Abstract
There is growing evidence that crosstalk between mantle cell lymphoma (MCL) cells and stromal microenvironments, such as bone marrow and secondary lymphoid tissues, promotes tumor progression by enhancing survival and growth as well as drug resistance of MCL cells. Recent advances in the understanding of lymphoma microenvironment have led to the identification of crucial factors involved in the crosstalk and subsequent generation of their targeted agents. In the present study, we evaluated the combinatory effect of blocking antibodies (Ab) targeting CXCR4 and VLA-4, both of which were known to play significant roles in the induction of environment-mediated drug resistance (EMDR) in MCL cell line, Jeko-1. Simultaneous treatment with anti-CXCR4 and anti-VLA-4 Ab not only reduced the migration of Jeko-1 cells into the protective stromal cells, but also enhanced sensitivity of Jeko-1 to a chemotherapeutic agent to a greater degree than with either Ab alone. These combinatorial effects were associated with decreased phosphorylation of ERK1/2, AKT and NF-κB. Importantly, drug resistance could not be overcome once the adhesion of Jeko-1 to the stromal occurred despite the combined use of Abs, suggesting that the efforts to mitigate migration of MCLs should be attempted as much as possible. Our results provide a basis for a future development of therapeutic strategies targeting both CXCR4 and VLA-4, such as Ab combinations or bispecific antibodies, to improve treatment outcomes of MCL with grave prognosis.
Mantle cell lymphoma (MCL) is an aggressive and distinct subtype of non-Hodgkin's lymphoma (NHL) that accounts for only 6% of its cases and known to be derived from naïve CD5+ B cells originating from the mantle zone surrounding reactive germinal centers (1). Despite substantial efforts to improve clinical outcomes over the past few decades, long-term disease-free survival rate of MCL remains low primarily due to the persistence of minimal residual disease (MRD) that causes the relapse of MCL and subsequent treatment failure. Cells that constitute MRD have capacity to find refuge in protective microenvironments and the selective pressure of chemotherapy leads to the development of acquired resistance in these surviving cells and eventually promotes the outgrowth of MRD. A previous report also proposed that environment-mediated drug resistance (EMDR) contributes substantially to MRD by transiently protecting tumor cells from cell death induced by chemotherapy or radiation (2). Increasing evidence suggests that the interactions between MCL cells and stromal microenvironment, including lymphatic tissues, are critical for the induction of drug resistance in MCL to chemotherapy (3,4).
CXCR4, a chemokine receptor for CXCL12, is highly expressed on the surface of cells in various hematologic malignancies (5,6,7,8,9,10). CXCL12 is constitutively secreted by stromal cells and induces trafficking and homing of the cancer cells to the supportive microenvironment, where stromal cells provide growth as well as drug resistance signals to cancer cells staying in close contact. Accordingly, the CXCR4/CXCL12 axis has been considered as a major contributor to EMDR and targeted agents against CXCR4 has been emerging as potential treatment modality for various types of malignancies (11,12). VLA-4, also known as α4β1 integrin, is a cell surface receptor that interacts with extracellular matrix (ECM) proteins, such as fibronectin and vascular cell adhesion molecule-1 (VCAM-1), expressed by stromal cells (13). Cell adhesion mediated by VLA-4 confers protection against drug-induced apoptosis in various types of cancers and this phenomenon is commonly described as cell-adhesion mediated drug resistance (CAM-DR) (14). CXCL12 has been reported to induce firm adhesion and migration by inducing activation of VLA-4 on hematopoietic stem cells (15). Conversely, adhesion molecules may trigger signals for both enhanced CXCR4 expression and increased function (16). Additionally, the CXCR4/CXCL12 axis interacts with VLA-4 to regulate migration and adhesion of malignant cells in the bone marrow microenvironment (17). MCL cells express high levels of both CXCR4 and VLA-4, and adhere and spontaneously