Abstract
We examined the immunogenicity of H-2 class I-restricted and HLA-A2-restricted epitopes through peptide immunization of HLA-A2-transgenic mice that also express mouse H-2 class I molecules. All four of the tested epitopes restricted by H-2 class I robustly elicited T-cell responses, but four of seven epitopes restricted by HLA-A2 did not induce T-cell responses, showing that HLA-A2-restricted peptide epitopes tend to be poorly immunogenic in HLA-A2-transgenic mice. This finding was confirmed in HLA-A2-transgenic mice infected with a recombinant vaccinia virus expressing hepatitis C virus proteins. We examined the precursor frequency of epitope-specific naïve CD8+ T cells in HLA-A2-transgenic and conventional C57BL/6 mice and found that the poor immunogenicity of HLA-A2-restricted peptide epitopes is related to the paucity of naïve CD8+ T-cell precursors in HLA-A2-transgenic mice. These results provide direction for the improvement of mouse models to study epitope repertoires and the immunodominance of human T-cell responses.
T cells express TCRs for the recognition of peptide epitopes presented on MHC molecules. They acquire diverse TCRs during development via the rearrangement of TCR genes. In the thymus, T cells with TCRs that bind weakly to self-peptides presented on self-MHC molecules survive through a process called positive selection (1). Then, T cells with TCRs that bind strongly to peptide-MHC complexes in the thymus are eliminated through a process called negative selection. Once these selection processes are complete, mature CD4+ or CD8+ T cells are released to peripheral circulation (1).
CD8+ CTLs recognize endogenously processed peptides presented on MHC class I molecules. In particular, CTLs recognize peptides generated via the proteasomal cleavage of viral or tumor antigen proteins and therefore play a critical role in immune responses against virus-infected and tumor cells (2). When CTLs are activated by TCR stimulation, they exert cytotoxic activities through the degranulation of perforin and granzymes and secrete cytokines such as interferon (IFN)-γ and tumor necrosis factor α (2).
After viral infection or vaccination, CTL responses are predominantly directed to a small number of epitopes, although many potential epitopes are present in viral proteins (3). Therefore, there are immunodominant and subdominant epitopes that elicit large and small CTL responses, respectively, in the protein pool of a given virus. This hierarchy of CTL responses is reproducible and predictable in a genetically identical mouse strain (3). The immunodominance of CTL responses is known to be determined by the frequency of naïve CTL precursors with TCRs that recognize a given epitope (4). In fact, a high frequency of naïve CTL precursors often results in immunodominant CTL responses after viral infection (5,6).
Human MHC class I-expressing mice have been established to study human MHC class I-restricted CTL responses in mice. HLA-A2-transgenic mice were developed first and then improved upon because HLA-A2 is the most abundant MHC class I allotype in human beings (7,8,9). Other human MHC class I-transgenic mice have also been developed (10,11,12) and used for several purposes, including the identification of human CTL epitopes and acquisition of human CTL epitope-specific T cells. However, HLA-A2-restricted peptide epitopes were usually poorly immunogenic in HLA-A2-transgenic mice, and their immunogenicity was improved in H-2 class I knockout HLA-A2-transgenic mice (13,14,15).
In the present study, we used HLA-A2-transgenic mice that also express mouse H-2 class I molecules. We found that in many cases, HLA-A2-restricted peptide epitopes were much less immunogenic than H-2 class I-restricted epitopes. We also found that the poor immunogenicity of HLA-A2-restricted epitopes was associated with the paucity of epitope-specific, naïve CTL precursors in HLA-A2-transgenic mice.
