Abstract
In the present study, we investigated if priming of autoreactive CD8+ T cells would be inhibited by competitive peptides for major histocompatibility complex (MHC) class I binding. We used a mouse model of vitiligo which is induced by immunization of Kb-binding tyrosinase-related protein 2 (TRP2)-180 peptide. Competitive peptides for Kb binding inhibited IFN-γ production and proliferation of TRP2-180-specific CD8+ T cells upon ex vivo peptide restimulation, while other MHC class I-binding peptides did not. In mice, the capability of inhibition was influenced by T-cell immunogenicity of the competitive peptides. The competitive peptide with a high T-cell immunogenicity efficiently inhibited priming of TRP2-180-specific CD8+ T cells in vivo, whereas the competitive peptide with a low T-cell immunogenicity did not. Taken together, the inhibition of priming of autoreactive CD8+ T cells depends on not only competition of peptides for MHC class I binding but also competitive peptide-specific CD8+ T cells, suggesting that clonal expansion of autoreactive T cells would be affected by expansion of competitive peptide-specific T cells. This result provides new insights into the development of competitive peptides-based therapy for the treatment of autoimmune diseases.
Immune responses against self-antigens caused by failure of self-tolerance develop autoimmune diseases which affect approximately 7~9% of the general population (1). However, current treatments of autoimmune diseases are not curative and induce general immunosuppression which is associated with serious infection and other adverse outcomes (2). Therefore, it is necessary to develop new therapeutics in order to selectively suppress autoreactive immune cells, including B cells, CD4+ T cells, and CD8+ T cells.
Unlike B cells, T cells recognize epitope peptides presented by major histocompatibility complex (MHC) molecules. Binding of T-cell epitope peptides to MHC is determined by hydrophobic or charge interactions between the residue of peptides and the cleft of MHC molecules (3,4). Thus, peptides presented on MHC molecules can be replaced extracellularly by other peptides depending on their affinity to MHC molecules (5,6). If peptides with high affinity to MHC molecules are present extracellularly in excess, they would occupy the cleft of MHC molecules and replace preexisting peptides on MHC molecules. This concept might be exploited for the treatment of autoimmune diseases. Autoimmune responses induced by self peptide-specific T cells could be inhibited by the addition of competitive peptides that replace self peptides on MHC molecules and inhibit presentation of the self peptide on antigen presenting cells (APCs).
In the present study, we investigated if priming of autoreactive CD8+ T cells would be inhibited by competitive peptides for MHC class I binding. We used a mouse model of vitiligo which is induced by immunization of Kb-binding tyrosinase-related protein 2 (TRP2)-180 peptide. Previously, we established a novel mouse model of vitiligo by immunization with TRP2-180 peptide in combination with lipopolysaccharides (LPS) and CpG oligodeoxynucleotides (CpG ODN) (7). This mouse model of vitiligo was characterized by progressive depigmentation of the skin due to selective loss of melanocytes. In addition, the extent of skin depigmentation correlated with the frequency of TRP2-180-specific CD8+ effector T cells in the spleen. Therefore, we used this mouse model of vitiligo to analyze how efficiently the competitive peptides for MHC class I binding inhibit priming and activation of melanocyte-specific autoreactive CD8+ T cells.
In this study, competitive peptides for Kb binding inhibited IFN-γ production and proliferation of TRP2-180-specific CD8+ T cells upon ex vivo peptide restimulation. Interestingly, the capability of inhibition was influenced by T-cell immunogenicity of the competitive peptides in vivo. The competitive peptide with a high T-cell immunogenicity efficiently inhibited priming of TRP2-180-specific CD8+ T cells in vivo, whereas the competitive peptide with a low T-cell immunogenicity did not. Taken together, the inhibition of the priming of autoreactive CD8+ T cells depends on not only competition of peptides for MHC class I binding but also competitive peptide-specific CD8+ T cells. Thus, highly T cell-immunogenic peptides which are competitive for MHC binding might be useful for inhibiting priming of autoreactive T cells for the treatment of autoimmune diseases.
