-acetyl-L-cysteine (NAC), diphenyleneiodonium (DPI) as an inhibitor of NADPH oxidase, BAY11-7082 (Bay), and caffeic acid phenethyl ester (CAPE) were purchased from Calbiochem (San Diego, CA, USA). Anti-β-actin (I-19) was purchased from Santa Cruz Biotechnology (Santa Cruz, CA, USA). Dimethyl sulfoxide (DMSO; Sigma-Aldrich, St. Louis, MO, USA) was added to cultures at a concentration of 0.1% (v/v) as a solvent control.
M. scrofulaceum ATCC and clinical strains (KMRC 200-30113, -30114, -30115, -30116) were obtained from the Korean Institute of Tuberculosis (Osong, Chungbuk, Korea), maintained in Ogawa medium, and inoculated in Middlebrook 7H9 broth (Difco Laboratories, Detroit, MI, USA) supplemented with 10% oleic acid-albumin-dextrose-catalase (OADC) (BD Pharmingen, San Diego, CA, USA), 0.5% glycerol, and 0.05% Tween 80 (Sigma-Aldrich) at 37℃. Inocula were prepared from the mid-exponential-growth phase cultures. Bacteria were washed four times with cold PBS and then concentrated or diluted as needed. The number of colony-forming units (CFU) per milliliter was determined by plating on 7H10 agar supplemented with OADC at 37℃. For the in vitro experiments, macrophage infections were performed as described previously (16). Briefly, cells were infected with mycobacteria at different MOIs and incubated for 4 h at 37℃ in a 5% CO2 atmosphere. After allowing time for phagocytosis, cells were washed four times with fresh PBS to remove extracellular bacteria and then incubated with complete DMEM without antibiotics. As controls, cultures of uninfected macrophages were maintained under the same conditions. For the in vivo experiments, a bacterial inoculum was prepared by thawing a frozen aliquot of a mycobacterium. Mice were intravenously injected with a suspension of 5×107 or 5×108 CFU bacteria per mouse in a final volume of 100µl. Mice were euthanized at 0, 3, 7, 15, and 30 days after infection and the bacterial loads in the infected organs were determined by plating serial 10-fold dilutions of tissue homogenates on Middlebrook 7H10 medium supplemented with OADC. The plates were incubated for 4~6 weeks at 37℃. Control mice were injected with sterile saline.
Colony morphology was evaluated on 7H10 agar plates by microscopic observation. For detection of the cord formation, bacteria were cultured in 7H9 under stationary phase conditioned broth at 37℃ for 7 days. Culture suspensions were spun onto glass slides using a cytocentrifuge and the bacteria were heat-fixed and then stained with crystal violet dye. Bacteria were examined at ×40 or ×100 magnification using a light microscope (Olympus, Japan) and photographed.
BMDMs or mice were infected as indicated in the figures and processed for analysis by RT-PCR, Western blot, and sandwich ELISA as described previously (16). Briefly, total RNA was isolated from BMDMs or lungs of mice using TRIzol reagent (Invitrogen, Carlsbad, CA) according to the manufacturer's instructions. Then, semi-quantitative reverse transcription polymerase chain reaction (RT-PCR) and real-time quantitative PCR were performed. The sequences of the primers used are as follows: TNF-α (forward) 5'-CGGACTCCGCAAAGTCTAAG-3', (reverse) 5'-ACGGCATGGATCTCAAAGAC-3', IL-6 (forward) 5'-GGAAATTGGGGTAGGAAGGA-3', (reverse) 5'-CCGGAGAGGAGACTTCACAG-3', IL-12p40 (forward) 5'-GGA AGCACGGCAGCAGAATA-3', (reverse) 5'-AACTTGAGGGAGAAGTAGGAATGG-3', β-actin (forward) 5'-TCATGAAGTGTGACGTTGACATCCGT-3', (reverse) 5'-CCTAGAAGCATTTGCGGTGCACGATG-3'. PCR was performed as follows: For proinflammatory cytokine PCR, 30 cycles of denaturation at 95℃ for 1 min, annealing at 58℃ for 1 min, and extension at 72℃ for 1 min, with a final extension at 72℃ for 10 min. Real-time PCR was performed using SYBR Green PCR Master Mix (Molecular Probes, Carlsbad, CA, USA), and transcript levels were quantified using an ABI 7900 Sequence Detection System (Applied Biosystems). The mean value of triplicate reactions was normalized against the mean value for β-actin.
For the NF-κB luciferase assay, BMDMs were transduced with NF-κB p65 luciferase reporter adenovirus [20 plaque-forming units (PFU)/cell] constructs. After 36 h, the culture medium was changed to new medium and then cells were infected with MS strains (MOI=1, 5, 10). After 4 h, the cells were harvested in lysis buffer and luciferase activity was measured by a dual luciferase assay (Promega, Madison, WI, USA) as recommended by the manufacturer.
NF-κB p65 translocation was detected by immunofluorescence microscopy analysis as described previously (9). Briefly, BMDMs differentiated on coverslips were infected with MS strains at 37℃ for 6 h, fixed with 4% (w/v) paraformaldehyde in PBS for 10 min at RT, permeabilized with 0.25% (v/v) Triton X-100 in PBS for 15 min at RT, and then blocked with 3% bovine serum albumin (BSA) in PBS for 1 h. Cells were immunostained with rabbit anti-mouse NF-κB p65 (1:400) for 2 h and the staining was detected using Alexa Fluor 488-conjugated anti-rabbit immunoglobulin (Ig) G (1:400, Molecular Probes, Eugene, OR, USA) for 1 h at RT. Nuclei were stained by incubation with 1µg/ml 4',6'-diamidino-2-phenylindole (DAPI; Sigma-Aldrich). After mounting, fluorescence images were acquired with a confocal laser scanning microscope (DP ver. 1.2.108, Olympus).
For immunoblot analysis, primary Abs were diluted 1:1,000. Membranes were developed using a chemiluminescent reagent (ECL; Pharmacia-Amersham, Freiburg, Germany) (17). In sandwich ELISA, the levels of TNF-α, IL-6, and IL-12p40 in cell culture supernatants were analyzed using DuoSet antibody pairs (BD Pharmingen), according to the manufacturer's protocol.
ROS production was measured by DHE assay, as previously described (8). Briefly, BMDMs were infected with MS strains (MOI=5, 10) for 30 min and washed three times with phosphate-buffered saline (PBS) to remove extracellular bacteria. The cells were incubated with 2µM DHE dye to detect superoxide for 30 min at 37℃ in 5% CO2 and then fixed with 4% paraformaldehyde in PBS at room temperature (RT) followed by flow cytometry using a FACSCanto II flow cytometer (Becton Dickinson, San Jose, CA, USA) and FlowJo software (Tree Star, Ashland, OR, USA).