We used HLA-A2-transgenic mice expressing AAD, which consists of the α1 and α2 domains of the HLA-A2.1 molecule and the α3 domain of the mouse H-2 Dd molecule, under the direction of the HLA-A2.1 promoter (Jackson Laboratory, Bar Harbor, ME, USA) (8,16). These mice expressed AAD in addition to mouse MHC H-2b. Conventional C57BL/6 mice without the HLA-A2 transgene were also used. Mice were kept in accordance with institutional guidelines and used at the age of 6 weeks for peptide immunization.
We used ovalbumin (OVA)257 (17), vaccinia virus (VV) A11R198 (18), Japanese encephalitis virus NS4B215 (19), and West Nile virus NS4B215 (19) peptides as H-2 class I-restricted peptide epitopes. We also used influenza A virus (IAV) M158 (20), IAV (PR8) NA231 (21), hepatitis C virus (HCV) core35 (22), HCV core132 (22), HCV (genotype 1b) NS31073 (21), HCV (genotype 1b) NS31406 (23), and Epstein-Barr virus BMLF1280 (20) peptides as HLA-A2-restricted peptide epitopes. Their sequences and MHC restrictions are summarized in Table I. Peptides were purchased from Peptron (Daejeon, Korea) and dissolved in 5% dimethyl sulfoxide phosphate-buffered saline.
Female mice were immunized three times subcutaneously in the hind footpad at 2-week intervals with a mixture of a peptide epitope (100µg), a helper epitope (50µg), lipopolysaccharide (5µg; Invivogen, San Diego, CA, USA), and CpG oligodeoxynucleotide 1826 (5µg; Genotech, Daejeon, Korea). The helper epitope was the H-2 I-Ab-restricted, hepatitis B virus core128 peptide (TPPAYRPPNAPIL) (24). Twelve days after the third immunization, the mice were killed, and splenocytes were prepared for direct ex vivo IFN-γ ELISPOT assays and MHC class I pentamer staining.
We used an rVV expressing HCV (genotype 1b) NS3, NS4A, and part of NS4B (amino acids 1007~1890 of the HCV polyprotein; rVV-HCVNS3-4B) (25). The rVV was derived from the WR strain. Female mice were challenged intraperitoneally with 107 plaque-forming units of rVV-HCVNS3-4B (25). Ten days later, the mice were killed, and splenocytes were prepared for direct ex vivo IFN-γ ELISPOT assays.
Direct ex vivo IFN-γ ELISPOT assays were performed as previously described (26). Triplicate cultures of 500,000 splenocytes were established in RPMI-1640 medium containing 10% fetal bovine serum and 2 mM L-glutamine. The splenocytes were stimulated with a peptide epitope (10µg/ml), and 5% dimethyl sulfoxide phosphate-buffered saline was used as a negative control. After 30 h of culture, IFN-γ spots developed. The spots were counted with an ELISPOT reader (CTL, Cleveland, OH, USA), and the number of specific spots was calculated by subtracting the mean number of spots in negative control wells from the mean number of spots in peptide-stimulated wells.
We used PE-conjugated HLA-A0201 pentamers loaded with IAV M158 or HCV core132 peptides (Proimmune, Oxford, UK). After peptide immunization, splenocytes were stained with ethidium monoazide and then with PE-conjugated HLA-A2 pentamer for 20 min at room temperature. Further staining was performed with fluorochrome-conjugated anti-CD3 (BD Biosciences, San Jose, CA, USA) and anti-CD8 (BD Biosciences). The stained cells were analyzed on an LSRII (BD Biosciences) using FACSDiva software (BD Biosciences) and FlowJo software (Tree Star, Ashland, OR, USA).
To enrich epitope-specific, naïve CTL precursors from the whole body of a mouse, we harvested the spleen and inguinal, cervical, mesenteric, periaortic, and axillary lymph nodes and prepared a single-cell suspension (27). The cells were stained with PE-conjugated H-2 Kb pentamer loaded with OVA257 peptide or HLA-A0201 pentamers loaded with IAV M158 or HCV core132 peptides (Proimmune) and further labeled with anti-PE magnetic microbeads (Miltenyi Biotec, Bergisch Gladbach, Germany). The microbead-labeled cell suspension was passed through an LS column (Miltenyi Biotec), and MHC class I pentamer-stained cells were enriched. The enriched cells were further stained with fluorochrome-conjugated anti-CD3, anti-CD8, and anti-CD44 (BD Biosciences) and analyzed on the LSRII using FACSDiva and FlowJo software. The frequency of naïve CTL precursors in the mouse was calculated by multiplying the number of cells in the enriched cell population by the percentage of MHC class I pentamer(+) cells (27).