Male C57BL/6 mice were kept in accordance with the institutional guidelines, and used at the age of 4~5 weeks for peptide immunization. Peptides were purchased from Peptron (Daejeon, Korea) and dissolved in 5% DMSO phosphate-buffered solution (PBS).
Splenocytes of naive C57BL/6 were incubated with different combinations of peptides, including SIINFEKL at 0.5µM and various concentrations of additional peptides (0.5, 5, or 50µM) as indicated, at 37℃ for 4 hrs. After incubation, splenocytes were washed and fixed by 4% paraformaldehyde. The density of SIINFEKL-binding Kb molecules on lymphocytes was analyzed by flow cytometry using 25-D1.16 monoclonal antibody (8) that reacts with SIINFEKL-binding Kb molecule. The percentage of decreased density of SIINFEKL-binding Kb molecules by additional peptides was calculated as follows: [100-mean fluorescence index (MFI) of SIINFEKL-binding Kb in SIINFEKL at 0.5µM with additional peptides at 5µM/MFI of SIINFEKL-binding Kb in SIINFEKL at 0.5µM×100].
C57BL/6 mice were subcutaneously immunized twice at 1-week intervals in the footpad with TRP2-180 (50µg), LPS (5µg), and CpG ODN (5µg). One week after the second immunization, splenocytes were harvested and stimulated with different combinations of peptides, including TRP2-180 at 0.1µM and various doses of additional peptides (0, 0.1, 1, or 10µM). TRP2-180-specific IFN-γ production was analyzed by flow cytometry after intracellular cytokine staining (ICS) as described in the section of 'Intracellulr Cytokine Staining'.
CD45.2 C57BL/6 mice were subcutaneously immunized 3 times at 1-week intervals in the hind footpad with TRP2-180 (50µg), LPS (5µg) and CpG ODN (5µg). One week after the last immunization, splenocytes were harvested, and splenic CD8+ T cells were sorted using anti-CD8 microbeads (Miltenyi Biotec, Auburn, CA). These sorted CD8+ T cells were restimulated in vitro 3 times at 2-week intervals with TRP2-180 peptide (10µg/ml) in the presence of IL-2 (20 IU/ml) and CD45.1 splenocytes as APCs. The purity of TRP2-180-specific CD8+ T cells was evaluated by TRP2-180-specific IFN-γ production and was >80%. Two weeks after the last stimulation, CD8+ T cells were used for proliferation analysis.
CD45.2+, TRP2-180-specific CD8+ T cell line (0.2×106 cells) labeled with CFSE at 10µM (Invitrogen Ltd, Paisley, UK) was cocultured with CD45.1+ splenocytes (1×106 cells) pulsed with different combinations of peptides, including TRP2-180 at 0.1µM and additional peptides at 10µM. Four days after coculture, TRP2-180-specifc proliferation of CD8+ T cells was evaluated by fluorescence intensity of CFSE in flow cytometry analysis. TRP2-180-specific CD8+ T cells were distinguished from antigen presenting splenocytes by CD45.2 gating (anti-CD45.2-APC-H7, BD Biosciences, San Jose, CA, USA), and dead cells were excluded using 7-Amino-Acinomycin D (7-AAD, BD Biosciences). The percentage of proliferation inhibition by additional peptides was calculated as follows: [100-(% of proliferation in TRP2-180 at 0.1µM with additional peptides-% of proliferation in the absence of TRP2-180)/(% of proliferation in TRP2-180 at 0.1µM-% of proliferation in the absence of TRP2-180)×100].
C57BL/6 mice were subcutaneously immunized in the footpad with TRP2-180 (5 nmole), LPS (5µg), and CpG ODN (5µg). One day after the immunization, additional competitive peptides (50 nmole) were injected into the same footpad without adjuvants twice at 1-day intervals. One week after TRP2-180 immunization, splenocytes were harvested and stimulated with TRP-180 or competitive peptides, and peptide-specific IFN-γ+ CD8+ T cell population was analyzed by flow cytometry after ICS.
