Abstract
It is debatable whether AMP-activated protein kinase (AMPK) activation is involved in anti-apoptotic or pro-apoptotic signaling. AICAR treatment increases AMPK-α1 phosphorylation, decreases intracellular reactive oxygen species (ROS) levels, and significantly increases Annexin V-positive cells, DNA laddering, and caspase activity in human myeloid cell. AMPK activation is therefore implicated in apoptosis. However, AMPK-α1-knockdown THP-1 cells are more sensitive to apoptosis than control THP-1 cells are, suggesting that the apoptosis is AMPK-independent. Low doses of AICAR induce cell proliferation, whereas high doses of AICAR suppress cell proliferation. Moreover, these effects are significantly correlated with the downregulation of intracellular ROS, strongly suggesting that AICAR-induced apoptosis is critically associated with the inhibition of NADPH oxidase by AICAR. Collectively, our results demonstrate that in AICAR-induced apoptosis, intracellular ROS levels are far more relevant than AMPK activation.
AMP-activated protein kinase (AMPK) is a member of a family of protein kinases that have been highly evolutionarily conserved in animals, plants, and yeast, and which perform significant functions in cell responses to metabolic stress (1,2). Activated AMPK suppresses the principal enzymes involved in ATP-consuming anabolic pathways, and also effects increases in intracellular ATP (1,3). With regard to the protective activation of AMPK from metabolic stresses, however, a controversy persists as to whether AMPK is involved in anti-apoptotic or pro-apoptotic signaling, as the results thus far have been contradictory. It appears that either cell-type specificity or some other complicated mechanism is involved in this process.
5-aminoimidazole-4-carboxamide riboside (AICAR) is a widely employed AMPK activator (4). AICAR cannot be detected in normal healthy cells, but the mono- and tri-phosphorylated forms of AICAR can be detected in the blood of patients with congenital purine metabolism defects (5). AICAR exerts a variety of both in vivo and in vitro effects, presumably occurring via an AMPK-mediated pathway. These effects include the inhibition of growth and the depletion of pyrimidine nucleotide pools in fibroblasts (6,7), accelerated repletion of purine nucleotide pools in the heart (8), reduction of endurance in skeletal muscles (9), the inhibition of fatty acid and sterol synthesis and gluconeogenesis in hepatocytes, and an increase in glucose uptake in muscles (3). It also functions as an immunomodulator, and can ameliorate the symptoms of multiple sclerosis (MS) (10). A previous study showed that acadecine-induced AMPK activation reduces both translocation to the cell membrane and the phosphorylation of a cytosolic component of NADPH oxidase, thereby indicating that AICAR may exert antioxidant effects (11). Although numerous studies have reported that AICAR induces apoptosis in several cell types, including B-cell chronic lymphocytic leukemia cells and cancer cells, the mechanism by which AICAR elicits apoptosis, and whether AICAR acts as an antioxidant during this process, remain unclear.
The intracellular production and accumulation of reactive oxygen species (ROS), including superoxide radicals, singlet oxygen, hydrogen peroxide (H2O2), and hydroxyl radicals, can be induced by a variety of stimuli with complex effects, and can positively or negatively affect cellular events (12,13). A number of recent studies have shown that ROS are important signal transduction molecules and mediators of damage in cellular processes, including apoptosis and cell necrosis (14,15). Among the ROS, H2O2 is known to be rapidly inducible in response to peptide growth factors, including insulin, epidermal growth factor, and platelet-derived growth factor in non-phagocytic cells, and is induced by oxidative stresses (16,17). One such relevant process is H2O2-mediated cell death, occurring via either apoptosis or necrosis. Moderate H2O2 concentrations have been reported to trigger apoptosis, whereas relatively high H2O2 concentrations are known to induce necrotic cell death (18,19). Moreover, the mechanisms by which H2O2 induces cell death remain unclear.