To examine the immunogenicity of H-2 class I-restricted and HLA-A2-restricted peptide epitopes, we immunized HLA-A2-transgenic mice with one of these peptide epitopes and evaluated epitope-specific T-cell responses with direct ex vivo IFN-γ ELISPOT assays. For H-2 class I-restricted peptide epitopes, we used OVA257, VV A11R198, Japanese encephalitis virus NS4B215, and West Nile virus NS4B215 peptides (see Table I). All of these epitopes elicited robust T-cell responses in HLA-A2-transgenic mice (Fig. 1A). For HLA-A2-restricted peptide epitopes, we used IAV M158, IAV NA231, HCV core35, HCV core132, HCV NS31073, HCV NS31406, and Epstein-Barr virus BMLF1280 peptides (Table I). IAV M158, HCV core35, and HCV NS31406 elicited epitope-specific T-cell responses in HLA-A2-transgenic mice, but the other peptides did not (see Fig. 1A). We also performed MHC class I pentamer staining for HLA-A2-restricted peptide epitopes. HCV core132-specific CD8+ T cells were not detected in the spleen after peptide immunization, whereas IAV M158-specific CD8+ T cells were detectable (Fig. 1B), validating the results of the IFN-γ ELISPOT assays. Taken together, these results confirm previous reports that HLA-A2-restricted peptide epitopes tend to be poorly immunogenic in HLA-A2-transgenic mice (13,14,15).
We also compared the immunogenicity of H-2 class I-restricted and HLA-A2-restricted peptide epitopes in the context of viral infection. HLA-A2-transgenic mice were infected with rVV-HCVNS3-4B, and epitope-specific T-cell responses were assessed with direct ex vivo IFN-γ ELISPOT assays. After rVV-HCVNS3-4B infection, T-cell responses directed to HLA-A2-restricted HCV NS3 peptide epitopes such as HCV NS31073 and HCV NS31406 were scarcely induced, whereas H-2 Kb-restricted VV A11R198-specific T-cell response was vigorously elicited (Fig. 1C). This result shows that HLA-A2-restricted peptide epitopes are not immunodominant in HLA-A2-transgenic mice.
Next, we pursued the explanation for the poor immunogenicity of HLA-A2-restricted peptide epitopes in HLA-A2-transgenic mice by examining the precursor frequency of epitope-specific naïve CD8+ T cells. We isolated cells from the secondary lymphoid organs of the whole body of a mouse, stained them with MHC class I pentamer, and magnetically enriched the MHC class I pentamer(+) cells. After enrichment, CTL precursors recognizing the H-2 Kb-restricted OVA257 epitope were detected in the naïve CD8+ T-cell population of HLA-A2-transgenic mice (Fig. 2A). However, CTL precursors specific to the HLA-A2-restricted HCV core132 epitope were not detected in these mice (see Fig. 2A). In the case of the HLA-A2-restricted IAV M158 epitope that elicited T-cell responses (see Fig. 1A and B), epitope-specific CTL precursors were detected in the naïve CD8+ T-cell population of HLA-A2-transgenic mice (see Fig. 2A). We also calculated the frequency of naïve CTL precursors specific to each peptide in an HLA-A2-transgenic mouse (see Fig. 2A). Taken together, these results led us to conclude that the frequency of naïve CTL precursors is correlated with CTL immunogenicity and the immunodominance of epitopes and that the poor immunogenicity of HLA-A2-restricted peptide epitopes is related to the paucity of naïve CTL precursors in HLA-A2-transgenic mice.