Vitiligo was induced by TRP2-180 immunization in mice as described previously (7). Briefly, C57BL/6 mice were subcutaneously immunized in the footpad with TRP2-180 (5 nmole), LPS (5µg) and CpG ODN (5µg) twice at 1-week intervals. One week after the second immunization, TRP2-180 (5 nmole) with the same adjuvants was injected into the tail dermis for disease induction twice at 1-week intervals. In this model, disease progression was evaluated with or without administration of competitive peptides. Competitive peptides (50 nmole) were injected into the tail dermis 1 day after third and fourth TRP2-180 immunization without adjuvants twice at 1-day intervals. Five weeks after the last TRP2-180 immunization, splenocytes were harvested and stimulated with TRP-180 or competitive peptides. Peptide-specific IFN-γ+ CD8+ T-cell population was analyzed by flow cytometry after ICS. In addition, the area of depigmented skin per tail was calculated by using the ImageJ program (National Institutes of Health, Bethesda, MD, USA) and presented as the percentage of depigmented skin per tail.
Splenocytes were resuspended at 107 cells/ml in RPMI 1640 containing 1% FBS, and 200µl of the suspension was added per well. Single cell suspensions were stimulated with TRP2-180 (5µg/ml) or additional competitive peptides (5µg/ml), and brefeldin A (GolgiPlug, BD Biosciences) was added 1 hr later. After another 5 hrs of incubation at 37℃, cells were stained with ethidium monoazide (Sigma-Aldrich, St. Louis, MO, USA). After washing, the cells were stained with anti-CD3-pacific blue, anti-CD4-APC-Cy7, anti-CD8-PE-Cy7, and anti-CD44-PE (all from BD Biosciences), permeabilized using a Cytofix/Cytoperm kit (BD Biosciences), and stained with anti-IFN-γ-APC (BD Biosciences). Stained cells were analyzed on an LSR flow cytometer (BD Biosciences).
In the present study, Kb-binding TRP2-180 peptide was used as a self peptide to induce melanocyte-specific CD8+ T cells and develop vitiligo (Table I) (7,9). For competition with TRP2-180 for Kb binding, we included vaccinia virus A11R 198-205 (VV A11R-198) (10), lymphocytic choriomeningitis virus GP 118-125 (LCMV GP-118) (10), and β-actin 219-227 (β-actin-219) (11) which bind to Kb. We also included Japanese encephalitis virus NS4B 215-223 (JEV NS4B-215) (12) which bind to Db, and Epstein-Barr virus BMLF1 280-288 (EBV BMLF1-280) (13) which bind to human leukocyte antigen (HLA)-A0201, as non-competitive peptides for Kb binding.
First, we evaluated relative Kb-binding affinity of the peptides by a competition assay using SIINFEKL peptide. In this assay, splenocytes were pulsed with SIINFEKL peptide in combination with competitive peptides, then the density of SIINFEKL-binding Kb molecules was quantified by flow cytometry using antibody specific to SIINFEKL-binding Kb molecule. Without competitive peptides, SIINFEKL peptide pulsing increased the density of SIINFEKL-binding Kb molecule in a dose-dependent manner (Fig. 1A). When splenocytes were pulsed with a fixed concentration (0.5µM) of SIINFEKL peptide in combination with increased concentration of additional peptides, Kb-binding peptides (TRP2-180, VV A11R-198, LCMV GP-118, and β-actin-219) decreased the density of SIINFEKL-binding Kb molecules in a dose-dependent manner (Fig. 1A). In particular, VV A11R-198, LCMV GP-118, and β-actin-219 more decreased the density of SIINFEKL-binding Kb molecules than TRP2-180 (Fig. 1A), indicating that they have a higher affinity for Kb than TRP2-180. Non-Kb-binding peptides (JEV NS4B-215 and EBV BMLF1-280) did not decrease the density of SIINFEKL-binding Kb molecules (Fig. 1A), confirming that they do not bind to Kb. By calculating the percentage of decreased density of SIINFEKL-binding Kb molecules, the relative Kb-binding affinity of peptide was determined (Fig. 1B).
To evaluate the inhibition of TRP2-180-specific IFN-γ production by competitive peptides, splenocytes of TRP2-180-immunized mice were stimulated ex vivo with TRP2-180 peptide alone or in combination with competitive peptides, and IFN-γ ICS was performed. IFN-γ production was observed in CD44+, antigen-experienced CD8+ T cells. As expected from the relative Kb-binding affinity of each peptide, VV A11R-198, LCMV GP-118, and β-actin-219 significantly inhibited IFN-γ production of TRP2-180-specific T cells in a dose-dependent manner, while JEV NS4B-215 and EBV BMLF1-280 did not (Fig. 2A and B).
