Abstract
Swiprosin-1 exhibits the highest expression in CD8+ T cells and immature B cells and has been proposed to play a role in lymphocyte biology through actin remodeling. However, regulation of swiprosin-1 gene expression is poorly understood. Here we report that swiprosin-1 is up-regulated in T cells by PKC pathway. Targeted inhibition of the specific protein kinase C (PKC) isotypes by siRNA revealed that PKC-θ is involved in the expression of swiprosin-1 in the human T cells. In contrast, down-regulation of swiprosin-1 by A23187 or ionomycin suggests that calcium-signaling plays a negative role. Interestingly, swiprosin-1 expression is only reduced by treatment with NF-κB inhibitors but not by NF-AT inhibitor, suggesting that the NF-κB pathway is critical for regulation of swiprosin-1 expression. Collectively, these results suggest that swiprosin-1 is a PKC-θ-inducible gene and that it may modulate the late phase of T cell activation after antigen challenge.
Swiprosin-1 was first identified in human lymphocytes, predominantly in CD8+ lymphocytes (1) and later in immature B cells, resting and activated B cells, and non-lymphoid tissue, especially in the brain (2). Recently, we reported that swiprosin-1 is also expressed in mast cells and modulates mast cell activation through actin remodeling (3). Database mining revealed that swiprosin-1 putatively contains four myristoylation sites, three binding sites for SH3 domain containing proteins, two potential EF-hand domains, and a coiled-coil domain at the C-terminus, and therefore, may have a role as a small adaptor protein involved in calcium signaling (2). In accordance with this, swiprosin-1 was implicated in phosphotyrosine-based signaling events that are involved in the cellular stimulation of early growth factor (EGF) and in actin rearrangement (4).
However, the functions of swiprosin-1 are not well understood. Swiprosin-1 is known to be associated with lipid rafts in the immature B-cell line WEHI231 and it participates in enhancement of BCR signals and contributes to BCR-induced apoptosis (2,5). Actin binding proteins that regulate dynamic rearrangements of actin cytoskeleton are associated with various functions of immune cells (6). Recently, we found that swiprosin-1 is highly accumulated in actin cytoskeleton-rich regions and modulates mast cell activation through actin remodeling (3). Swiprosin-1 is also found in the cytoskeleton fraction in NK-like cells with actin and actin-binding proteins such as α-actinin, plastin, and filamin (7), suggesting that swiprosin-1 may regulate cell activation through actin remodeling.
It was interesting to note that swiprosin-1 expression is up-regulated both in vitro cultured mast cells by phorbol ester or cross-linking of FcεR1 and in vivo model tissues of passive cutaneous anaphylaxis and atopic dermatitis (8). The results strongly demonstrate that swiprosin-1 potentially acts as a regulator for cytokine expression and activation of mast cells. Although swiprosin-1 is also expressed in T cells, no evidence has been reported yet whether swiprosin-1 expression is regulated in T cells and it plays a role for T cell function.
In this study, we examined whether the expression of swiprosin-1 is regulated in T cells. A variety of pharmacologic agents and small interfering RNAs (siRNA) were employed to specifically determine which intracellular signaling pathways are involved in regulation of swiprosin-1 expression in T cells. It has been known that PKC is an important regulator for T cell activation (9,10). Accordingly, it has been noticed that PKC is a drug target for prevention of T cell-mediated autoimmunity and allograft rejection (11,12). In the current study, interestingly, we found that swiprosin-1 expression in T cells is up-regulated by treatment with phorbol ester, we primarily examined the involvement of specific PKC isotypes in swiprosin-1 expression in T cells.