We also examined naïve CTL precursors in conventional C57BL/6 mice without the HLA-A2 transgene. As in HLA-A2-transgenic mice, OVA257-specific naïve CTL precursors were detected and HCV core132-specific naïve CTL precursors were not detected in C57BL/6 mice (Fig. 2B). However, IAV M158-specific naïve CTL precursors were not detected in C57BL/6 mice, whereas they were detected in HLA-A2-transgenic mice (see Fig. 2B). These results suggest that IAV M158-specific naïve CTL precursors survive through positive selection in the thymus of HLA-A2-transgenic mice but not in that of C57BL/6 mice. However, the positive selection of HCV core132-specific naïve CTL precursors is not influenced by HLA-A2 expression in the thymus. In this case, the paucity of naïve CTL precursors might be caused by differences in germline TCR genes between humans and mice; there is a hole for TCRs recognizing HCV core132 in the TCR repertoire of C57BL/6-background mice.
Since their establishment, HLA-A2-transgenic mouse strains have been useful tools for the identification of HLA-A2-restricted peptide epitopes within viral or tumor antigen proteins. However, HLA-A2-restricted peptide epitopes tend to be poorly immunogenic in HLA-A2-transgenic mice (13,14,15), although one report has shown an extensive overlap between the TCR repertoires of HLA-A2-transgenic mice and human CTLs (24). In the current study, we demonstrate the poor immunogenicity of several HLA-A2-restricted peptide epitopes in HLA-A2-transgenic mice. To improve the immunogenicity of HLA-A2-restricted peptide epitopes in HLA-A2-transgenic mice, H-2 class I was knocked out in HLA-A2-transgenic mice (13). In fact, HLA-A2-restricted CTL responses in H-2 class I knockout HLA-A2-transgenic mice better resemble human CTL responses (14,15).
Although we examined only HLA-A2-transgenic mice in the present study, we expect similar results in mice expressing other HLA class I allotypes (10,11,12). HLA class II-transgenic mice have also been used for immunological studies (28,29,30,31). Examinations of the immunogenicity of HLA class II-restricted peptide epitopes in relation to the frequency of naïve CD4+ T cell precursors are warranted in HLA class II-transgenic mice, as performed in the current study.
Given the results of the present study, we suggest that differences in germline TCR genes between humans and mice might cause the paucity of naïve CTL precursors recognizing HLA-A2-restricted epitopes in HLA-A2-transgenic mice based on the frequency of HCV core132-specific naïve CD8+ T cells (see Fig. 2A and B). Therefore, there might be holes for TCRs recognizing certain HLA-A2-restricted epitopes in the mouse TCR repertoire. If this is the case, the immunogenicity of HLA-A2-restricted peptide epitopes in HLA-A2-transgenic mice could be improved by the introduction of the human TCR gene loci into HLA-A2-transgenic mice. Then, CTL immunodominance patterns in HLA-A2-transgenic mice would better reflect those in HLA-A2(+) humans. Indeed, mice transgenic for human TCR gene loci have been established, and they have a diverse human TCR repertoire (32). In this way, the T-cell immune system of a mouse model will better resemble that of humans, improving these tools for the study of epitope repertoires and the immunodominance of human T-cell responses.
ACKNOWLEDGEMENTS
This work was supported by the National Agenda Project grant from Korea Research Council of Fundamental Science and Technology (NTM1311423), the Korea Research Institute for Bioscience and Biotechnology (KRIBB) Initiative program (KGM3121423), and a grant of the Korean Health Technology R&D Project, Ministry of Health and Welfare, Korea (HI13C1263). This work was also supported by National Research Foundation grants (2012-M3C1A1-048860) and the Korea Advanced Institute of Science and Technology (KAIST) Future Systems Healthcare Project funded by the Ministry of Science, ICT and Future Planning of Korea.
References
1. Spits H. Development of alphabeta T cells in the human thymus. Nat Rev Immunol. 2002; 2:760–772. PMID: 12360214.
2. Barry M, Bleackley RC. Cytotoxic T lymphocytes: all roads lead to death. Nat Rev Immunol. 2002; 2:401–409. PMID: 12093006.