The primary objective of the present study was to elucidate the mechanism of AICAR-induced apoptosis in human lymphoid (Jurkat) and myeloid (THP-1) cells. We found that AICAR treatment induces an increase in phosphorylation of AMPK-α1 and a decrease in intracellular ROS, and significantly induces apoptosis. Interestingly, AMPK-α1-knockdown THP-1 cells are particularly sensitive to apoptosis compared to control THP-1 cells, suggesting AMPK-independent apoptosis. Moreover, AICAR showed dual effects: low doses of AICAR induce their proliferation, whereas high doses suppress their proliferation. These effects are significantly correlated with the downregulation of intracellular ROS, strongly suggesting that AICAR-induced apoptosis is critically associated with the inhibition of NADPH oxidase by AICAR. Taken together, these results demonstrate that intracellular ROS levels regulated by AICAR are far more relevant in the AICAR-induced apoptosis than is AMPK activation.
The Jurkat and THP-1 cell lines were grown in RPMI 1640 medium (Life Technologies, Carlsbad, CA) supplemented with 1% antibiotics, 1% glutamine, and 10% fetal bovine serum. AMPK-αl-knockdown cells were cultured in the same medium containing puromycin (4~8 µg/ml).
Jurkat, THP1, AMPK-α1-knockdown cells (1×106) were treated with and without 500 µM AICAR for different times as indicated in each experiment, and were harvested and washed in 3 ml of cold PBS. The cells were then incubated for 120 min in complete darkness with 5 µl of propidium iodide (PI), 1 µl of Annexin V-FITC (BD, Franklin Lakes, NJ) and 10 µl of binding buffer (100 mM HEPES pH 7.4, 140 mM NaCl, 25 mM CaCl2). The controls were incubated with either binding buffer or unlabeled Annexin V. An additional 400 µl of binding buffer was added to each of the samples prior to FACScan analysis (FACSCalibur, BD). For cell cycle analysis, the cells were stained with a Cell Cycle Analysis Kit (BD) according to the manufacturer's instructions prior to analysis using the FACSCalibur.
The oxidation of 2',7'-dichlorodihydrofluorescein (DCFH) to fluorescent 2',7'-dichlorofluorescein (DCF: Molecular Probes, Inc.) was measured in order to determine the levels of intracellular H2O2. The cells were treated with or without different concentrations of AICAR for different times as indicated in each experiment. For DCF staining, DCFH was added at a final concentration of 20 µM, and incubated for 30 min at 37℃. The cells were washed once in PBS and maintained in 1 ml of medium. Cellular fluorescence was evaluated via flow cytometry (FACSCalibur, BD) using an emission window of 510~540 nm.
Jurkat and THP1 cells were treated with or without 5 mM AICAR (Toronto Research Chemicals, Inc) for different times, as indicated in each experiment. Cells were washed once with PBS and lysed in sample buffer (80~120 µl), and then immediately boiled for 5 min. Each of the samples was resolved in 10 or 12% SDS-PAGE, after which the proteins were electrotransferred onto a polyvinylidene difluoride (PVDF) membrane (GE Healthcare, Waukesha, WI). The membrane was then blocked with 5% nonfat dry milk in Tris-buffered saline with Tween 20 (TBS-T; 20 mM Tris-HCl pH 7.4, 8 g/L NaCl, and 0.1% Tween 20) for 1~2 h at room temperature. The membrane was then incubated overnight with anti-phospho-AMPKα (Thr172) in TBS-T containing 5% nonfat dry milk at 4℃. The membrane was washed three times in TBS-T and probed with horseradish peroxidase-conjugated secondary antibody for 1.5~2 h at room temperature. After four washings with TBS-T, the protein was visualized using an ECL Plus Western blotting detection system according to the manufacturer's instructions (GE Healthcare). For the apoptosis assay, Jurkat, THP-1, and AMPK-α1-knockdown cells were treated with 5 mM AICAR for different times, as indicated in the experiment. The protein samples were prepared, separated, and electrotransferred onto a PVDF membrane, according to the procedures described above. The membrane was incubated with anti-caspase-3 (Santa Cruz Biotech, Inc), anti-caspase-8 (Santa Cruz Biotech, Inc), and anti-caspase-9 (Santa Cruz Biotech, Inc) antibodies, and then probed with horseradish peroxidase-conjugated secondary antibody. The protein was visualized using the ECL Plus Western blotting detection system (Amersham ECL).