migrate beneath marrow stromal cells (MSCs) in a CXCR4- and VLA-4-dependent fashion (3). Taken together, the interactions between CXCR4 and VLA-4 constitute a 2-way pathway where both receptors work cooperatively. Therefore, it is expected that the combined blockade of both CXCR4 and VLA-4 is more effective in overcoming EMDR than solely blocking the respective receptor. Few studies today have investigated the effect of combined inhibition of both receptors by using specific antibodies (Abs). Here, the combined use of specific Abs for both receptors was tested to see whether there is an additive or combinatorial effect to overcome EMDR. These results may provide a basis for future development of therapeutic tools targeting both CXCR4 and VLA-4 such as bispecific antibody (Ab), for instance, to improve the treatment outcome of MCL which has a grave prognosis.
Mantle cell lymphoma cell line Jeko-1 was purchased from ATCC (Manassas, VA, USA) and maintained in RPMI-1640 containing 20% fetal bovine serum (FBS; Gibco by Life Technologies, Carlsbad, CA, USA) and 1% penicillin-streptomycin (Gibco by Life Technologies). Cultures were maintained by the addition of fresh replacement medium every 2 to 3 days. The murine stromal cell line M2-10B4 was purchased from ATCC (Manassas, VA, USA). Cells were maintained in RPMI-1640 containing 10% FBS and 1% penicillin-streptomycin. Cultures were maintained by removing medium, rinsing with 0.25% Trypsin-EDTA solution (Gibco by Life Technologies) followed by the addition of a fresh culture medium every 2 to 3 days.
Recombinant human CXCL12 (SDF-1α) was purchased (R&D Systems, Minneapolis, MN, USA). The following monoclonal Abs specific for human surface antigens were used: Purified NA/LE mouse anti-human CD184, FITC anti-human CD49d and FITC mouse anti-human CD45 (BD Pharmingen, San Jose, CA, USA). For inhibition experiments, anti-CXCR4 Ab (Ab) and anti-integrin alpha 4 Ab [P1H4] azide free for VLA-4 were purchased (abcam, Cambridge, UK).
For flow cytometry, the cells were adjusted to a concentration 1×106 cell/ml in HBSS with 2% FBS (FACS buffer). The cells were stained with saturating Ab concentration for 30 minutes at 4℃, washed and analyzed on a FACSCalibur™ (BD Bioscience, San Jose, CA, USA).
5×105 Jeko-1 was added to a volume of 100µl RPMI-1640 with 0.5% BSA in the upper chamber and SDF-1α in the lower chamber of transwell insert. After the incubation, the upper chambers were placed onto the lower chamber at 37℃ for 2 hours where migrated cells in lower chamber were suspended and divided into aliquots. This was used to count with FACSCalibur. Among the concentration of SDF-1α, We used only the concentration that induced maximal migration of Jeko-1 for the subsequent experiments.
For the migration inhibition assay, Jeko-1 (5×105 cells in a volume of 100µl RPMI-1640 with 0.5% BSA) treated with varying concentrations of the anti-CXCR4 Ab were added to the upper chamber of 6.5-mm diameter Transwell inserts (Corning Life Sciences, Acton, MA, USA) with a 5µm pore size and incubated at 37℃ for 30 minutes. The upper chambers were transferred to the wells containing a medium in 1% antibiotics-containing RPMI-1640 with 100 ng/ml SDF-1α. The chambers were incubated 2 hours at 37oC in 5% CO2. After the incubation, the cells in the lower chamber were suspended and stained with FITC anti-human CD45. The percentage of transmigrated cells in the lower chamber was analyzed using FACSCalibur. Among the concentration of anti-CXCR4 in the upper chamber, only the concentration that induced minimum migration of Jeko-1 was used for the subsequent experiments.
To test effects of combined use of Abs on the migration, MCL cell line was preincubated with anti-CXCR4, anti-VLA-4 or both Abs in the upper chamber at 37℃ for 30 minutes, after which the cells were analyzed for migration to the lower chamber in response to 100 ng/ml SDF-1α after incubation for 2 hrs in 5% CO2.