Mice were sacrificed at 0, 3, 7, 15, and 30 days after infection with MS strains and lung tissue was collected from individual mice. Lung tissues were fixed in 10% formalin and then dehydrated, paraffin-embedded, and sectioned at 5µm. Sections of lung tissues were stained with haematoxylin and eosin for histopathological analysis. Morphometric image analysis was performed at ×100 or ×200 magnification. For immunohistochemical staining of COX-2, paraffin-embedded lung sections were stained using rabbit anti-mouse polyclonal COX-2 antibody (Abcam, Cambridge, UK), biotin-conjugated rabbit anti-mouse IgG, and a peroxidase-conjugated streptavidin antibody. Then, visualization was performed with 3,3-diaminobenzidine as the peroxidase substrate (Sigma-Aldrich).
The data were obtained as the mean (±standard deviation) value from independent experiments on 3~5 mice per group. The statistical analyses were performed using Student's t-test with Bonferroni correction or analysis of variance in addition to two-way ANOVA using GraphPad Prism version 5 (GraphPad software Inc, San Diego, CA, USA). p<0.05 was considered significant.
Previous studies demonstrated that the morphotype of mycobacteria is associated with virulence (18,19). To identify the correlation between morphotype and host immune responses of M. scrofulaceum, we prepared various strains of M. scrofulaceum including ATCC 19991 (ATCC), clinically isolated KMRC 00200-30113 (113S), MS KMRC 00200-30114 (114R), KMRC 00200-30115 (115S), and KMRC 00200-30116 (116S). Microscopic images indicated that only colonies of the 114R strain had a rough morphology similar to that of the M. tuberculosis virulent strain (H37RV) (Fig. 1A). We next examined whether the colony morphology affects the production of proinflammatory cytokines such as tumor necrosis factor (TNF)-α, interleukin (IL)-6, and IL-12p40. We infected bone marrow-derived macrophages (BMDMs) with the ATCC, 113S, or 114R strains of M. scrofulaceum and examined the mRNA and protein expression of proinflammatory cytokines. As shown in Fig. 1B, the cells infected with 114R showed higher mRNA expression of TNF, IL-6, and IL-12p40 than those infected with ATCC or 113S at both 3 h and 6 h of infection. Consistent with these findings, protein expression of proinflammatory cytokines was highly increased in cells infected with 114R compared with those infected with ATCC, 113S, 115S, 116S in concentration- and time-dependent manners (Fig. 1C). These findings indicate that M. scrofulaceum-mediated proinflammatory responses depend on colony morphotype.
Numerous studies have reported that mycobacteria and their antigenic components activate intracellular signaling cascade through innate recognition of TLRs (9,12,16,17,20). To determine the roles of TLR2 in mycobacteria-induced production of proinflammatory cytokines in macrophages, BMDMs from TLR2 wild type (WT) and knockout (KO) mice were stimulated with ATCC, 113S, or 114R strains of M. scrofulaceum in an MOI-dependent manner. As shown in Fig. 2A, the mycobacteria-induced production of TNF, IL-6, and IL-12p40 was absolutely inhibited in BMDMs from TLR2 KO mice. Because the adaptor proteins MyD88 and TRIF are essential for signal transduction of TLR (21), we further examined whether the production of these three strain-induced cytokines was dependent on either MyD88 or TRIF. BMDMs from MyD88 KO mice but not from TRIF KO mice showed a significant decrease of TNF, IL-6, and IL-12p40 levels after infection with each of the strains (Fig. 2B). These results suggest that the TLR2/MyD88 signaling pathway is important for host inflammatory responses in M. scrofulaceum infection.
As a consequence of cross-talk between pattern-recognition receptors (PRRs) and mycobacteria, inflammatory responses are mainly initiated by a conserved signaling pathway involving NF-κB activation (12). We therefore examined NF-κB luciferase activity and nuclear translocation in BMDMs infected with the ATCC, 113S, or 114R strains of M. scrofulaceum. As shown in Fig. 3A, 114R-infected cells showed higher NF-κB luciferase activity than cells infected with ATCC or 113S in a dose-dependent manner. NF-κB nuclear translocation is followed by phosphorylation of the IκB kinase (IKK) complex and the inhibitor of NF-κB proteins (IκB)-α, which is critical for the transcriptional activation of target genes including proinflammatory cytokines (22). We found that 114R infection resulted in a greater enhancement of nuclear translocation of p65 (Fig. 3B) than ATCC or 113S infection. In addition, pretreatment with NF-κB specific inhibitors including Bay 11-7085 (BAY) and caffeic acid phenethyl ester (CAPE) significantly inhibited the mRNA expression of 114R-induced proinflammatory cytokines such as TNF-α and IL-6 (Fig. 3C). These results suggest that 114R activated NF-κB signaling more strongly than ATCC or 113S to produce proinflammatory cytokines.
We previously found that mycobacterial infection induced phosphorylation of MAPK such as p38, JNK, and ERK1/2, resulting in production of inflammatory cytokines (16,20). To determine whether M. scrofulaceum-induced inflammatory responses were dependent on MAPK signaling, we monitored MAPK activation in macrophages. Although these three strains induced phosphorylation of p38, SAPK/JNK and ERK1/2, the 114R strain especially induced rapid and strong activation within 30 min (Fig. 4A and B). We next investigated the role of MAPK signaling in proinflammatory cytokine production induced by the 114R strain. As shown in Fig. 4C, pretreatment with specific inhibitors of JNK (SP600125), p38 (SB203580), or MEK (U0126) caused significant inhibition of 114R-induced TNF-α, IL-6, and IL-12p40 production in a concentration-dependent manner (Fig. 4C). Together, these results suggest that the 114R strain strongly induces the production of proinflammatory cytokines through enhanced activation of MAPK signaling compared to ATCC or 113S in macrophages.