Jurkat, THP-1, and AMPK-α1-knockdown cells were treated with 5 mM AICAR for different times, as indicated in the experiment. Caspase-3, -8, and -9 activity assays were conducted using a Fluorometric Assay Kit (R&D Systems, Minneapolis, MN) according to the manufacturer's instructions. The level of caspase activity in the cell lysates was determined to be directly proportional to the fluorescence signal detected with a Fluoroskan Ascent fluorescence microplate reader (Labsystems, Vantaa, Finland).
Jurkat cells were treated with 5 mM AICAR for different times, as indicated in each experiment. DNA laddering assays were conducted using a TACS DNA Laddering Detection Kit (R&D Systems), according to the manufacturer's instructions. Each of the DNA samples was resolved in a 1.5% agarose gel, and then visualized under UV illumination after ethidium bromide staining (0.5 µg/ml). The gels were photographed using Polaroid 667 film (Sigma-Aldrich, St. Louis, MO).
For cell proliferation assays, Jurkat or THP-1 were plated in 96-well round-bottom plates (Corning Inc., Corning, NY) at 5×105 cells/well and cultured with or without different concentrations of AICAR for different times in an atmosphere of 5% CO2 at 37℃. The cells were pulsed with 1 µCi 3H-thymidine and harvested with a Tomcat Mach II harvester (Wallac, Turku, Finland) onto filter plates, which were read using a β-counter.
AICAR induces the activation of AMPK and acts as an inhibitor of NADPH oxidase (11). Apoptosis is thereby induced, presumably by AMPK activation, in different cell types (5,20,21). To investigate the role of AICAR in apoptosis in Jurkat (human T cell leukemia cell line) cells and THP1 (human acute monocytic leukemia cell line) cells, we first examined the known functions of AICAR. As had been expected, AICAR significantly induced AMPK phosphorylation at Thr172 in both cells (Fig. 1A, Jurkat; Fig. 1B, THP-1) According to AICAR treatment, moreover, intracellular ROS levels gradually decreased in a time-dependent manner (Fig. 1C, Jurkat; Fig. 1D, THP-1). We postulated that if AICAR does indeed induce apoptosis, then these effects are associated with AICAR-induced apoptosis. To address this hypothesis, we next determined the toxicity of AICAR in these cells. The cells were treated with different concentrations of AICAR for 24 h and a cell proliferation assay was performed. In both cells, treatment with AICAR markedly inhibited cell proliferation in a dose-dependent manner (Fig. 1E). The results revealed that treatment with 5 mM AICAR significantly inhibited cell proliferation in both cell types, by as much as 95%. Moreover, when treated with 10 mM AICAR, cell proliferation was markedly inhibited after 1 h of treatment, and the inhibitory effects showed a plateau after 9 h of treatment (Fig. 1F), demonstrating that AICAR markedly inhibits cell proliferation in a dose- and time-dependent manner, although the details of this mechanism are still unclear.
A recent report has shown that AICAR activates caspase-3 and caspase-9 in astrocytoma cells, which results in the induction of apoptosis (21). To confirm the induction of apoptosis following treatment with AICAR, we examined early and late apoptosis using an Annexin V-FITC and PI staining method. Jurkat and THP-1 cells were treated with 10 mM AICAR for different times and apoptosis was assessed. AICAR-induced early apoptosis, as shown by Annexin V-FITC-positive cells, was markedly apparent in Jurkat cells after 3 h (Fig. 2A). Moreover, AICAR-induced late apoptosis, as shown by Annexin V-FITC- and PI-positive cells, gradually increased until 24 h (Fig. 2A). However, a significant increase in necrotic cells, as would have been shown by Annexin V-FITC-negative and PI-positive cells, was not observed in Jurkat cells treated with 10 mM AICAR, indicating that treatment with AICAR specifically induces apoptosis rather than necrosis. This result in Jurkat cells was similar overall to that observed in THP-1 cells (Fig. 2B). Moreover, treatment with 10 mM AICAR in the Jurkat cells markedly induced activation of caspase-3, caspase-8, and caspase-9 (Fig. 2C), and their activities significantly increased in a time-dependent manner (Fig. 2E, caspase-3; Fig. 2F, caspase-9). In addition, similar results could be seen in the DNA laddering assay (Fig. 2D), suggesting that AICAR induced apoptosis in these cell.