M2-10B4 stromal cells were seeded onto gelatin (Sigma Aldrich, St Louis, MO)-coated 12-well plates at a concentration of 1.5×105 cells per well in RPMI-1640 with 1% antibiotics. After overnight incubation, MCL cell lines were added onto the confluent stromal cell layers to a final concentration of 1.0×106 cells per well. For inhibition experiments, MCL cells were preincubated for 1 hour with 2.5µg/ml, 5µg/ml and 10µg/ml of anti-VLA4 Ab, respectively. The plates were then incubated for 6 hours at 37℃ in 5% CO2. After the removal of non-migrated cells by vigorously washing the plate, stromal cell layers containing transmigrated cells were photographed with an inverted microscope.
In 24-well plates, MCL cell lines were cocultured with MSCs to investigate the roles of M2-10B4 cells. MCL cell lines were treated by anti-CXCR4 and anti-VLA-4 Ab and incubated for 30 minutes at 37℃. Then, 40 ng/ml cytosine arabinoside (Ara-C; kindly provided by JW pharmaceutical, Seoul, South Korea) was added to wells. The cells were rinsed with 0.25% trypsin, suspended with FACS buffer and MCL cell apoptosis and death were assessed by flow cytometry using Annexin V Apoptosis Detection Kit APC (eBioscience, San Diego, CA, USA) and Propidium Idodide Staining Solution (BD Pharmingen) after electronic gating on CD45+ (for MCL cell lines).
To test the actual effect of migration on drug resistance, we had the cells migrate through Transwell insert and compared the degree of apoptosis between the cells in two compartments. The Abs were added onto the upper chamber and preincubated for 30 minutes. Inserts were then transferred to the wells containing MSC and incubated for 2 hrs at 37℃ in 5% CO2. After this migration, 40 ng/ml of Ara-C was added to the upper and lower chamber, respectively. The cells were then incubated for 48 hours at 37℃. Cells from the upper chamber were analyzed for apoptosis and cell death by using flow cytometry. In the lower chamber, the cells were gated on CD45 to distinguish MCL cells from MSCs after they were rinsed, washed and suspended with FACS buffer. Then, these cells were analyzed for apoptosis and cell death.
After coculture of cells with MSCs in 24-well plates in the presence of the Abs, the cells were incubated with Ara-C for 48 hrs to check the status of downstream effector proteins. After this incubation, the cells suspended with FACS buffer were stained with anti-human CD45 FITC (eBioscience), fixed with IC fixation buffer (eBioscience), then permeabilized with 100% methanol and then added anti-Hu/Mo pERK1/2 (T204/Y202) APC, anti-human pNF-κB p65 (S529) eFlour® 660 and Alexa Flour® 647 mouse anti-AKT (pS473) (eBioscience). The stained cells were re-suspended in 300µl FACS buffer and detected using FACSCalibur™.
Results are expressed as the mean and all experiments were performed in triplicate. Differences between groups were assessed using a 2-independent-sample t-test. Multiple comparisons were done with chi-square tests of crosstabs. The differences were considered statistically significant when p-values <0.05. SPSS software version ver. 16.0 (SPSS, Chicago, IL, USA) was used analyze data throughout the study.
Fig. 2A displays the chemotactic response of MCL cell line Jeko-1 to varying concentrations of SDF-1α. SDF-1α elicited maximum migration at a concentration of 100 ng/ml; more than 80% of input cells migrated through the micro pore filters, whereas stimulation with 200 ng/ml and 300 ng/ml SDF-1α resulted in less migration compared to the concentration of 100 ng/ml. We have used 100 ng/ml of SDF-1α for the subsequent experiments.
Chemotaxis of MCL cells was significantly inhibited by preincubation with anti-CXCR4 Ab at a concentration of 7.5µg/ml in the presence of 100 ng/ml SDF-1α (p=0.005, Fig. 2B). We have used this concentration anti-CXCR4 Ab for the subsequent experiments.
Coculture of MCL cell lines with M2-10B4 stromal cells resulted in spontaneous migration of MCL cell lines beneath stromal cell layer. During this in vitro phenomenon known as pseudo-emperipolesis, because migrated cells are localized within the same focal plane as the stromal cells, the cells acquire dark appearance. On the contrary, non-migrated cells remain refractile by inverted microscopy (Fig. 3B). Pseudoemperipolesis of Jeko-1 pre-treated with anti-VLA4 Ab was markedly reduced (Fig. 3C). However, there were little differences in inhibiting pseudoemperipolesis based on the concentration of VLA-4 Ab (data not shown). Therefore, we have used 2.5µg/ml of SDF-1α for the subsequent experiment, which is the lowest concentration tested.