Previous studies proposed that Gp91phox/NADPH oxidase (NOX) 2 is critical for regulation of TLR-mediated inflammatory responses and host innate immune responses against M. tuberculosis (15) and M. massiliense (20). We first investigated whether M. scrofulaceum induced intracellular superoxide production in BMDMs using the oxidative fluorescent dye dihydroethidium (DHE). Flow cytometric analysis (Fig. 5A) showed that 114R infection, but not that of ATCC or 113S, strongly induced superoxide generation at MOI 5 and 10. We further examined whether 114R-induced ROS generation was essential for the production of proinflammatory cytokines in macrophages infected with the three strains. As shown in Fig. 5B, pretreatment with the general ROS scavenger NAC and NADPH oxidase inhibitor DPI significantly attenuated the production of TNF-α in a concentration-dependent manner. These data suggest that 114R activated ROS generation more strongly than ATCC or 113S, which resulted in hyperactivation of inflammatory responses.
Mycobacterial infection is known as a major source of granulomatous reactions in humans and in animal models (23). We first investigated whether infection by M. scrofulaceum ATCC, 113S, and 114R strains induced granuloma formation in a murine model. At day 7, 114R-infected mice showed a stronger granulomatous response and infiltration of immune cells (Fig. 6A) than ATCC- or 113S-infected mice. In comparison to animals infected with the ATCC or 113S strain, 114R-infected mice showed significantly higher bacterial loads at day 7 post infection (Fig. 6D).
During M. tuberculosis infection, inflammatory responses are closely associated with granulomatous reactions (24). We thus examined the inflammatory responses in mice infected with the three strains. As shown in Fig. 6C, expression of cyclooxygenase-2, a key enzyme for activation of the inflammatory cascade, was significantly higher in the lungs of 114R-infected mice than in those of ATCC- or 113S-infected mice. We further analyzed the mRNA expression of proinflammatory cytokines in mouse lungs infected with the three strains. mRNA expression of TNF-α, IL-6, and IL-12p40 was higher in the lungs of 114R-infected mice than in those of ATCC or 113S-infected mice (Fig. 6B). Together, these data indicate that 114R infection induces strong inflammatory responses that finally result in enhanced granulomatous reactions.
M. scrofulaceum belonging to the MAIS organisms (with M. avium and M. intracellulare) (4) is associated with non-tuberculous mycobacterial (NTM) lymphadenitis and pulmonary disseminated infection in adult patients with or without immunodeficiency (1,2,3,25,26,27). M. scrofulaceum is an environmental mycobacteria isolated from soil and water in tropical regions (28) and also found in cooling towers that are suitable environments for potentially pathogenic mycobacteria (29).
M. scrofulaceum infection is relatively rare compared to infection by other MAIS organisms but appears to have clinical relevance associated with HIV infection in humans (1,30). In animal infection models, prior M. scrofulaceum infection (with two other mycobacterial strains, such as M. avium and M. vaccae) led to significant inhibition of BCG multiplication and reduced vaccination effects in sensitized mice (31). Thus, it is considered that priming immune host responses with environmental mycobacteria could reduce the protective effects of BCG infection or vaccination to a level insufficient to control M. tuberculosis infection (31,32). Despite these findings, the inducing activities and regulatory mechanisms of the slow-growing NTM M. scrofulaceum with respect to the host innate and inflammatory immune responses have been poorly characterized, compared to those of M. tuberculosis or rapidly growing NTMs. In the present study, we found that R isolates formed multicellular cords, similar to those observed in M. tuberculosis, but S isolates did not. We then subjected each morphotype to further immune studies. We found that M. scrofulaceum-infected macrophages showed a robust induction of proinflammatory cytokine expression, and the R morphotype of M. scrofulaceum clinical isolates induced higher cytokine production than type strains and the S morphotype.
Although previous studies described the R morphology of a M. avium-M. intracellulare-M. scrofulaceum complex lacking outer cell wall C-mycoside glycopeptidolipids (GPLs) (33), the present data on increased induction of inflammatory responses by the M. scrofulaceum R morphotype partially supports the findings of previous studies in other NTM strains showing the induction of enhanced immune and inflammatory responses by R type NTM strains. In certain NTM strains, the S morphotypes usually contain GPLs that are found in the outermost part of the cell envelope and also play a key role in biofilm formation (18,34). The R type that lacks GPLs can multiply in host macrophages and cause persistent infection although it cannot form biofilms (18). Recently, it was shown that the massive production of serpentine cords by the R variant of M. abscessus contributes to resistance against phagocytosis in macrophages or neutrophils and induces abscess formation and increased virulence in zebrafish models (35). In addition, the R type of M. abscessus activates innate immune responses in macrophages through TLR2, suggesting that GPLs on NTM cell walls mask the underlying mycobacterial lipids, thereby promoting innate immune responses and airway inflammation (36). Recent studies also showed that human monocytes were not able to internalize the R type of M. abscessus and induced higher IL-1β production and lower IL-10 production than the S type (37). Taken together, the current observations that the R type of M. scrofulaceum induced increased proinflammatory responses in macrophages are consistent with previous findings for other NTM strains, especially M. abscessus.