Goat polyclonal antibody to swiprosin-1 was from Imgenex (San Diego, CA). Antibodies to protein kinase C (PKC)-α, PKC-βI, PKC-η, PKC-ζ, actin, and I-κBα were from Santa Cruz Biotechnology, Inc (Santa Cruz, CA). Antibodies to PKC-δ, and PKC-θ were from Cell Signaling Technology, Inc (Beverly, MA). Antibody to human CD3 (OKT3) was purified from hybridomas ATCC CRL-8001. Anti-human CD28 antibody was purchased from R&D Systems, Inc. (Minneapolis, MN). HRP-conjugated anti-goat, anti-rabbit, and anti-mouse IgGs were from GE Healthcare (Chalfont St. Giles, United Kingdom). Phorbol 12-myristate 13-acetate (PMA), A23187, phytohemagglutinin A (PHA), BAPTA-AM, ionomycin, SB203580, PD098059, SP600125, caffeic acid phenethyl ester (CAPE), and cyclosporine A (CsA) were purchased from Sigma Chemical Co (St. Louis, MO). Gö6983, Gö6976, rottlerin, and staurosporine were purchased from Calbiochem-Behring (La Jolla, CA). Total RNA isolation reagent was from WelPrep™ Join Bio Innovation (Daegu, South Korea). Maxime RT Premix (oligo dT primer), Maxime PCR PreMix, and a plasmid purification kit were from iNtRON Biotechnology (Daejon, South Korea). SYBR premix Ex Taq was from Takara Bio Inc (Shiga, Japan). The dual-luciferase reporter assay system was from Promega Corporation (Madison, WI). Small interfering RNA (siRNA) targeting PKC isotypes and a scrambled siRNA were obtained as a pool of four or more siRNA duplexes from Dharmacon (Chicago, IL).
Jurkat T cells (ATCC TIB-152, Manassas, VA) were maintained in RPMI 1640 medium (GIBCO, Gaitherburg, MD) supplemented with 10% (v/v) FBS (GIBCO, Invitrogen). Exponentially growing cells were seeded at 0.5-2×106 per six-well plate, and used for various experimental purposes. After written informed consent, human primary PBLs were isolated from healthy donors by dextran sedimentation and centrifugation through a discontinuous Ficoll gradient (Amersham Biosciences, Piscataway, NJ). The cell lines and human PBLs mentioned above were cultured at 37℃ in a humidified incubator containing 5% CO2 and 95% air. All experiments using human PBLs were approved by Ethics Committee of the School of Life Sciences, GIST.
Jurkat T cells (1.5×106) or human primary PBLs were stimulated with either plate-bound anti-CD3 (OKT3 for human, 10 µg/ml)/CD28 (2 µg/ml), phytohemagglutinin A (PHA) and/or PMA (200 nM)/A23187 (1 µM). In some case, the cells were pretreated for 30 min with the various reagents that modulate intracellular signalings.
Cells from the in vitro cultures were harvested and total RNA was isolated using the WelPrep™ JBI method (iNtRON Biotechnology, Daejon, Korea) according to the manufacturer's instructions. Reverse transcription of the RNA was performed using oligo dT primer Maxime RT-PCR PreMix (iNtRON Biotechnology, Daejon, Korea). Two micrograms of RNA was transferred to an oligo dT primer mixture tube. The reaction volume was 20 µl. cDNA synthesis was performed at 45℃ for 60 min, followed by RT inactivation at 95℃ for 5 min. Thereafter, the RT-generated DNA was diluted to 40 µl volume with distilled water. The diluted RT-generated DNA (2 µl) was amplified using Maxime PCR PreMix (iNtRON Biotechnology, Daejon, Korea). The primers used for cDNA amplification were as follows: Swip-1, sense 5'-ATCTTCCGCAAGGCGGCGGCCGGGGAG-3' and antisense 5'-GACTGCAGCTCCTTGAAGGCCGCTTTC-3'; hIL-2, 5'-CACGTCTTGCACTTGTCAC-3' and antisense 5'-CCTTCTTGGGCATGTAAAACT-3'; hIL-3, sense 5'-CTTTGCCTTTGCTGGACTTC-3' and antisense 5'-CGAGGCTCAAAGTCGTCTG-3'; GAPDH, sense 5'-CGGAGTCAACGGATTTGGTCGTAT-3' and antisense 5'-AGCCTTCTCCATGGTGGTGAAGAC-3'. Amplification conditions were denaturation at 94℃ for 30 s, annealing at 58~68℃ for 20 s, and extension at 72℃ for 40 s for 30~35 cycles. The PCR products were resolved and visualized on a 1 or 1.5% agarose gel and stained with ethidium bromide.