Migration of MCL cell line was significantly reduced by 6.3% and 16.7% with anti-CXCR4 and anti-VLA-4 Ab, respectively, in terms of absolute value in percentage of input compared with control. Combined treatment with both Abs markedly inhibited the migration of MCL cell lines by 24.9% (p=0.009, Fig. 4).
We tested the protective effect of MSCs on Jeko-1 treated with Ara-C to investigate whether MCL cell lines exhibit CAM-DR in the stromal microenvironment. We observed definite protective effect of MSCs on the survival of MCL cell lines. Marked decrease in apoptosis (Fig. 5B) and apoptosis + cell death (Fig. 5D) was observed in the presence of MSCs in comparison to in the absence of MSCs (Fig. 5A and C). 53.6% of MCL cell lines grown without MSCs were apoptotic, whereas only 15.9% of MCL cell lines cocultured with MSCs were apoptotic (Table I). The administration of anti-CXCR4 or anti-VLA-4 Ab increased apoptosis and apoptosis+cell death regardless of the existence of MSCs (p=0.004, respectively). These effects were most prominent when both Abs were used simultaneously, demonstrating a synergistic effect on inducing apoptosis and apoptosis+cell death (See differences between pre- and post-Ab treatment value, Table I).
In the presence of MSCs, increased phosphorylation of MCL cell lines was observed when compared to the absence of MSCs; the phosphorylation increased approximately twice (Table II). The addition of the Abs resulted in decreased phosphorylation of the proteins regardless of MSC and the reduction was most prominent when the cells were treated with both Abs simultaneously (Fig. 6). The degree of reduction in phosphorylation was more striking in the presence of MSCs demonstrating combinatorial reduction. Differences in phosphorylation for ERK1/2 (the values with Ab - the value of the control) were -1.9, -1.9 and -4.9, respectively with anti-CXCR4, anti-VLA-4 and both Abs. For AKT, the values were -1.9, -4.5 and -7.2, respectively. For NF-κB, the values were -2.1, -3.9 and -5.0, respectively. Although reductions of the phosphorylation were observed in the absence of MSCs (p=0.05, respectively), the differences were not prominent as in the presence of MSCs. The decrease in phosphorylation was combinatorial only for AKT; the others were less than additive.
After exposure of the MCL cells to Abs, we have the cells migrate from upper chamber of Transwell insert to the lower chamber and compared the drug resistance of the cells in each chamber to see the influence of migration on the resistance. The remained cells in the upper chamber after exposure to anti-CXCR4 or anti-VLA-4 Ab showed increased apoptosis and apoptosis+cell death, and the increment was most prominent in the presence of both Abs (p=0.01, Fig. 7A and B). This finding demonstrates that combined use of the Abs induces a combinatorial increase in the degree of apoptosis (upper chamber, Table III). Meanwhile, the migrated cells in the lower chamber, and in turn, adhered to MSCs or underwent pseudoemperipolesis demonstrated marked decrease in apoptosis, regardless of Ab treatment, compared to cells in the upper chamber (lower chamber, Table II). There was no statistical difference in the level of apoptosis according to the addition of Abs. Similar results were seen in apoptosis+cell death (Fig. 7B and Table II).
In this study, we could reconfirm a few findings from the previous works. First, MCL cell line Jeko-1 highly expresses both CXCR4 and VLA-4 on their surface. Secondly, chemokine CXCL12 enhances migration of the MCL cell lines and the migration can be inhibited by blocking Abs. Thirdly, MCL cells migrate beneath MSCs spontaneously (pseudo-emperipolesis) and this phenomenon can be inhibited by anti-VLA-4 Ab. Fourthly, MSCs protect MCL cell lines from cytotoxic agents indicating stromal microenvironment plays an important role in EMDR.