TLR2 and MyD88 are required for early proinflammatory cytokine generation in the macrophage response against M. scrofulaceum infection. Our data indicate a dependence on NF-κB to also drive inflammatory responses against M. scrofulaceum. Previous studies by us and others showed that TLR2-dependent signaling is important for NF-κB signaling and proinflammatory cytokine generation in macrophages in response to diverse mycobacterial strains including M. tuberculosis, M. avium, M. abscessus, and M. massilience (7,8,9,20,38,39). In addition, mycobacterial components, including lipomannans from M. chelonae and M. kansasii were shown to induce proinflammatory cytokine generation through TLR2 (40). M. tuberculosis was shown to recognize TLR2, TLR4, and TLR9 in initiating innate immune responses (38,41). The TLR adaptor MyD88 plays a key role in the activation of innate immune responses to mycobacterial infection. Based on these findings, MyD88-deficient mice have increased susceptibility for acute M. tuberculosis infection (42). In addition, MyD88-deficient macrophages and dendritic cells showed defective cytokine and nitric oxide production in response to mycobacterial infection (42). The current data demonstrate that TLR2-MyD88-NF-κB signaling is mainly required for M. scrofulaceum-induced innate immune responses in macrophages. TLR2-induced host immune responses are essential for the suppression of mycobacterial proliferation within macrophages but also function in mycobacterial escape through decreased MHC-II expression or antigen-processing ability (43).
The MAPK signaling pathway has been described to be involved in the generation of proinflammatory cytokines such as TNF-α, IL-6, and IL-12p40 during mycobacterial infection (7,44). TLR-induced MAPK signaling is required for the initiation of effective innate immune responses against mycobacterial components and M. tuberculosis (7,44,45). Our previous studies showed that MAPK activation in response to the mycobacterial 38-kDa antigen was highly increased in human monocytes from active pulmonary tuberculosis patients (46). Recently, it was shown that MAPK activation and pro- and anti-inflammatory cytokine secretion were significantly depressed in monocytes from patients with M. abscessus pulmonary diseases (47). Therefore, the analysis of the activation of the MAPK signaling pathway may contribute to an explanation of M. tuberculosis host susceptibility and atypical mycobacterial infection.
Phagocytized bacterial infection by host innate immune cells including mycobacteria initiates an oxidative burst reaction (48). Generation of free radicals derived from phagocytes infected with human pathogens contributes to both effective defense and immune escape during microbial infection (49,50). Moreover, intracellular ROS are critical signaling mediators in immune cells for various physiological functions including TLR signaling in response to various types of microbial ligand stimulation (15). Our support previous findings that ROS signaling is required for inflammatory cytokine generation in macrophages infected with M. massiliense (20). Previous studies found that ROS generation is required for the ER stress-induced apoptosis in macrophages infected with clinical isolates of M. kansasii (51). Our earlier studies also showed that antimicrobial activity during anti-mycobacterial chemotherapy is dependent on cellular and mitochondrial ROS-mediated autophagy activation (52). In addition, oxidative stress induced by vitamin C has been found to be crucial for bactericidal activity against M. tuberculosis (53). Indeed, different M. tuberculosis strains have been shown to exhibit diverse patterns of ROS production (54). Because multidrug-resistant strains lack the ability to induce neutrophil apoptosis and ROS generation (54), it is tempting to speculate that the different ability to induce ROS generation among clinical strains is associated with disease pathology or human susceptibility to atypical mycobacterial infection.
Our in vivo data showed that the R morphotype of M. scrofulaceum induced increased pathology, granulomatous lesions, and immune cell infiltration in lungs, compared to the S morphotype or ATCC strain. In addition, the bacterial loads were higher in mice infected with the R morphotype than in those infected with other strains. Among different M. avium complex strains, smooth transparent variants, the most frequent types found in AIDS patients, showed increased virulency and mycobacterial multiplication in the lungs, livers, and spleens of mice than strains of other morphotypes (55). Furthermore, mouse studies using different strains of M. abscessus showed that differences among strains were highly associated with differences in virulence and distinct types of clinical disease including the upper lobe fibrocavitary form and nodular bronchiectatic form (56). Future studies should clarify whether the differences in pathology and virulence among diverse clinical strains is associated with the human host susceptibility to M. scrofulaceum. Together, our data indicate that M. scrofulaceum activates innate immune responses through the TLR2-MyD88-NF-κB, MAPK, and ROS signaling pathways. Among different strains, the R morphotype showed the greatest induction of inflammatory responses and intracellular signaling activation. We further showed that the in vitro immune profile was highly correlated with the in vivo manifestation of infection by different strains. Characterization of immune signaling features may contribute to understanding the host-pathogen interaction for the development of novel therapeutics or preventives against M. scrofulaceum infection.
Figures and Tables
Figure 1
Mycobacterium scrofulaceum-114R elicits cord formation and enhanced expression of proinflammatory cytokines in BMDMs. (A) The colony type of M. scrofulaceum-ATCC, -113S, and -114R on 7H10 agar plates was characterized microscopically at ×40 (a) and ×100 magnification (b), and the cords formed in broth culture after 14 days of incubation were heat fixed and then stained with crystal violet (c). (B and C) BMDMs were infected with different clinical M. scrofulaceum strains (MOI=3) for the indicated times. The mRNA expression and production of TNF-α, IL-6, and IL-12p40 were evaluated by semi-quantitative RT-PCR (B) and ELISA (C), respectively. The relative expression levels were normalized against those of β-actin (B, bottom). The data show the means±SD from three independent experiments. U; uninfected control.