In all the experiments, the expression levels of the examined genes were evaluated by real-time RT-PCR, unless otherwise indicated. PCR amplification was performed in DNA Engine Opticon for a continuous fluorescence detection system (MJ Research, Waltham, MA) in a total volume of 20 µl containing 2 µl of cDNA/control and gene specific primers using the SYBR premix Ex Taq kit (Takara, Shiga, Japan). The PCR was performed under the following conditions: 94℃ for 30 s, 58 ~68℃ for 30 s, 72℃ for 30 s, plate read (detection of fluorescent product) for 40 cycles, followed by 7 min of extension at 72℃. A melting curve analysis was done to characterize the dsDNA product by slowly raising the temperature (0.2℃/s) from 65℃ to 95℃ with fluorescence data collected at 0.2℃ intervals. The levels of expression (of swiprosin-1, IL-3, and IL-8) that were normalized by GAPDH were expressed as a relative value (i.e., percentage) of the maximum. The result of the maximum level in each experiment was considered as 100%.
For the analysis of swiprosin-1, and PKC isoforms (including PKC-α PKC-βI, PKC-θ, PKC-δ, PKC-η, and PKC-ζ. Jurkat T cells were rinsed twice with ice-cold PBS and then lysed in ice-cold lysis buffer (10 mM Tris-HCl, pH 7.4, containing 50 mM NaCl, 1% Triton X-100, and a protease inhibitor cocktail tablet). Cell lysates were centrifuged at 14,000 rpm for 20 min at 4℃ and equal amounts of protein supernatant were mixed with a one-fourth volume of 4X SDS sample buffer, boiled for 5 min, and then separated through an 8 or 10% sodium dodecyl sulfate-polyacrylamide gel. After electrophoresis, proteins were transferred to a nitrocellulose membrane by means of the Trans-Blot SD semidry transfer cell (Bio-Rad, Hercules, CA). The membrane was blocked in 5% skim milk (1 h), rinsed, and incubated overnight at 4℃ with primary antibodies in TBS containing 0.1% Tween 20 (TBS-T) and 3% skim milk. Excess primary Ab was then removed by washing the membrane three times in TBS-T, and the membrane was incubated with 0.1 µg/ml horseradish peroxidase-labeled secondary Ab (against goat, rabbit, or mouse) for 2 h. Following three washes in TBS-T, bands were visualized by ECL western blotting detection reagents and exposed to x-ray film.
Jurkat T cells (1.5×106) were transfected with 100 µl of Amaxa's Nucleofector solution (Amaxa, Cologne, Germany) containing 1 µg of swiprosin-1/pEGFP-C1 or pEGFP-C1 with 1 µg of pRL-TK renilla and 1 µg of pGL3/NF-κB or pGL3/NF-AT, and then the cells were immediately transferred to 2.0 ml of complete medium and cultured in 6-well plates at 37℃. After 24 h of transfection, the medium was replaced with IMDM medium containing 10% FBS and antibiotics. Cells were treated with reagents as described above and incubated at 37℃ for 12 h. Cell lysates were prepared and assayed for luciferase activity using the Dual Luciferase Assay System (Promega, Madison, WI), according to the manufacturer's instructions.
The mean values were calculated from data taken from at least three (usually four or more) separate experiments conducted on separate days. Where significance testing was performed, an independent t test (Student's; two populations) was used. p-value of less than 0.05 was considered an indicator of statistical significance.