EMDR is one form of de novo drug resistance where tumor cells evade apoptosis transiently through triggers such as chemotherapy, radiotherapy or receptor-mediated cell death (18,19). Drug resistance can be subdivided into two categories: soluble factor mediated drug resistance (SFM-DR) and cell adhesion-mediated drug resistance (CAM-DR). The CXCR4/CXCL12 axis and VLA-4/ECM or stromal fibroblasts axis are known to be major components of SFM-DR and CAM-DR, respectively. Therefore, blocking these major components of EMDR simultaneously may be an attractive strategy to cure some type of cancers. There have been many reports that the blockade of CXCR4 and VLA-4, respectively, abrogate migration and/or adhesion, which could lead to overcoming drug resistance to chemotherapeutic agents (3,17,20). However, few papers investigated the effect of simultaneous blockade of both receptors on migration and drug resistance as far as we know. In our study, we found that on the chemotaxis assay, anti-CXCR4 alone and anti-VLA-4 Ab alone could reduce migration of MCL cell lines and the combinatorial effect in the reduction was observed when both Abs were used simultaneously. The combinatorial effect of combined use of Abs may have originated from the findings that CXCR4 and VLA-4 constitutes a 2-way pathway and both receptors work cooperatively in homing and adhesion to the stromal microenvironment; VLA-4 interacts with SDF-1α directly (17) and, SDF-1α induces activation of VLA-4 (15). Influences of SDF-1α on VLA-4 may be blocked by combining treatment with both Abs. Otherwise, the continued activation by SDF-1α sustains inducing the migration of the cells. We think this finding has an important clinical implication because the less hematologic malignancy cells migrate to the stromal microenvironment conferring drug resistance to chemotherapeutic agents, the higher the probability of overcoming EMDR will be. In animal studies, we expect the addition of antagonists for integrin such as VLA-4 to CXCR4 antagonist to be more effective in inhibiting migration because of the vascular endothelial cells, which are the first barrier of transmigration of tumor cell to the stromal microenvironment, also express integrin. This prediction should be verified in the future experiments.
In the presence of MSCs, apoptosis was markedly suppressed, but the addition of Abs enhanced apoptosis and/or cell death, specifically when Abs were combined, resulting in a combinatorial increase. This finding suggests that even under the protective influence of MSCs, combined use of Abs can overcome EMDR more effectively. One of the mechanisms of MSCs to induce drug resistance has been known to be the activation of downstream signaling pathways. In our study, combined use of both Abs induced maximal apoptosis and apoptosis+cell death as well as the lowest phosphorylation indicating the maximal apoptosis is associated with decreased phosphorylation. This finding suggests that the mechanism of overcoming drug resistance by the blocking Abs is through the suppression of downstream pathways. We also found that combined use of Abs produced a combinatorial effect on the suppression of phosphorylation, especially in the presence of MSCs, which implies that simultaneous treatment with both Abs can overcome EMDR more effectively. Since downstream pathways including PI3K/AKT/mTOR and NF-κB are known to be activated in MCL (21,22), combined treatment of blocking Abs that suppresses these pathways seems to be a reasonable and potential therapeutic tool.
Finally, drug resistance according to migration of MCL cells was investigated. Although Ab treatment could enhance apoptosis of MCL cells after exposure to Abs (the cells remained in the upper chamber of Transwell insert), migrated cells (escaped from such blockade and, in turn adhered to MSCs or migrated beneath the MSCs) showed markedly reduced apoptosis regardless of Ab combination. This suggests that combined Ab treatment could not reverse drug resistance once the cancer cells migrated and adhered to MSCs. Efforts to reduce migration and hence block CAM-DR as much as possible should be attempted to reduce DR, which may be able to be accomplished maximally by the combined use.