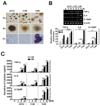
Figure 2
Mycobacterium scrofulaceum-induced proinflammatory cytokine production is mediated by TLR2/MyD88 signaling. (A and B) BMDMs isolated from WT and TLR2 KO mice (for A) or WT, MyD88, and TRIF KO mice (for B) were stimulated with M. scrofulaceum-ATCC, -113S, and -114R (MOI=1, 5, 10) for 18 h. The levels of TNF-α, IL-6, and IL-12p40 in the supernatants were measured by ELISA. Pam3CSK4 (P; 100 ng/ml) was used as positive ligand for TLR2. The data represent the mean±SD from three independent experiments. U; uninfected control.
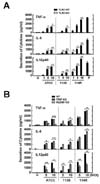
Figure 3
Mycobacterium scrofulaceum-114R strongly increases the production of proinflammatory cytokines through NF-κB signaling in BMDMs. (A) BMDMs were transduced with NF-κB p65 adenovirus luciferase construct (20 PFU/cells) for 36 h and then infected with M. scrofulaceum-ATCC, -113S, -114R (MOI=1, 5, 10) for 4 h. Cell lysates were harvested and luciferase activity was measured. (B) BMDMs were infected with M. scrofulaceum strains (MOI=5) for 30 min and then immunolabeled with anti-NF-κB p65 antibody and anti-rabbit-AlexaFluor 488 (green), and the nuclei were stained with DAPI (blue). Representative immunofluorescence images (left panel) and the average mean fluorescence intensity of cells exhibiting NF-κB nuclear translocation (right panel) are shown. (C) BMDMs were preincubated in the presence or absence of BAY11-7082 (BAY; 0.3, 1, 3µM) or CAPE (1, 5, 10µM) for 45 min prior to infection with M. scrofulaceum strains (MOI=5). The mRNA expression and production of TNF-α and IL-6 were evaluated by semi-quantitative RT-PCR. The data show the mean±SD from three independent experiments. U; uninfected control, SC; solvent control (0.1% DMSO).
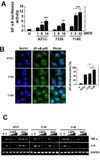
Figure 4
Mycobacterium scrofulaceum 114R-induced pro-inflammatory cytokine production is modulated through MAPK signaling in BMDMs. (A) Kinetics of phospho-RK1/2, -p38, and -SAPK/JNK in BMDMs infected with M. scrofulaceum-ATCC, -113S, and -114R (MOI=5). The cell lysates were collected at the indicated times and phosphorylated MAPKs were examined by western blot analysis. β-ctin was used as a loading control. (B) Expression levels were normalized against those of β-actin (C) BMDMs were pretreated with p38 inhibitor (SB203580; 1, 5, and 10µM), MEK-1 inhibitor (U0126; 5, 10, and 20µM), and JNK inhibitor (JNK; 5, 10, and 20µM) for 45 min prior to infection with different M. scrofulaceum strains (MOI=5). The culture supernatants were harvested at 18 h, and the production of TNF-α , IL-6, and IL-12p40 cytokines was measured by ELISA. The data show the mean±SD of three independent experiments. Significant differences: #p<0.05 compared with uninfected control; *p<0.05, **p<0.01, and ***p<0.001 compared with solvent control. U; uninfected control, SC; solvent control (0.1% DMSO).
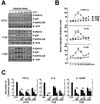
Figure 5
ROS signaling-mediated proinflammatory cytokine production in Mycobacterium scrofulaceum-infected BMDMs (A) BMDMs were infected with M. scrofulaceum-ATCC, -113S, or -114R (MOI 5, 10) for 30 min and then stained with DHE for 30 min. Superoxideroducing cells were analyzed by flow cytometry. (B) BMDMs were incubated with NAC (10, 20, and 30 mM) or DPI (1, 5, and 10µM) before infection with different M. scrofulaceum strains (MOI=5). The supernatant was harvested at 20 h, and cytokine production was measured by ELISA. The data show the mean±SD of three independent experiments. Significant differences: **p<0.01 and ***p<0.001 compared with solvent control. U; uninfected control, SC; solvent control (0.1% DMSO).
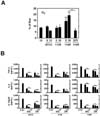
Figure 6
Mycobacterium scrofulaceum-114R infection induces granulomatous lesion formation, higher mycobacteria burden and inflammation in the lung C57BL/6 mice were infected intravenously with 5×107 CFU of M. scrofulaceum-ATCC, -113S, or 114R or treated with saline (UI) for the indicated periods of time. (A) Lung tissues were collected and fixed with 10% formalin and paraffin-embedded. Lung sections were stained with hematoxylin and eosin (H&E). Inflammatory cell infiltration, granulomatous lesions, and lung histology were observed at ×100 (upper) or ×200 (bottom) magnification; the black arrowhead in the panel indicates the granulomatous lesion. (B) mRNA expression of TNF-α, IL-6, and IL-12p40 cytokines in the lungs was determined by real-time PCR analysis. (C) Expression of COX-2 in lungs of mice infected with M. scrofulaceum strains was examined at 14 days post-infection by immunohistochemical staining of COX-2. (D) The mycobacterial loads of the lungs were determined at 0 and 7 days post-infection. The data show the mean±SD of three individual mice per group. Significant difference: *p<0.05, compared to M. scrofulaceum-ATCC or -113S-infected mice; **p<0.01 compared with the uninfected control (UI).
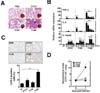
ACKNOWLEDGEMENTS
We thank Heekyung Yoo and Young Kil Park for providing clinical strains of M. scrofulaceum and for their discussion. This work was supported by the National Research Foundation of Korea (NRF) grant funded by the Korea government (MSIP) (2007-0054932) at Chungnam National University.
ACKNOWLEDGEMENTS
We thank Heekyung Yoo and Young Kil Park for providing clinical strains of M. scrofulaceum and for their discussion. This work was supported by the National Research Foundation of Korea (NRF) grant funded by the Korea government (MSIP) (2007-0054932) at Chungnam National University.
References
1. Sanders JW, Walsh AD, Snider RL, Sahn EE. Disseminated Mycobacterium scrofulaceum infection: a potentially treatable complication of AIDS. Clin Infect Dis. 1995; 20:549.