We next checked whether the AICAR-induced apoptosis is dependent on the activation of AMPK. For this, we generated AMPK-α1-knockdown (AMPKKD) THP-1 cells as described in our previous report. After confirmation of the AMPK knockdown (SI: Fig. S1), wild type and AMPKKD THP-1 cells were assayed for apoptosis. Similar to the results in Fig. 2B, AICAR treatment significantly induced apoptosis, as detected by the increases in Annexin V-FITC-positive cells (Fig. 3A) and apoptotic populations (Fig. 3C), and degradation (Fig. 3E, wild type THP-1) and activities of caspase-3, -9, and -8 (closed circles in Fig. 3F, Fig. 3G, and Fig. 3H). Interestingly, AMPKKD THP-1 cells revealed that, according to the AICAR treatment, apoptotic markers such as Annexin V-FITC-positive cells (Fig. 3B), apoptotic populations (Fig. 3D), and caspase-3, -8, and -9 activities (Fig. 3E, AMPKKD THP-1 cells; open circles in Fig. 3F, Fig. 3G, and Fig. 3H) were markedly higher than those of wild type THP-1 cells. These results strongly suggest that AICAR-induced apoptosis might be independent of AMPK.
As shown in Fig. 1C and Fig. 1D, AICAR showed antioxidant activity that might result in the inhibition of NADPH oxidase, which is a key enzyme for regulating intracellular H2O2 levels (11). The increase in intracellular H2O2 levels caused by a variety of stimuli has multiple functions such as intracellular signaling, apoptosis, and necrotic cell death (12,13,14,15,16,17,18,19). In contrast, many previous reports have shown that antioxidants capable of downregulating intracellular ROS induce the cytotoxicity that is critically associated with apoptosis (22,23,24). Furthermore, previous reports have shown that the activation of AMPK is critically involved in anti-apoptotic effects through the activation of NF-κB or p38 MAPK-dependent pathways (25,26). Therefore, we hypothesized that if the AMPK activation by AICAR is not associated with apoptosis, then the antioxidant activity of AICAR may be associated with the cytotoxicity. Similar to previous reports, interestingly, Jurkat and THP-1 cells exhibited increases in cell proliferation at low concentrations of AICAR treatment (<1 mM), and these increases were significantly maintained until 16 h after treatment. Although cell proliferation transiently increased at high concentrations of AICAR (>2 mM), it gradually decreased in a time-dependent manner (Fig. 4A, Jurkat; Fig. 4B, THP-1). Furthermore, this phenomenon significantly correlated with intracellular ROS levels; AICAR treatment at low concentrations (<0.1 mM) did not induce the downregulation of intracellular ROS, whereas ROS levels dramatically decreased at high concentrations of AICAR (>5 mM) (Fig. 4C, Jurkat; Fig. 4D, THP-1). These data suggest that the AICAR-induced apoptosis might be related to its ability to induce the downregulation of intracellular ROS.
In summary, the results of the present study reveal that, at least in the Jurkat and THP1 cells, AICAR-induced apoptosis is independent of the activation of AMPK, as shown in Fig. 3. In contrast, we showed that the apoptosis is critically associated with the antioxidant activity induced by AICAR. As depicted in Fig. 4E, AICAR may have dual functions: AMPK activation and inhibition of NADPH oxidase. The activation of AMPK induced by AICAR may initially promote cellular proliferation at early time points (Fig. 4E, left). However, the inhibition of NADPH oxidase by AICAR induces the down-regulation of intracellular ROS levels, which induces cytotoxicity and ultimately apoptosis (Fig. 4E, right). We believe that our data might contribute to a better understanding of AICAR's effects when the drug is administered experimentally in vivo, as well as therapeutically as an antioxidant for treating oxidative stress-related diseases.
Figures and Tables
Figure 1
The bifunctional role of AICAR as an AMPK activator and NADPH oxidase inhibitor induces cytotoxicity in human lymphoid cells. (A and B) Jurkat (A) and THP-1 cells (B) were treated with or without 5 mM AICAR for the times indicated. Cell lysates were analyzed by immunoblot with antibodies specific for phospho-AMPK. The immunoblot was reprobed with an anti-GAPDH antibody. Data are representative of three independent experiments. (C and D) Jurkat (C) and THP-1 cells (D) were treated with or without 5 mM AICAR for the times indicated. Intracellular ROS concentrations were measured by DCF-DA staining. Data are the averages±SD of mean fluorescence intensity (MFI) obtained from three independent experiments. (E and F) Jurkat (closed circles) and THP-1 cells (open circles) were treated with different concentrations of AICAR for 24 h. A 3H-thymidine-incorporation assay was performed as described in Materials and Methods. The inhibitory effects of AICAR are expressed as relative cell viabilities, as calculated by cpm (AICAR-treated)/cpm (untreated) ×100. Data are the means±SD of the triplicate samples.