To examine the inhibition of T cells proliferation by competitive peptides in vitro, a TRP2-180-specific T-cell line was established, and their proliferation responding to TRP2-180 peptide was evaluated by CFSE labeling. Proliferation of the T-cell line was increased by stimulation with TRP2-180 peptide (Fig. 3A). VV A11R-198, LCMV GP-118, and β-actin-219 inhibited TRP2-180-specific proliferation of T cells, while JEV NS4B-215 and EBV BMLF1-280 did not (Fig. 3B). The percentage of proliferation inhibition was calculated and presented for each peptide (Fig. 3C).
Next, we determined if Kb-binding competitive peptides would inhibit priming of TRP2-180-specific T cells in vivo. C57BL/6 mice were subcutaneously immunized in the footpad with TRP2-180, LPS, and CpG ODN. One day after the immunization, doses 10-times higher than competitive peptides were injected into the same footpad without adjuvants twice at 1-day intervals. One week after TRP2-180 immunization, splenocytes were harvested and TRP2-180-specific IFN-γ+ CD8+ T cells were analyzed by ICS and flow cytometry. Competitive peptide-specific IFN-γ+ CD8+ T cells were also analyzed. Non-Kb-binding peptides (JEV NS4B-215 and EBV BMLF1-280) did not decrease the frequency of TRP2-180-specific IFN-γ+ CD8+ T cells as expected (Fig. 4A). Among the Kb-binding peptides, only VV A11R-198 significantly decreased the frequency of TRP2-180-specific IFN-γ+ CD8+ T cells (Fig. 4A). LCMV GP-118 showed a tendency to decrease the frequency of TRP2-180-specific IFN-γ+ CD8+ T cells (Fig. 4A), while β-actin-219 did not (Fig. 4A). Interestingly, the ability of Kb-binding peptides to inhibit TRP2-180-specific T-cell priming was associated with T-cell immunogenicity of each peptide. VV A11R-198 which significantly inhibited TRP2-180-specific T-cell priming showed the highest T-cell immunogenicity (Fig. 4B), and β-actin-219 which did not inhibit the priming did not show T-cell immunogenicity (Fig. 4B). The data suggest that inhibition of priming of autoreactive CD8+ T cells depends on not only the competition of peptides for MHC class I binding but also competitive peptide-specific CD8+ T cells.
C57BL/6 mice were subcutaneously immunized in the footpad with TRP2-180, LPS, and CpG ODN twice at 1-week intervals. One week after the second immunization, TRP2-180 with the same adjuvants was injected into the tail dermis for disease induction twice at 1-week intervals. Competitive peptides were injected into the tail dermis 1 day after the third and fourth TRP2-180 immunization without adjuvants twice at 1-day intervals. Five weeks after the last TRP2-180 immunization, splenocytes were harvested and TRP2-180-specific IFN-γ+ CD8+ T cells were analyzed by ICS and flow cytometry. Competitive peptide-specific IFN-γ+ CD8+ T cells were also analyzed. In this case, no peptide decreased the frequency of TRP2-180-specific IFN-γ+ CD8+ T cells, while the 2 Kb-binding peptides (VV A11R-198 and LCMV GP-118) were immunogenic (Fig. 5A and B). As expected from the fact that no peptide decreased the frequency of TRP2-180-specific IFN-γ+ CD8+ T cells, disease progression evaluated by the percentage of depigmented skin lesions in a tail was not prevented by any peptide (Fig. 5C). VV A11R-198 showed a tendency to decrease the area of depigmented lesions in a tail, without statistical significance (Fig. 5C).
In the present study, we employed competitive peptides for Kb binding to inhibit activation of TRP2-180-specific CD8+ T cells, melanocyte-specific autoreactive CD8+ T cells. The competitive peptides displayed a higher Kb-binding affinity than TRP2-180 peptide, an autoimmunogenic peptide (Fig. 1A and B) and inhibited IFN-γ production and proliferation of TRP2-180-specific CD8+ T cells in vitro, while non-Kb-binding peptides did not (Fig. 2 and 3). The data demonstrate that the inhibition of TRP2-180-specific CD8+ T-cell activation in vitro depends on competition of peptides for MHC binding.