2. Hsueh PR, Hsiue TR, Jarn JJ, Ho SW, Hsieh WC. Disseminated infection due to Mycobacterium scrofulaceum in an immunocompetent host. Clin Infect Dis. 1996; 22:159–161.


3. Ryoo SW, Shin S, Shim MS, Park YS, Lew WJ, Park SN, Park YK, Kang S. Spread of nontuberculous mycobacteria from 1993 to 2006 in Koreans. J Clin Lab Anal. 2008; 22:415–420.


4. Falkinham JO III. Factors influencing the chlorine susceptibility of Mycobacterium avium, Mycobacterium intracellulare, and Mycobacterium scrofulaceum. Appl Environ Microbiol. 2003; 69:5685–5689.


6. Verrall AJ, Netea MG, Alisjahbana B, Hill PC, van CR. Early clearance of Mycobacterium tuberculosis: a new frontier in prevention. Immunology. 2014; 141:506–513.


7. Jo EK, Yang CS, Choi CH, Harding CV. Intracellular signalling cascades regulating innate immune responses to Mycobacteria: branching out from Toll-like receptors. Cell Microbiol. 2007; 9:1087–1098.


8. Shin DM, Yang CS, Yuk JM, Lee JY, Kim KH, Shin SJ, Takahara K, Lee SJ, Jo EK. Mycobacterium abscessus activates the macrophage innate immune response via a physical and functional interaction between TLR2 and dectin-1. Cell Microbiol. 2008; 10:1608–1621.


9. Lee HM, Shin DM, Choi DK, Lee ZW, Kim KH, Yuk JM, Kim CD, Lee JH, Jo EK. Innate immune responses to Mycobacterium ulcerans via toll-like receptors and dectin-1 in human keratinocytes. Cell Microbiol. 2009; 11:678–692.


10. Torrado E, Cooper AM. Cytokines in the balance of protection and pathology during mycobacterial infections. Adv Exp Med Biol. 2013; 783:121–140.


12. Basu J, Shin DM, Jo EK. Mycobacterial signaling through toll-like receptors. Front Cell Infect Microbiol. 2012; 2:145.


13. Quigley M, Martinez J, Huang X, Yang Y. A critical role for direct TLR2-MyD88 signaling in CD8 T-cell clonal expansion and memory formation following vaccinia viral infection. Blood. 2009; 113:2256–2264.