We first examined whether the level of swiprosin-1 is regulated in human T cells. To this end, Jurkat T cells were stimulated with anti-CD3/28 antibodies, and then the expression of swiprosin-1 and IL-2 was determined by RT-PCR. As shown in Fig. 1A, swiprosin-1 mRNA was significantly over-induced in Jurkat T cells and the maximum level was reached at the 6 h of stimulation. As a control, IL-2 expression was assessed (Fig. 1A). The up-regulation of swiprosin-1 expression was also achieved by treatment of cells with other stimuli such as PMA, PHA, PMA+A23187, and PMA+PHA+A23187 (Fig. 1B). Interestingly, however, we found that the up-regulation of swiprosin-1 is independent from the activation status of T cells as single PMA treatment significantly up-regulated swiprosin-1 mRNA expression while it did not induce cytokine expression including IL-2 and IL-3 (Fig. 1B). On the contrary, full activation of T cells by PMA+A23187 or PMA+PHA+A23187 did not significantly induce swiprosin-1 expression (Fig. 1B). Western blot analysis also revealed that single PMA treatment up-regulates the levels of swiprosin-1 protein in a time-dependent manner (Fig. 1C), suggesting that PKC may involve in the pathway of swiprosin-1 expression in T cells.
Interestingly, we found that single treatment with calcium ionophore A23187 had no effect on the expression of swiprosin-1 (Fig. 2A) in Jurkat T cells. This result led us to next examine whether PMA-induced swiprosin-1 expression is modulated by calcium signals. To this end, Jurkat T cells were pretreated with calcium modulators such as BAPTA (calcium blocker), A23187 (calcium ionophore), and ionomycin (calcium ionophore). Interestingly, the expression of swiprosin-1 was significantly reduced by treatment with A23187 or ionomycin but not by BAPTA, suggesting that calcium signal acts as a negative signal for swiprosin-1 expression in T cells (Fig. 2A, left). The effect of A23187 was also confirmed by Western blot analysis (Fig. 2A, right). We also tested the involvement of other signaling modules such as MAP kinase pathway. However, the expression of swiprosin-1 was not changed by treatment with any of three MAP kinase inhibitors (SB203580, p38 kinase inhibitor; PD098059, ERK inhibitor; SP600125, JNK inhibitor) (Fig. 2B).
As PKCs are primary targets for PMA, we next examined which PKC-isoforms could be responsible for swiprosin-1 expression in Jurkat T cells. Initially, we used pharmacologic agents that can modulate specific PKC isoforms. To this end, Jurkat T cells were pretreated with various concentrations of diverse PKC inhibitors, and then the cells were stimulated with PMA. As shown in Fig. 2C, Gö6976 (inhibitor of PKCα and PKCβI) and rottlerin (inhibitor of PKCδ, and PKCι) revealed no significant effects on PMA-induced swiprosin-1 expression. Gö6983 (inhibitor of PKCs α, βI, βII, δ and ζ) showed medium blocking effect. In contrast, staurosporine, a non-specific PKC inhibitor, significantly blocked PMA-induced swiprosin-1 expression (Fig. 2C). The data to this point demonstrated a requirement for PKC(s) in PMA-induced swiprosin-1 expression, but did not establish which PKC isoform (s) is (are) specifically involved in this process. We therefore used siRNAs to knockdown target PKC isoforms. Western blot analysis revealed that Jurkat T cells express PKCα, β, η, ζ, θ and δ forms (Fig. 3A) and that transfection of siRNA significantly down-regulated each target PKC in a time-dependent manner, showing maximal inhibition at 72 h of transfection (Fig. 3B). Among the six PKC isoforms examined, targeted knockdown of PKC-θ significantly inhibited PMA-induced swiprosin-1 expression (Fig. 3C), suggesting that PKC-θ plays a major function in regulating swiprosin-1 expression. Knockdown of PKC-θ also significantly reduced anti-CD3/28-induced swiprosin-1 up-regulation in both Jurkat T cells (Fig. 4A) and human peripheral blood lymphocytes (Fig. 4B).