Inhibiting of chemokine receptors and/or integrins may be of concern as it may have a detrimental outcome such as immune deficiency because these molecules play an important role in lymphocyte development (23,24). Despite having numerous genetic lesions, some cancers cells rely on one single dominant oncogene for growth and survival, so that inhibition of this gene is sufficient to halt cancer growth. This concept is called 'oncogene addiction' and considerable evidence, both from basic and clinical research, has shown that this concept is valid (25). Whether normal lymphocyte development is less damaged by antagonizing CXCR4 and/or VLA-4 due to the tendency of cancer cells to be dependent on one or two pathways preferentially (oncologic addiction) should be clarified in the future studies.
The combined blockade of CXCR4 and VLA-4, which are major components of SFM-DR and CAM-DR, respectively, resulted in synergistic effects in reducing migration and inducing apoptosis of MCL cells. These findings are associated with reduced phosphorylation of downstream signaling proteins. The result of our study may provide a basis for future development of therapeutic tools targeting both CXCR4 and VLA-4 such as bispecific antibody, for instance, to improve treatment outcome of MCL with a grave prognosis.
Figures and Tables
Figure 1
Expression of CXCR4 and VLA-4. Surface expression of CXCR4 (A) and VLA-4 (B) was determined by FlowJo analysis. Most of Jeko-1 expressed CXCR4 and VLA-4.
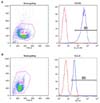
Figure 2
Chemotaxis in MCL cell line in response to SDF-1α and anti-CXCR4 Ab. (A) Migration of Jeko-1 cells increased in response to SDF-1α, peaked at 100 ng/ml, but without statistical significance. (B) SDF-1α-induced chemotaxis was inhibited by pretreatment with anti-CXCR4 Ab.
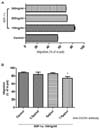
Figure 3
Pseudo-emperipolesis of MCL cell. (A) Control, with marrow stromal cells only. (B) Stromal cells with MCL cells. Migrated cells are characterized by a dark appearance, whereas non-migrated cells remain bright. (C) Stromal cells and MCL cells with anti-VLA-4 Ab. Reduced migration beneath marrow stromal cells (pseudo-emperipolesis) was observed in the presence of anti-VLA-4 Ab. Original magnification ×20.
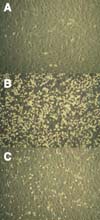
Figure 4
Effects of combined blockage by anti-CXCR4 and VLA-4 antibody. The migration was inhibited by preincubation of anti-CXCR4 (7.5µg/ml) and VLA-4 Ab (2.5µg/ml). *denotes statistical significance at p<0.05, compared with control sample.
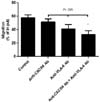
Figure 5
Effects of blocking antibodies on the protective effect of MSCs. In 24 well plate, MCL cells were cultured in the absence or presence of MSCs with Ara-C (A and C, MCL cells without MSC: B and D, MCL with MSC). Results are percentages of cells of apoptosis and apoptosis and cell death. **denotes statistical significance at p<0.01, compared with the control sample.
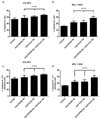
Figure 6
Phosphorylation of signaling pathways in MCL cells in response to chemotherapy Ara-C. MCL cells pre-treated with antibodies in the absence (A, B, and C) or presence (D, E, and F) of MSCs with Ara-C in a 24 well plate for 48 hours. Phosphorylation of ERK1/2, AKT and NF-κB was examined by flow cytometry. Phosphorylation of each signaling protein was markedly suppressed with the addition of antibodies, especially in the presence of both antibodies. *denotes statistical significance at p<0.05, compared with control sample.
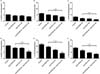
Figure 7
Effects of migration of MCL cells on apoptosis and cell death. MCL cells pretreated with Abs in the upper chamber were analyzed by annexin V (A) and PI (B) flow cytometry and compared with the cells migrated to the lower chamber. Results are expressed as percentages of apoptotic, necrotic and dead MCL cells. *denotes statistical significance at p<0.05, compared with control sample.
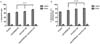
References
1. The Non-Hodgkin's Lymphoma Classification Project. A clinical evaluation of the International Lymphoma Study Group classification of non-Hodgkin's lymphoma. Blood. 1997; 89:3909–3918.
2. Matsunaga T, Takemoto N, Sato T, Takimoto R, Tanaka I, Fujimi A, Akiyama T, Kuroda H, Kawano Y, Kobune M, Kato J, Hirayama Y, Sakamaki S, Kohda K, Miyake K, Niitsu Y. Interaction between leukemic-cell VLA-4 and stromal fibronectin is a decisive factor for minimal residual disease of acute myelogenous leukemia. Nat Med. 2003; 9:1158–1165.