When we tested if Kb-binding competitive peptides inhibit priming of TRP2-180-specific CD8+ T cells, only VV A11R-198 significantly inhibited the priming (Fig. 4A), while β-actin-219 did not (Fig. 4A). Interestingly, the ability of Kb-binding peptides to inhibit TRP2-180-specific T-cell priming was associated with T-cell immunogenicity of each peptide. In fact, VV A11R-198 showed the highest immunogenicity to T cells (Fig. 4B), and β-actin-219 did not show any immunogenicity (Fig. 4B). These results suggest that clonal expansion of autoreactive T cells might be affected by expansion of competitive peptide-specific T cells. These findings are reminiscent of clonal competition in immunodominance and immune hierarchy in CD8+ T-cell responses (14). T cells primed by highly immunogenic peptides compete with autoreactive T cells for resources required for T-cell survival and proliferation (15). Therefore, we should consider not only competition of therapeutic peptides for MHC class I binding but also T-cell immunogenicity of therapeutic peptides in competitive peptides-based therapy for the treatment of autoimmune diseases.
Although VV A11R-198 inhibited the priming of TRP2-180-specific CD8+ T cells (Fig. 4A), it did not inhibit the boosting of them (Fig. 5A), and thus disease progression was not prevented by VV A11R-198 in a mouse model of vitiligo (Fig. 5C). The results demonstrate that VV A11R-198 could inhibit priming of naive T cells in vivo but could not inhibit reactivation of memory T cells in the tail skin. Since memory T cells rapidly proliferated and efficiently differentiated to effector T cells compared with naive T cells (16,17), our results could be explained by the difference between memory T cells and naive T cells. If competitive therapeutic peptides also target memory T cells, autoreactive memory T cells might be inhibited by competitive peptides. In this context, T-cell epitope peptides from influenza A virus or cytomegalovirus will be candidates for competitive peptides-based therapy because the majority of human population have a memory T-cell pool against these viruses (18,19).
In summary, competitive peptides for Kb binding inhibited IFN-γ production and proliferation of TRP2-180-specific CD8+ T cell in vitro. In mice, inhibition of TRP2-180-specific CD8+ T-cell priming required competitive peptides with a high immunogenicity for T cells. Although competitive peptides did not prevent the progression of the depigmented skin lesion in a mouse model of vitiligo, our results provide new insights into the development of competitive peptides-based therapy for the treatment of autoimmune diseases.
Figures and Tables
Figure 1
Relative affinity of T cell epitope peptides for Kb binding. Splenocytes of naive C57BL/6 mice were incubated with peptides. Peptide-pulsed splenocytes were fixed with 4% paraformaldehyde, and the density of SIINFEKL-binding Kb molecules on lymphocytes was analyzed by flow cytometry using antibody specific to SIINFEKL-binding Kb molecule (clone 25-D1.16). (A) A dashed line represents the mean fluorescence intensity (MFI) of 25-D1.16-stained lymphocytes pulsed with the indicated concentration of SIINFEKL without additional peptides. Each solid line represents MFI of 25-D1.16-stained lymphocytes pulsed with SIINFEKL at 0.5µM in combination with the indicated concentration of additional peptide. Each dot represents the mean+SEM from 3 independent experiments. (B) The percentage of decreased MFI of SIINFEKL-binding Kb molecules by additional peptides (SIINFEKL at 0.5µM and additional peptide at 5µM) was calculated as follows: [100-MFI of SIINFEKL-binding Kb in SIINFEKL at 0.5µM with additional peptides at 5µM/MFI of SIINFEKL-binding Kb in SIINFEKL at 0.5µM×100]. Each bar graph represents the mean+SEM from 3 independent experiments.
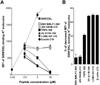
Figure 2