Since a central event in T cell activation is the pathway involving the nuclear factors (13), we therefore tested whether swiprosin-1 induction is regulated by the transcriptional activities of the NF-κB and/or NF-AT. Both NF-κB and NF-AT promoter activities were highly induced in response to the PMA/A23187 (Fig. 5A). PMA significantly induced NF-κB promoter activity while it has no effect on NF-AT (Fig. 5A). In contrast, A23187 induced only NF-AT activity (Fig. 5A), suggesting that NF-AT promoter activity is dependent on the calcium signaling. Interestingly, the up-regulation of swiprosin-1 expression was significantly blocked by the NF-κB inhibitor CAPE (Fig. 5B). The effect of CAPE was also mimicked by the proteasome inhibitor MG132, a known indirect inhibitor of NF-κB (Fig. 5B). However, swiprosin-1 expression was not blocked by the NF-AT inhibitor CsA. These data further suggest that NF-κB signaling is critical for the swiprosin-1 induction in T cells.
In this study, we have shown that the expression of actin-regulating protein swiprosin-1 is controlled during T cell activation. Among different PKC isotypes expressed in T cells, PKC-θ was involved in the regulation of swiprosin-1 expression. However, calcium played a negative role for swiprosin-1 expression, suggesting that PKC and calcium may cross-talk in terms of swiprosin-1 expression in T cells. Moreover, we found that swiprosin-1 expression is mediated through nuclear factor NF-κB but not by NF-AT. This result was further corroborated by the pharmacologic approach using PKC or calcium modulators. Taken together, the current results demonstrate that swiprosin-1 is an inducible gene in T cells through PKC-θ and NF-κB axis.
A previous study demonstrated that swiprosin-1 is expressed in spleen, lung, liver, and, most abundantly, in brain (2). In consistent with these data, the authors demonstrated that swiprosin-1 is expressed throughout B-cell differentiation, with the highest expression in immature bone marrow B cells (2). Moreover, recently we have shown that swiprosin-1 is also expressed in mast cells and the expression is regulated through PKC-βI/η pathway (8). We demonstrated that the expression of swiprosin-1 is up-regulated in vivo model tissues of passive cutaneous anaphylaxis and atopic dermatitis (8). Increased expression of swiprosin-1 in tissue models of PCA and atopic dermatitis suggests a potential role for swiprosin-1 in inflammatory responses in vivo. In the current study, although we did not show the in vivo evidence of the expression regulation of swiprosin-1, our results strongly suggest that swiprosin-1 is regulated during T cell activation process in some immunologic situations. Especially, swiprosin-1 may involve in the T cell migration during inflammatory reactions as our unpublished results demonstrate that swiprosin-1 is an actin-binding protein and regulates actin bundling and lamellipodia formation. Interestingly, previous reports demonstrated that PKC-θ pathway is involved in chemokine-mediated T cell migration (14). Therefore, further studies are now in progress to elucidate the role of swiprosin-1 in vivo by making transgenic mice that hyper-express swiprosin-1 in T cells.
Members of the PKC family are expressed in many cell types, where they are known to regulate a wide variety of cellular processes that affect cell growth and differentiation, cytoskeletal remodeling, and gene expression in response to diverse stimuli (15,16). PKC-regulated signaling pathways play an especially significant role in many aspects of immune responses, from development, differentiation, activation, and survival of lymphocytes to macrophage activation (15). It was interesting to be noticed that among various PKC subtypes, only PKC-θ is responsible for the swiprosin-1 expression in T cells. Actually, PKC-θ, first identified in 1992, is a key enzyme in the regulation of T cell activation and survival (17-19). Therefore, PKC-θ is a good candidate for the potential anti-inflammatory therapeutics (11,20). Although current results do not represent that swiprosin-1 is involved in T cell-mediated immune responses, expression regulation through PKC-θ suggests that swiprosin-1 may also be a potential target for the anti-inflammatory therapeutics. Moreover, we think that swiprosin-1 could be a biological marker for T cell activation or inflammatory responses.
The role of swiprosin-1 in T cells is still unknown. As swiprosin-1 potentially involves in actin remodeling (3), it may activate T cell responses. However, our unpublished results demonstrated that overexpression of swiprosin-1 does not mediate T cell activation induced by TCR stimulation or intracellular signaling activation, suggesting that swiprosin-1 is not involved in the initial T cell activation. However, maximum expression of swiprosin-1 at the 6 to 12 h after T cell stimulation suggests that swiprosin-1 may involve in the late phase of T cell activation. Further work is now undertaking to understand the physiology of swiprosin-1 in T cells.