3. Yewdell JW, Bennink JR. Immunodominance in major histocompatibility complex class I-restricted T lymphocyte responses. Annu Rev Immunol. 1999; 17:51–88. PMID: 10358753.


4. Jenkins MK, Moon JJ. The role of naive T cell precursor frequency and recruitment in dictating immune response magnitude. J Immunol. 2012; 188:4135–4140. PMID: 22517866.


5. Kotturi MF, Scott I, Wolfe T, Peters B, Sidney J, Cheroutre H, von Herrath MG, Buchmeier MJ, Grey H, Sette A. Naive precursor frequencies and MHC binding rather than the degree of epitope diversity shape CD8+ T cell immunodominance. J Immunol. 2008; 181:2124–2133. PMID: 18641351.
6. Obar JJ, Khanna KM, Lefrançois L. Endogenous naive CD8+ T cell precursor frequency regulates primary and memory responses to infection. Immunity. 2008; 28:859–869. PMID: 18499487.
7. Epstein H, Hardy R, May JS, Johnson MH, Holmes N. Expression and function of HLA-A2.1 in transgenic mice. Eur J Immunol. 1989; 19:1575–1583. PMID: 2676561.


8. Le AX, Bernhard EJ, Holterman MJ, Strub S, Parham P, Lacy E, Engelhard VH. Cytotoxic T cell responses in HLA-A2.1 transgenic mice. Recognition of HLA alloantigens and utilization of HLA-A2.1 as a restriction element. J Immunol. 1989; 142:1366–1371. PMID: 2464645.
9. Vitiello A, Marchesini D, Furze J, Sherman LA, Chesnut RW. Analysis of the HLA-restricted influenza-specific cytotoxic T lymphocyte response in transgenic mice carrying a chimeric human-mouse class I major histocompatibility complex. J Exp Med. 1991; 173:1007–1015. PMID: 1706750.


10. Nickerson CL, Hanson J, David CS. Expression of HLA-B27 in transgenic mice is dependent on the mouse H-2D genes. J Exp Med. 1990; 172:1255–1261. PMID: 2212952.


11. Alexander J, Oseroff C, Sidney J, Wentworth P, Keogh E, Hermanson G, Chisari FV, Kubo RT, Grey HM, Sette A. Derivation of HLA-A11/Kb transgenic mice: functional CTL repertoire and recognition of human A11-restricted CTL epitopes. J Immunol. 1997; 159:4753–4761. PMID: 9366399.
12. Alexander J, Oseroff C, Sidney J, Sette A. Derivation of HLA-B*0702 transgenic mice: functional CTL repertoire and recognition of human B*0702-restricted CTL epitopes. Hum Immunol. 2003; 64:211–223. PMID: 12559623.


13. Pascolo S, Bervas N, Ure JM, Smith AG, Lemonnier FA, Pérarnau B. HLA-A2.1-restricted education and cytolytic activity of CD8(+) T lymphocytes from beta2 microglobulin (beta2m) HLA-A2.1 monochain transgenic H-2Db beta2m double knockout mice. J Exp Med. 1997; 185:2043–2051. PMID: 9182675.
14. Firat H, Garcia-Pons F, Tourdot S, Pascolo S, Scardino A, Garcia Z, Michel ML, Jack RW, Jung G, Kosmatopoulos K, Mateo L, Suhrbier A, Lemonnier FA, Langlade-Demoyen P. H-2 class I knockout, HLA-A2.1-transgenic mice: a versatile animal model for preclinical evaluation of antitumor immunotherapeutic strategies. Eur J Immunol. 1999; 29:3112–3121. PMID: 10540322.


15. Firat H, Cochet M, Rohrlich PS, Garcia-Pons F, Darche S, Danos O, Lemonnier FA, Langlade-Demoyen P. Comparative analysis of the CD8(+) T cell repertoires of H-2 class I wild-type/HLA-A2.1 and H-2 class I knockout/HLA-A2.1 transgenic mice. Int Immunol. 2002; 14:925–934. PMID: 12147629.