3. Kurtova AV, Tamayo AT, Ford RJ, Burger JA. Mantle cell lymphoma cells express high levels of CXCR4, CXCR5, and VLA-4 (CD49d): importance for interactions with the stromal microenvironment and specific targeting. Blood. 2009; 113:4604–4613.


4. Lwin T, Hazlehurst LA, Dessureault S, Lai R, Bai W, Sotomayor E, Moscinski LC, Dalton WS, Tao J. Cell adhesion induces p27Kip1-associated cell-cycle arrest through down-regulation of the SCFSkp2 ubiquitin ligase pathway in mantle-cell and other non-Hodgkin B-cell lymphomas. Blood. 2007; 110:1631–1638.


5. Mohle R, Failenschmid C, Bautz F, Kanz L. Overexpression of the chemokine receptor CXCR4 in B cell chronic lymphocytic leukemia is associated with increased functional response to stromal cell-derived factor-1 (SDF-1). Leukemia. 1999; 13:1954–1959.


6. Bradstock KF, Makrynikola V, Bianchi A, Shen W, Hewson J, Gottlieb DJ. Effects of the chemokine stromal cell-derived factor-1 on the migration and localization of precursor-B acute lymphoblastic leukemia cells within bone marrow stromal layers. Leukemia. 2000; 14:882–888.