14. Schieber M, Chandel NS. ROS function in redox signaling and oxidative stress. Curr Biol. 2014; 24:R453–R462.


15. Yang CS, Shin DM, Kim KH, Lee ZW, Lee CH, Park SG, Bae YS, Jo EK. NADPH oxidase 2 interaction with TLR2 is required for efficient innate immune responses to mycobacteria via cathelicidin expression. J Immunol. 2009; 182:3696–3705.


16. Yuk JM, Shin DM, Yang CS, Kim KH, An SJ, Rho J, Park JK, Jo EK. Role of apoptosis-regulating signal kinase 1 in innate immune responses by Mycobacterium bovis bacillus Calmette-Guerin. Immunol Cell Biol. 2009; 87:100–107.


17. Shin DM, Yuk JM, Lee HM, Lee SH, Son JW, Harding CV, Kim JM, Modlin RL, Jo EK. Mycobacterial lipoprotein activates autophagy via TLR2/1/CD14 and a functional vitamin D receptor signalling. Cell Microbiol. 2010; 12:1648–1665.


18. Howard ST, Rhoades E, Recht J, Pang X, Alsup A, Kolter R, Lyons CR, Byrd TF. Spontaneous reversion of Mycobacterium abscessus from a smooth to a rough morphotype is associated with reduced expression of glycopeptidolipid and reacquisition of an invasive phenotype. Microbiology. 2006; 152:1581–1590.


19. Byrd TF, Lyons CR. Preliminary characterization of a Mycobacterium abscessus mutant in human and murine models of infection. Infect Immun. 1999; 67:4700–4707.


20. Kim TS, Kim YS, Yoo H, Park YK, Jo EK. Mycobacterium massiliense induces inflammatory responses in macrophages through Toll-like receptor 2 and c-Jun N-terminal kinase. J Clin Immunol. 2014; 34:212–223.


21. Kawai T, Akira S. Toll-like receptors and their crosstalk with other innate receptors in infection and immunity. Immunity. 2011; 34:637–650.


22. Oeckinghaus A, Ghosh S. The NF-kappaB family of transcription factors and its regulation. Cold Spring Harb Perspect Biol. 2009; 1:a000034.
23. Cruz-Knight W, Blake-Gumbs L. Tuberculosis: an overview. Prim Care. 2013; 40:743–756.
24. Huynh KK, Joshi SA, Brown EJ. A delicate dance: host response to mycobacteria. Curr Opin Immunol. 2011; 23:464–472.


25. Marazzi MG, Chapgier A, Defilippi AC, Pistoia V, Mangini S, Savioli C, Dell'Acqua A, Feinberg J, Tortoli E, Casanova JL. Disseminated Mycobacterium scrofulaceum infection in a child with interferon-gamma receptor 1 deficiency. Int J Infect Dis. 2010; 14:e167–e170.
26. Haas WH, Kirschner P, Ziesing S, Bremer HJ, Bottger EC. Cervical lymphadenitis in a child caused by a previously unknown mycobacterium. J Infect Dis. 1993; 167:237–240.


27. Tortoli E, Kirschner P, Springer B, Bartoloni A, Burrini C, Mantella A, Scagnelli M, Scarparo C, Simonetti MT, Bottger EC. . Cervical lymphadenitis due to an unusual mycobacterium. Eur J Clin Microbiol Infect Dis. 1997; 16:308–311.
28. Kamala T, Paramasivan CN, Herbert D, Venkatesan P, Prabhakar R. Isolation and Identification of Environmental Mycobacteria in the Mycobacterium bovis BCG Trial Area of South India. Appl Environ Microbiol. 1994; 60:2180–2183.


29. Torvinen E, Suomalainen S, Paulin L, Kusnetsov J. Mycobacteria in Finnish cooling tower waters. APMIS. 2014; 122:353–358.


30. Puthanakit T, Oberdorfer P, Ukarapol N, Akarathum N, Punjaisee S, Sirisanthana T, Sirisanthana V. Immune reconstitution syndrome from nontuberculous mycobacterial infection after initiation of antiretroviral therapy in children with HIV infection. Pediatr Infect Dis J. 2006; 25:645–648.


31. Brandt L, Feino CJ, Weinreich OA, Chilima B, Hirsch P, Appelberg R, Andersen P. Failure of the Mycobacterium bovis BCG vaccine: some species of environmental mycobacteria block multiplication of BCG and induction of protective immunity to tuberculosis. Infect Immun. 2002; 70:672–678.


32. Flaherty DK, Vesosky B, Beamer GL, Stromberg P, Turner J. Exposure to Mycobacterium avium can modulate established immunity against Mycobacterium tuberculosis infection generated by Mycobacterium bovis BCG vaccination. J Leukoc Biol. 2006; 80:1262–1271.


33. Barrow WW, Brennan PJ. Isolation in high frequency of rough variants of Mycobacterium intracellulare lacking C-mycoside glycopeptidolipid antigens. J Bacteriol. 1982; 150:381–384.


34. Recht J, Kolter R. Glycopeptidolipid acetylation affects sliding motility and biofilm formation in Mycobacterium smegmatis. J Bacteriol. 2001; 183:5718–5724.