Inhibition of TRP2-180-specific IFN-γ production of CD8+ T cells by competitive peptides for Kb binding in vitro. C57BL/6 mice were immunized with TRP2-180, and splenocytes were harvested and stimulated ex vivo with TRP2-180 peptide alone or in combination with competitive peptides. Then, IFN-γ ICS was performed and analyzed by flow cytometry. (A) The splenocytes were stimulated ex vivo with 0.1µM of TRP2-180 peptide alone or in combination with competitive peptides at 10µM. Representative FACS dot plots for IFN-γ and CD44 are shown in the gate of the CD8+ T cell population. (B) The splenocytes were stimulated ex vivo with TRP2-180 peptide at 0.1µM alone or in combination with the indicated concentration of competitive peptides, and the percentages of TRP2-180-specific IFN-γ+ cells in the CD8+ T-cell population are presented as bar graphs. Each bar graph represents the mean+SEM from 6 independent experiments (**p<0.01).
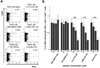
Figure 3
Inhibition of TRP2-180-specific CD8+ T-cell proliferation by competitive peptides for Kb binding in vitro. A TRP2-180-specific T-cell line (CD45.2) labeled with CFSE at 10µM was cocultured with splenocytes (CD45.1) pulsed with TRP2-180 peptide alone or in combination with competitive peptides. Four days after coculture, cocultured cells were stained with anti-CD45.2-APC-H7 and analyzed by flow cytometry. (A) CFSE-labeled T-cell line was cocultured with splenocytes pulsed with the indicated concentration of TRP2-180. The fluorescence intensity of CFSE was analyzed in the gate of the CD45.2+-cell population. (B) CFSE labeled T cell line was cocultured with splenocytes pulsed with TRP2-180 at 0.1µM in combination with additional peptides at 10µM. The fluorescence intensity of CFSE was analyzed in the gate of the CD45.2+-cell population. (C) The percentage of proliferation inhibition by additional peptide was calculated as follows: [100-(% of proliferation in TRP2-180 at 0.1µM with additional peptides-% of proliferation in the absence of TRP2-180)/(% of proliferation in TRP2-180 at 0.1µM-% of proliferation in the absence of TRP2-180)×100], and presented by bar graphs.
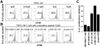
Figure 4
Inhibition of TRP2-180-specific CD8+ T-cell priming by competitive peptides in vivo. C57BL/6 mice were subcutaneously immunized in the footpad with TRP2-180 (5 nmole), LPS, and CpG ODN. One day after the immunization, competitive peptides at 50 nmole were injected into the same footpad without adjuvants twice at 1-day intervals. One week after TRP2-180 immunization, splenocytes were harvested. (A) TRP2-180-specific IFN-γ+ CD8+ T cells were analyzed by ICS and flow cytometry in each group. (B) Competitive peptide-specific IFN-γ+ CD8+ T cells were analyzed by ICS and flow cytometry in each group. Each bar graph represents the mean+SEM from 3 independent experiments with 3 mice per group (*p<0.05).
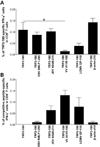
Figure 5
Competitive peptides do not prevent progression of depigmented skin lesions in a mouse model of vitiligo. C57BL/6 mice were subcutaneously immunized in the footpad with TRP2-180 (5 nmole), LPS, and CpG ODN twice at 1-week intervals. One week after the second immunization, TRP2-180 (5 nmole) with the same adjuvants was injected into the tail dermis for the disease induction twice at 1-week intervals. Fifty nmole competitive peptides were injected into the tail dermis 1 day after third and fourth TRP2-180 immunization without adjuvants twice at 1-day intervals. Five weeks after the last TRP2-180 immunization, splenocytes were harvested. (A) TRP2-180-specific IFN-γ+ CD8+ T cells were analyzed by ICS and flow cytometry in each group. (B) Competitive peptide-specific IFN-γ+ CD8+ T cells were analyzed by ICS and flow cytometry in each group. (C) Disease progression was evaluated by the percentage of depigmented skin lesions in the tail in each group. Each bar graph represents the mean+SEM from 3 independent experiments with 3 mice per group (n.s., not significant).
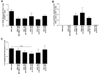
ACKNOWLEDGEMENTS
This work was supported by National Research Foundation grants funded by the Korea government (Ministry of Education, Science and Technology) (2012-M3C1A1-048860).
References
1. Cooper GS, Bynum ML, Somers EC. Recent insights in the epidemiology of autoimmune diseases: improved prevalence estimates and understanding of clustering of diseases. J Autoimmun. 2009; 33:197–207.