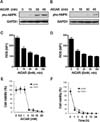
Figure 2
AICAR induces apoptosis in human lymphoid cells. (A and B) Jurkat (A) and THP-1 cells (B) were treated with 10 mM AICAR for the times indicated. Cells were stained with Annexin V-FITC and PI and analyzed by flow cytometry. Data are representative of three independent experiments. Early apoptotic cells (Annexin V-positive cells) and late apoptotic cells (Annexin V-positive and PI-positive cells) were analyzed by flow cytometry. Data are the means±SD of a percentage of cells obtained from three independent experiments. (C) Jurkat cells were treated with or without 10 mM AICAR for the times indicated. Cell lysates were analyzed by immunoblot with antibodies specific for caspase-3, caspase-8, and caspase-9. The immunoblot was reprobed with an anti-GAPDH antibody. Data are representative of three independent experiments. (D) Jurkat cells were treated with or without 10 mM AICAR for the times indicated and a DNA laddering assay was performed as described in Materials and Methods. (E and F) Jurkat cells were treated with or without 5 mM AICAR for the times indicated. Caspase-3 (E) and caspase-9 (F) activities were analyzed as described in Materials and Methods. Data are the means±SD of three independent experiments.
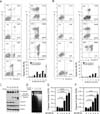
Figure 3
AMPK-α1-knockdown THP-1 cells are highly sensitive to treatment with AICAR. (A~D) Wild type (A and C) and AMPK-α1-knockdown THP-1 cells (B and D) were treated with 10 mM AICAR for the times indicated. Cells were stained with Annexin V-FITC and PI (A and B) or a cell cycle analysis kit (C and D), and then analyzed by flow cytometry. Data are representative of three independent experiments. Data are the means±SD of a percentage of cells obtained from three independent experiments. (E) Wild type and AMPK-α1-knockdown THP-1 cells were treated with or without 10 mM AICAR for the times indicated. Cell lysates were analyzed by immunoblot with antibodies specific for caspase-3, caspase-8, and caspase-9. The immunoblot was reprobed with an anti-GAPDH antibody. Data are representative of three independent experiments. (F~H) Wild type and AMPK-α1-knockdown THP-1 cells were treated with or without 10 mM AICAR for the times indicated. Caspase-3 (F), caspase-9 (G), and caspase-8 (H) activities were analyzed as described in Materials and Methods. Data are the means±SD obtained from three independent experiments.
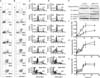
Figure 4
AICAR functions through the activation of AMPK or inhibition of NADPH oxidase in human lymphoid cells. (A and B) Jurkat (A) and THP-1 (B) cells were treated with or without different concentrations of AICAR for different times as indicated. A 3H-thymidine incorporation assay was performed as described in Materials and Methods. Data are the means±SD of the triplicate samples. (C and D) Jurkat (C) and THP-1 (D) cells were treated with or without different concentrations of AICAR for different times as indicated. DCF staining was performed as described in Materials and Methods, and flow cytometry analysis was performed. Data are the averages±SD of the mean fluorescence intensity in triplicate samples. (E) A model for the dual functions of AICAR: AMPK activation and NADPH oxidase inhibition. The activation of AMPK induced by AICAR may initially promote cellular proliferation at early time points (left). However, the inhibition of NADPH oxidase by AICAR induces the downregulation of intracellular ROS levels, which induces cytotoxicity and ultimately apoptosis (right).
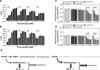
ACKNOWLEDGEMENTS
This work was supported by a grant from the Korea Healthcare Technology R&D Project, Ministry of Health and Welfare, Republic of Korea (A111636). Flow cytometry analysis was supported by Research Core Facility (RCF) in Sungkyunkwan University School of Medicine.
References
1. Hardie DG, Carling D, Carlson M. The AMP-activated/SNF1 protein kinase subfamily: metabolic sensors of the eukaryotic cell? Annu Rev Biochem. 1998; 67:821–855.