16. Newberg MH, Smith DH, Haertel SB, Vining DR, Lacy E, Engelhard VH. Importance of MHC class 1 alpha2 and alpha3 domains in the recognition of self and non-self MHC molecules. J Immunol. 1996; 156:2473–2480. PMID: 8786307.
17. Martin S, Ortmann B, Pflugfelder U, Birsner U, Weltzien HU. Role of hapten-anchoring peptides in defining hapten-epitopes for MHC-restricted cytotoxic T cells. Cross-reactive TNP-determinants on different peptides. J Immunol. 1992; 149:2569–2575. PMID: 1383319.
18. Kim SK, Cornberg M, Wang XZ, Chen HD, Selin LK, Welsh RM. Private specificities of CD8 T cell responses control patterns of heterologous immunity. J Exp Med. 2005; 201:523–533. PMID: 15710651.


19. Trobaugh DW, Yang L, Ennis FA, Green S. Altered effector functions of virus-specific and virus cross-reactive CD8+ T cells in mice immunized with related flaviviruses. Eur J Immunol. 2010; 40:1315–1327. PMID: 20213733.
20. Clute SC, Watkin LB, Cornberg M, Naumov YN, Sullivan JL, Luzuriaga K, Welsh RM, Selin LK. Cross-reactive influenza virus-specific CD8+ T cells contribute to lymphoproliferation in Epstein-Barr virus-associated infectious mononucleosis. J Clin Invest. 2005; 115:3602–3612. PMID: 16308574.
21. Wedemeyer H, Mizukoshi E, Davis AR, Bennink JR, Rehermann B. Cross-reactivity between hepatitis C virus and Influenza A virus determinant-specific cytotoxic T cells. J Virol. 2001; 75:11392–11400. PMID: 11689620.


22. Wedemeyer H, He XS, Nascimbeni M, Davis AR, Greenberg HB, Hoofnagle JH, Liang TJ, Alter H, Rehermann B. Impaired effector function of hepatitis C virus-specific CD8+ T cells in chronic hepatitis C virus infection. J Immunol. 2002; 169:3447–3458. PMID: 12218168.
23. Thimme R, Oldach D, Chang KM, Steiger C, Ray SC, Chisari FV. Determinants of viral clearance and persistence during acute hepatitis C virus infection. J Exp Med. 2001; 194:1395–1406. PMID: 11714747.


24. Wentworth PA, Vitiello A, Sidney J, Keogh E, Chesnut RW, Grey H, Sette A. Differences and similarities in the A2.1-restricted cytotoxic T cell repertoire in humans and human leukocyte antigen-transgenic mice. Eur J Immunol. 1996; 26:97–101. PMID: 8566090.


25. Wedemeyer H, Gagneten S, Davis A, Bartenschlager R, Feinstone S, Rehermann B. Oral immunization with HCV-NS3-transformed Salmonella: induction of HCV-specific CTL in a transgenic mouse model. Gastroenterology. 2001; 121:1158–1166. PMID: 11677208.


26. Lee DH, Kim SH, Kang W, Choi YS, Lee SH, Lee SR, You S, Lee HK, Chang KT, Shin EC. Adjuvant effect of bacterial outer membrane vesicles with penta-acylated lipopolysaccharide on antigen-specific T cell priming. Vaccine. 2011; 29:8293–8301. PMID: 21893142.


27. Moon JJ, Chu HH, Pepper M, McSorley SJ, Jameson SC, Kedl RM, Jenkins MK. Naive CD4(+) T cell frequency varies for different epitopes and predicts repertoire diversity and response magnitude. Immunity. 2007; 27:203–213. PMID: 17707129.


28. Altmann DM, Douek DC, Frater AJ, Hetherington CM, Inoko H, Elliott JI. The T cell response of HLA-DR transgenic mice to human myelin basic protein and other antigens in the presence and absence of human CD4. J Exp Med. 1995; 181:867–875. PMID: 7532684.