35. Bernut A, Herrmann JL, Kissa K, Dubremetz JF, Gaillard JL, Lutfalla G, Kremer L. Mycobacterium abscessus cording prevents phagocytosis and promotes abscess formation. Proc Natl Acad Sci U S A. 2014; 111:E943–E952.
36. Rhoades ER, Archambault AS, Greendyke R, Hsu FF, Streeter C, Byrd TF. Mycobacterium abscessus Glycopeptidolipids mask underlying cell wall phosphatidyl-myo-inositol mannosides blocking induction of human macrophage TNF-alpha by preventing interaction with TLR2. J Immunol. 2009; 183:1997–2007.


37. Jonsson B, Ridell M, Wold AE. Phagocytosis and cytokine response to rough and smooth colony variants of Mycobacterium abscessus by human peripheral blood mononuclear cells. APMIS. 2013; 121:45–55.


38. Means TK, Wang S, Lien E, Yoshimura A, Golenbock DT, Fenton MJ. Human toll-like receptors mediate cellular activation by Mycobacterium tuberculosis. J Immunol. 1999; 163:3920–3927.
39. Wang T, Lafuse WP, Zwilling BS. Regulation of toll-like receptor 2 expression by macrophages following Mycobacterium avium infection. J Immunol. 2000; 165:6308–6313.


40. Vignal C, Guerardel Y, Kremer L, Masson M, Legrand D, Mazurier J, Elass E. Lipomannans, but not lipoarabinomannans, purified from Mycobacterium chelonae and Mycobacterium kansasii induce TNF-alpha and IL-8 secretion by a CD14-toll-like receptor 2-dependent mechanism. J Immunol. 2003; 171:2014–2023.


41. Kleinnijenhuis J, Oosting M, Joosten LA, Netea MG, Van CR. Innate immune recognition of Mycobacterium tuberculosis. Clin Dev Immunol. 2011; 2011:405310.
42. Fremond CM, Togbe D, Doz E, Rose S, Vasseur V, Maillet I, Jacobs M, Ryffel B, Quesniaux VF. IL-1 receptor-mediated signal is an essential component of MyD88-dependent innate response to Mycobacterium tuberculosis infection. J Immunol. 2007; 179:1178–1189.


43. Yoshida A, Inagawa H, Kohchi C, Nishizawa T, Soma G. The role of toll-like receptor 2 in survival strategies of Mycobacterium tuberculosis in macrophage phagosomes. Anticancer Res. 2009; 29:907–910.
44. Koul A, Herget T, Klebl B, Ullrich A. Interplay between mycobacteria and host signalling pathways. Nat Rev Microbiol. 2004; 2:189–202.


45. Schorey JS, Cooper AM. Macrophage signalling upon mycobacterial infection: the MAP kinases lead the way. Cell Microbiol. 2003; 5:133–142.


46. Jung SB, Yang CS, Lee JS, Shin AR, Jung SS, Son JW, Harding CV, Kim HJ, Park JK, Paik TH, Song CH, Jo EK. The mycobacterial 38-kilodalton glycolipoprotein antigen activates the mitogen-activated protein kinase pathway and release of proinflammatory cytokines through Toll-like receptors 2 and 4 in human monocytes. Infect Immun. 2006; 74:2686–2696.


47. Sim YS, Kim SY, Kim EJ, Shin SJ, Koh WJ. Impaired Expression of MAPK Is Associated with the Downregulation of TNF-alpha, IL-6, and IL-10 in Mycobacterium abscessus Lung Disease. Tuberc Respir Dis (Seoul). 2012; 72:275–283.


48. Launois P, Niang M, Dieye A, Sarthou JL, Rivier F, Millan J. Human phagocyte respiratory burst by Mycobacterium bovis BCG and M. leprae: functional activation by BCG is mediated by complement and its receptors on monocytes. Int J Lepr Other Mycobact Dis. 1992; 60:225–233.
49. Ferrari CK, Souto PC, Franca EL, Honorio-Franca AC. Oxidative and nitrosative stress on phagocytes function: from effective defense to immunity evasion mechanisms. Arch Immunol Ther Exp (Warsz). 2011; 59:441–448.


50. Vazquez-Torres A, Fang FC. Oxygen-dependent anti-Salmonella activity of macrophages. Trends Microbiol. 2001; 9:29–33.


51. Lim YJ, Choi HH, Choi JA, Jeong JA, Cho SN, Lee JH, Park JB, Kim HJ, Song CH. Mycobacterium kansasii-induced death of murine macrophages involves endoplasmic reticulum stress responses mediated by reactive oxygen species generation or calpain activation. Apoptosis. 2013; 18:150–159.


52. Kim JJ, Lee HM, Shin DM, Kim W, Yuk JM, Jin HS, Lee SH, Cha GH, Kim JM, Lee ZW, Shin SJ, Yoo H, Park YK, Park JB, Chung J, Yoshimori T, Jo EK. Host cell autophagy activated by antibiotics is required for their effective antimycobacterial drug action. Cell Host Microbe. 2012; 11:457–468.


53. Vilcheze C, Hartman T, Weinrick B, Jacobs WR Jr. Mycobacterium tuberculosis is extraordinarily sensitive to killing by a vitamin C-induced Fenton reaction. Nat Commun. 2013; 4:1881.
54. Romero MM, Balboa L, Basile JI, Lopez B, Ritacco V, de la Barrera SS, Sasiain MC, Barrera L, Aleman M. Clinical isolates of Mycobacterium tuberculosis differ in their ability to induce respiratory burst and apoptosis in neutrophils as a possible mechanism of immune escape. Clin Dev Immunol. 2012; 2012:152546.