2. Kemp BE, Mitchelhill KI, Stapleton D, Michell BJ, Chen ZP, Witters LA. Dealing with energy demand: the AMP-activated protein kinase. Trends Biochem Sci. 1999; 24:22–25.


3. Hardie DG, Carling D. The AMP-activated protein kinase-fuel gauge of the mammalian cell? Eur J Biochem. 1997; 246:259–273.


4. Corton JM, Gillespie JG, Hawley SA, Hardie DG. 5-Aminoimidazole-4-carboxamide ribonucleoside. A specific method for activating AMP-activated protein kinase in intact cells? Eur J Biochem. 1995; 229:558–565.


5. Garcia-Gil M, Pesi R, Perna S, Allegrini S, Giannecchini M, Camici M, Tozzi MG. 5'-Aminoimidazole-4-carboxamide riboside induces apoptosis in human neuroblastoma cells. Neuroscience. 2003; 117:811–820.


6. Thomas CB, Meade JC, Holmes EW. Aminoimidazole carboxamide ribonucleoside toxicity: a model for study of pyrimidine starvation. J Cell Physiol. 1981; 107:335–344.


7. Sabina RL, Patterson D, Holmes EW. 5-Amino-4-imidazolecarboxamide riboside (Zriboside) metabolism in eukaryotic cells. J Biol Chem. 1985; 260:6107–6114.


8. Swain JL, Hines JJ, Sabina RL, Holmes EW. Accelerated repletion of ATP and GTP pools in postischemic canine myocardium using a precursor of purine de novo synthesis. Circ Res. 1982; 51:102–105.


9. Swain JL, Hines JJ, Sabina RL, Harbury OL, Holmes EW. Disruption of the purine nucleotide cycle by inhibition of adenylosuccinate lyase produces skeletal muscle dysfunction. J Clin Invest. 1984; 74:1422–1427.


10. Nath N, Giri S, Prasad R, Salem ML, Singh AK, Singh I. 5-Aminoimidazole-4-carboxamide ribonucleoside: a novel immunomodulator with therapeutic efficacy in experimental autoimmune encephalomyelitis. J Immunol. 2005; 175:566–574.