29. Nabozny GH, Baisch JM, Cheng S, Cosgrove D, Griffiths MM, Luthra HS, David CS. HLA-DQ8 transgenic mice are highly susceptible to collagen-induced arthritis: a novel model for human polyarthritis. J Exp Med. 1996; 183:27–37. PMID: 8551230.


30. Rosloniec EF, Brand DD, Myers LK, Esaki Y, Whittington KB, Zaller DM, Woods A, Stuart JM, Kang AH. Induction of autoimmune arthritis in HLA-DR4 (DRB1*0401) transgenic mice by immunization with human and bovine type II collagen. J Immunol. 1998; 160:2573–2578. PMID: 9510154.
31. Mix H, Weiler-Normann C, Thimme R, Ahlenstiel G, Shin EC, Herkel J, David CS, Lohse AW, Rehermann B. Identification of CD4 T-cell epitopes in soluble liver antigen/liver pancreas autoantigen in autoimmune hepatitis. Gastroenterology. 2008; 135:2107–2118. PMID: 18773898.


32. Li LP, Lampert JC, Chen X, Leitao C, Popovic J, Müller W, Blankenstein T. Transgenic mice with a diverse human T cell antigen receptor repertoire. Nat Med. 2010; 16:1029–1034. PMID: 20693993.