2. Lee E, Sinha AA. T cell targeted immunotherapy for autoimmune disease. Autoimmunity. 2005; 38:577–596.


3. Bjorkman PJ, Saper MA, Samraoui B, Bennett WS, Strominger JL, Wiley DC. Structure of the human class I histocompatibility antigen, HLA-A2. Nature. 1987; 329:506–512.


4. Bjorkman PJ, Saper MA, Samraoui B, Bennett WS, Strominger JL, Wiley DC. The foreign antigen binding site and T cell recognition regions of class I histocompatibility antigens. Nature. 1987; 329:512–518.


5. Babbitt BP, Matsueda G, Haber E, Unanue ER, Allen PM. Antigenic competition at the level of peptide-Ia binding. Proc Natl Acad Sci U S A. 1986; 83:4509–4513.


6. Adorini L, Muller S, Cardinaux F, Lehmann PV, Falcioni F, Nagy ZA. In vivo competition between self peptides and foreign antigens in T-cell activation. Nature. 1988; 334:623–625.


7. You S, Cho YH, Byun JS, Shin EC. Melanocyte-specific CD8+ T cells are associated with epidermal depigmentation in a novel mouse model of vitiligo. Clin Exp Immunol. 2013; [Epub ahead of print].


8. Porgador A, Yewdell JW, Deng Y, Bennink JR, Germain RN. Localization, quantitation, and in situ detection of specific peptide-MHC class I complexes using a monoclonal antibody. Immunity. 1997; 6:715–726.


9. Bloom MB, Perry-Lalley D, Robbins PF, Li Y, el-Gamil M, Rosenberg SA, Yang JC. Identification of tyrosinase-related protein 2 as a tumor rejection antigen for the B16 melanoma. J Exp Med. 1997; 185:453–459.


10. Kim SK, Cornberg M, Wang XZ, Chen HD, Selin LK, Welsh RM. Private specificities of CD8 T cell responses control patterns of heterologous immunity. J Exp Med. 2005; 201:523–533.


11. Karunakaran KP, Rey-Ladino J, Stoynov N, Berg K, Shen C, Jiang X, Gabel BR, Yu H, Foster LJ, Brunham RC. Immunoproteomic discovery of novel T cell antigens from the obligate intracellular pathogen Chlamydia. J Immunol. 2008; 180:2459–2465.


12. Trobaugh DW, Yang L, Ennis FA, Green S. Altered effector functions of virus-specific and virus cross-reactive CD8+ T cells in mice immunized with related flaviviruses. Eur J Immunol. 2010; 40:1315–1327.


13. Clute SC, Watkin LB, Cornberg M, Naumov YN, Sullivan JL, Luzuriaga K, Welsh RM, Selin LK. Cross-reactive influenza virus-specific CD8+ T cells contribute to lymphoproliferation in Epstein-Barr virus-associated infectious mononucleosis. J Clin Invest. 2005; 115:3602–3612.


14. Yewdell JW, Bennink JR. Immunodominance in major histocompatibility complex class I-restricted T lymphocyte responses. Annu Rev Immunol. 1999; 17:51–88.


15. Savir Y, Waysbort N, Antebi YE, Tlusty T, Friedman N. Balancing speed and accuracy of polyclonal T cell activation: a role for extracellular feedback. BMC Syst Biol. 2012; 6:111.


16. Curtsinger JM, Lins DC, Mescher MF. CD8+ memory T cells (CD44high, Ly-6C+) are more sensitive than naive cells to (CD44low, Ly-6C-) to TCR/CD8 signaling in response to antigen. J Immunol. 1998; 160:3236–3243.
17. Veiga-Fernandes H, Walter U, Bourgeois C, McLean A, Rocha B. Response of naïve and memory CD8+ T cells to antigen stimulation in vivo. Nat Immunol. 2000; 1:47–53.