11. Alba G, El Bekay R, Alvarez-Maqueda M, Chacon P, Vega A, Monteseirin J, Santa Maria C, Pintado E, Bedoya FJ, Bartrons R, Sobrino F. Stimulators of AMP-activated protein kinase inhibit the respiratory burst in human neutrophils. FEBS Lett. 2004; 573:219–225.


12. Stone JR, Yang S. Hydrogen peroxide: a signaling messenger. Antioxid Redox Signal. 2006; 8:243–270.


13. Immenschuh S, Baumgart-Vogt E. Peroxiredoxins, oxidative stress, and cell proliferation. Antioxid Redox Signal. 2005; 7:768–777.


14. Gechev TS, Hille J. Hydrogen peroxide as a signal controlling plant programmed cell death. J Cell Biol. 2005; 168:17–20.


15. Saito Y, Nishio K, Ogawa Y, Kimata J, Kinumi T, Yoshida Y, Noguchi N, Niki E. Turning point in apoptosis/necrosis induced by hydrogen peroxide. Free Radic Res. 2006; 40:619–630.


16. Rhee SG, Bae YS, Lee SR, Kwon J. Hydrogen peroxide: a key messenger that modulates protein phosphorylation through cysteine oxidation. Sci STKE. 2000; (53):PE1.


18. Davies KJ. The broad spectrum of responses to oxidants in proliferating cells: a new paradigm for oxidative stress. IUBMB Life. 1999; 48:41–47.


19. Hampton MB, Orrenius S. Dual regulation of caspase activity by hydrogen peroxide: implications for apoptosis. FEBS Lett. 1997; 414:552–556.


20. Kefas BA, Cai Y, Ling Z, Heimberg H, Hue L, Pipeleers D, Van de Casteele M. AMP-activated protein kinase can induce apoptosis of insulin-producing MIN6 cells through stimulation of c-Jun-N-terminal kinase. J Mol Endocrinol. 2003; 30:151–161.


21. Meisse D, Van de Casteele M, Beauloye C, Hainault I, Kefas BA, Rider MH, Foufelle F, Hue L. Sustained activation of AMP-activated protein kinase induces c-Jun N-terminal kinase activation and apoptosis in liver cells. FEBS Lett. 2002; 526:38–42.


22. Busch C, Jacob C, Anwar A, Burkholz T, Aicha Ba L, Cerella C, Diederich M, Brandt W, Wessjohann L, Montenarh M. Diallylpolysulfides induce growth arrest and apoptosis. Int J Oncol. 2010; 36:743–749.


23. Li N, Ragheb K, Lawler G, Sturgis J, Rajwa B, Melendez JA, Robinson JP. DPI induces mitochondrial superoxide-mediated apoptosis. Free Radic Biol Med. 2003; 34:465–477.


24. Yasmeen A, Beauchamp MC, Piura E, Segal E, Pollak M, Gotlieb WH. Induction of apoptosis by metformin in epithelial ovarian cancer: involvement of the Bcl-2 family proteins. Gynecol Oncol. 2011; 121:492–498.


Supplementary Material
Figure S1
Generation of AMPK-α1 knockdown THP-1 cells. THP-1 cells were infected with lentiviral particles containing shRNA targeted human AMPK-a1 (sc-29673-V) or control lentiviral particles (sc-108080) according to the manufacturer's protocol. Control THP-1 and AMPK-α1 knockdown THP-1 (AMPK-α1KD THP-1 cells were cultured in puromycin-containing medium (4 µg/ml) for 2 weeks to select stable clones, and maintained in RPMI medium containing 4 µg/ml puromycin, 10% FBS, 2 mM L-glutamine, 100 U/ml of penicillin, 100 µg/ml streptomycin, and 5×10-5 M β-mercaptoethanol. Immunoblot (IB) with antibody to anti-AMPK-α1 or anti-GAPDH was performed to evaluate the knockdown efficacy.