7. Dialynas DP, Shao L, Billman GF, Yu J. Engraftment of human T-cell acute lymphoblastic leukemia in immunodeficient NOD/SCID mice which have been preconditioned by injection of human cord blood. Stem Cells. 2001; 19:443–452.


8. Hideshima T, Anderson KC. Molecular mechanisms of novel therapeutic approaches for multiple myeloma. Nat Rev Cancer. 2002; 2:927–937.


9. Tavor S, Petit I, Porozov S, Avigdor A, Dar A, Leider-Trejo L, Shemtov N, Deutsch V, Naparstek E, Nagler A, Lapidot T. CXCR4 regulates migration and development of human acute myelogenous leukemia stem cells in transplanted NOD/SCID mice. Cancer Res. 2004; 64:2817–2824.


10. Beider K, Ribakovsky E, Abraham M, Wald H, Weiss L, Rosenberg E, Galun E, Avigdor A, Eizenberg O, Peled A, Nagler A. Targeting the CD20 and CXCR4 pathways in non-hodgkin lymphoma with rituximab and high-affinity CXCR4 antagonist BKT140. Clin Cancer Res. 2013; 19:3495–3507.


11. Lavrovsky Y, Ivanenkov YA, Balakin KV, Medvedeva DA, Ivachtchenko AV. CXCR4 receptor as a promising target for oncolytic drugs. Mini Rev Med Chem. 2008; 8:1075–1087.


12. Burger JA, Peled A. CXCR4 antagonists: targeting the microenvironment in leukemia and other cancers. Leukemia. 2009; 23:43–52.


13. Shishido S, Bonig H, Kim YM. Role of integrin alpha4 in drug resistance of leukemia. Front Oncol. 2014; 4:99.


14. Podar K, Tai YT, Lin BK, Narsimhan RP, Sattler M, Kijima T, Salgia R, Gupta D, Chauhan D, Anderson KC. Vascular endothelial growth factor-induced migration of multiple myeloma cells is associated with beta 1 integrin- and phosphatidylinositol 3-kinase-dependent PKC alpha activation. J Biol Chem. 2002; 277:7875–7881.


15. Peled A, Kollet O, Ponomaryov T, Petit I, Franitza S, Grabovsky V, Slav MM, Nagler A, Lider O, Alon R, Zipori D, Lapidot T. The chemokine SDF-1 activates the integrins LFA-1, VLA-4, and VLA-5 on immature human CD34(+) cells: role in transendothelial/stromal migration and engraftment of NOD/SCID mice. Blood. 2000; 95:3289–3296.


16. Ding Z, Issekutz TB, Downey GP, Waddell TK. L-selectin stimulation enhances functional expression of surface CXCR4 in lymphocytes: implications for cellular activation during adhesion and migration. Blood. 2003; 101:4245–4252.


17. Ngo HT, Leleu X, Lee J, Jia X, Melhem M, Runnels J, Moreau AS, Burwick N, Azab AK, Roccaro A, Azab F, Sacco A, Farag M, Sackstein R, Ghobrial IM. SDF-1/CXCR4 and VLA-4 interaction regulates homing in Waldenstrom macroglobulinemia. Blood. 2008; 112:150–158.


18. Meads MB, Hazlehurst LA, Dalton WS. The bone marrow microenvironment as a tumor sanctuary and contributor to drug resistance. Clin Cancer Res. 2008; 14:2519–2526.


19. Damiano JS, Cress AE, Hazlehurst LA, Shtil AA, Dalton WS. Cell adhesion mediated drug resistance (CAM-DR): role of integrins and resistance to apoptosis in human myeloma cell lines. Blood. 1999; 93:1658–1667.


20. Zeng Z, Shi YX, Samudio IJ, Wang RY, Ling X, Frolova O, Levis M, Rubin JB, Negrin RR, Estey EH, Konoplev S, Andreeff M, Konopleva M. Targeting the leukemia microenvironment by CXCR4 inhibition overcomes resistance to kinase inhibitors and chemotherapy in AML. Blood. 2009; 113:6215–6224.


21. Rizzatti EG, Falcao RP, Panepucci RA, Proto-Siqueira R, Anselmo-Lima WT, Okamoto OK, Zago MA. Gene expression profiling of mantle cell lymphoma cells reveals aberrant expression of genes from the PI3K-AKT, WNT and TGFbeta signalling pathways. Br J Haematol. 2005; 130:516–526.


22. Perez-Galan P, Mora-Jensen H, Weniger MA, Shaffer AL 3rd, Rizzatti EG, Chapman CM, Mo CC, Stennett LS, Rader C, Liu P, Raghavachari N, Stetler-Stevenson M, Yuan C, Pittaluga S, Maric I, Dunleavy KM, Wilson WH, Staudt LM, Wiestner A. Bortezomib resistance in mantle cell lymphoma is associated with plasmacytic differentiation. Blood. 2011; 117:542–552.


23. Bleul CC, Fuhlbrigge RC, Casasnovas JM, Aiuti A, Springer TA. A highly efficacious lymphocyte chemoattractant, stromal cell-derived factor 1 (SDF-1). J Exp Med. 1996; 184:1101–1109.