Figure 1
Immunogenicity of H-2 class I-restricted and HLA-A2-restricted peptide epitopes in HLA-A2-transgenic mice. (A) HLA-A2-transgenic mice were immunized with peptide epitopes, and epitope-specific T-cell responses were evaluated with direct ex vivo interferon gamma (IFN-γ) ELISPOT assays with splenocytes as described in Materials and Methods (n=3-5). (B) HLA-A2-transgenic mice were immunized with peptide epitopes, and MHC class I pentamer staining was performed with splenocytes as described in Materials and Methods. (C) HLA-A2-transgenic mice were infected with recombinant vaccinia virus-hepatitis C virus (rVV-HCV)NS3-4B, and epitope-specific T-cell responses were evaluated with direct ex vivo IFN-γ ELISPOT assays with splenocytes as described in Materials and Methods (n=5; **p<0.01).
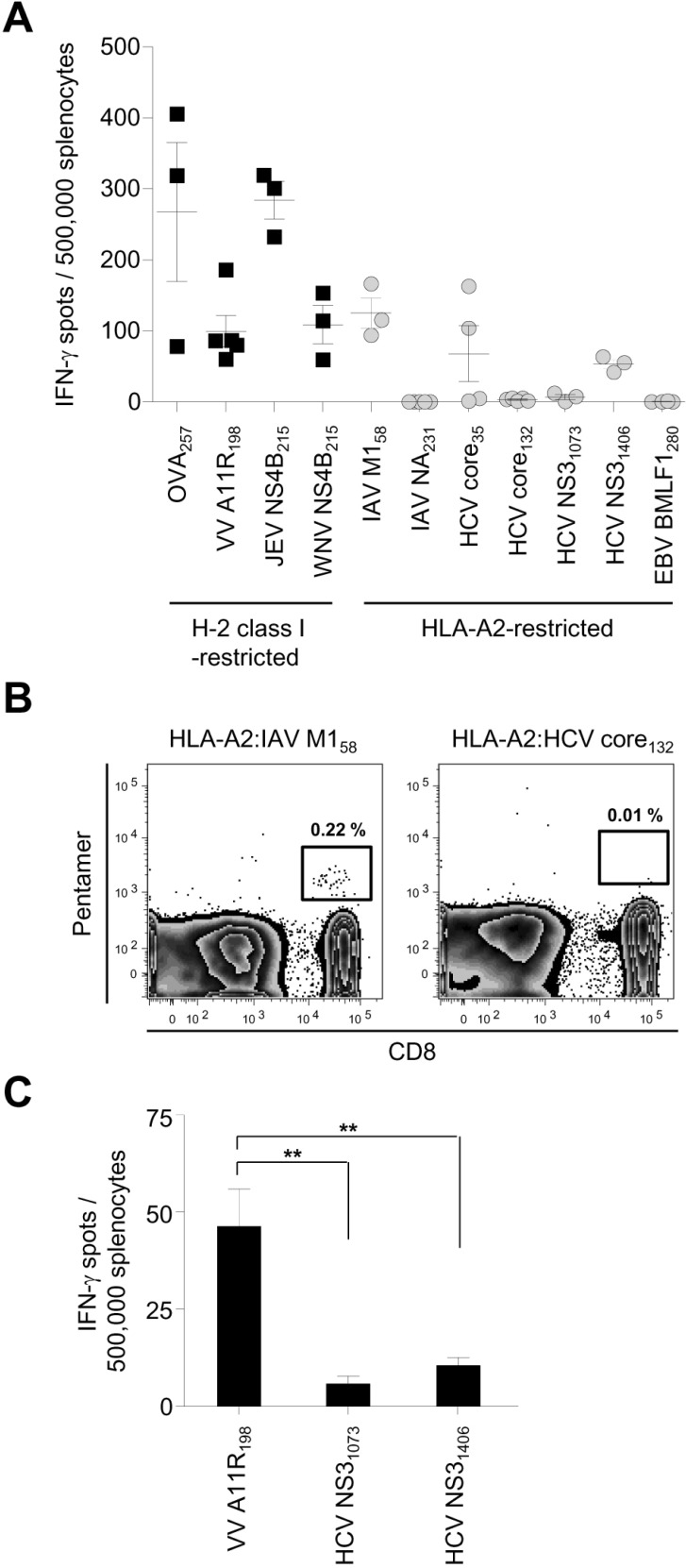
Figure 2
Precursor frequency of epitope-specific naïve CD8+ T cells. Cells were isolated from the secondary lymphoid organs of the whole body of an HLA-A2-transgenic (A) or C57BL/6 (B) mouse and stained with MHC class I pentamer. MHC class I pentamer(+) cells were magnetically enriched and detected with flow cytometry. The precursor frequency of epitope-specific naïve CD8+ T cells was calculated as described in Materials and Methods.
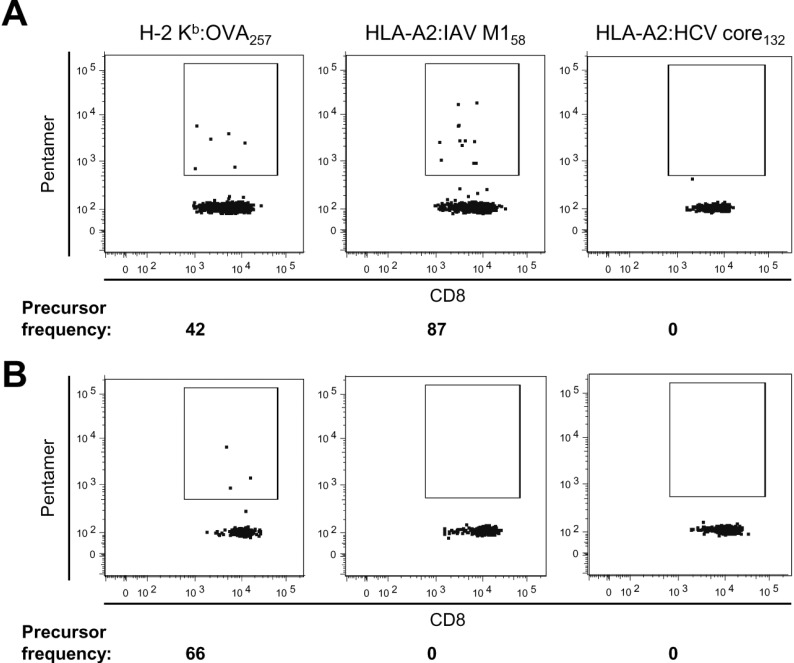