Calcium is important in initiating a signaling cascade leading to the T cell activation combined with the PKC signal (21,22). Cross-linking of TCR results in a sustained influx of extracellular calcium across the plasma membrane and leads to activation of transcription factor, thereby inducing cytokine genes (23-25). In the present study, however, it was surprising that calcium increase by A23187 or ionomycin induces down-regulation of swiprosin-1 rather than induction. Therefore, analysis of promoter regions over the swiprosin-1 genes will be critical to understand why or how calcium signaling inhibits swiprosin-1 expression in T cells. One expectation is that swiprosin-1 has two EF-hand motifs effectively binding to calcium (26,27). This substantial property of swiprosin-1 may modulate intracellular calcium signals or levels, and therefore, it can be inversely assumed that the calcium signal must regulate swiprosin-1 level in cells which, in turn, is important for maintaining intracellular calcium homeostasis as well as calcium signaling evoked by outside stimuli.
T cell is a central player in immune response. Although swiprosin-1 was first discovered in T cells, until now its function in T cells is not clearly demonstrated. However, the expression regulation of swiprosin-1 in T cells strongly suggests its main function in T cells and lymphocyte biology. More importantly, the connection of swiprosin-1 expression with PKC-θ and NF-κB pathway in T cells, with the fact that its expression is also up-regulated in mast cells during activation, implies its usefulness as the biological marker for the certain inflammatory immune reactions.
Figures and Tables
Figure 1
Expression analysis of swiprosin-1 mRNA by RT-PCR. (A) Expression of swiprosin-1 mRNA in Jurkat T cells. Jurkat T cells were stimulated with anti-CD3/CD28, and then the cells were harvested at the indicated time points (0~12 h). The mRNA levels of swiprosin-1 were determined by realtime quantitative PCR (top) and RT-PCR (bottom). GAPDH was used as an internal control. *p<0.01 versus 0 h. (B) Induction of swiprosin-1 in T cells by various stimuli. Jurkat T cells were treated with PMA (200 nM), PHA (1µg/ml), PMA/A23187 (1µM), or PMA/PHA/A23187. At the indicated time points, the expressions of swiprosin-1, IL-2, and IL-3 were determined by RT-PCR. GAPDH was used as an internal control. (C) Western blot analysis of swiprosin-1 expression in T cells. Jurkat T cells were activated by PMA, and then the cells were harvested at the indicated time points (0~24 h). The protein levels were determined by Western blot. All data are from at least three separate experiments. *p<0.01 versus 0 h.
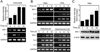
Figure 2
Swiprosin-1 is up-regulated by PMA but down-regulated by calcium ionophore. (A) Swiprosin-1 is regulated by PKC and calcium modulators. Jurkat T cells were pretreated for 30 min with BAPTAAM (50µM), A23187, or ionomycin (1µM), and then the cells were further incubated with medium alone or PMA, anti-CD3 (10µg/ml), and/or anti-CD28 (2µg/ml). After 6 h of incubation, the mRNA levels were determined as described in Fig. 1A. The protein levels were determined at the 18 h as described in Fig. 1C. Data represent three separate experiments. The data shown in the bar graphs represent mean±SD values of triplicate experiments. *p<0.01 versus PMA alone. (B) Jurkat T cells were pretreated for 30 min with MAP kinase inhibitors including SB203580 (10µM), PD098059 (10µM), and SP600125 (10µM), and then the cells were further stimulated for 6 h with PMA. The mRNA levels were determined as described in Fig. 1A. (C) Jurkat T cells were pretreated for 30 min with PKC inhibitors including Rottlerin (10µM), staurosporine (STSN, 500 nM), Gö6976 (100 nM), and Gö6983 (100 nM), and then the cells were further stimulated for 6 h with PMA. The mRNA levels were determined as described in Fig. 1A. Data represent three separate experiments. *p<0.01 versus PMA alone.
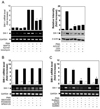
Figure 3
Swiprosin-1 expression is dependent on PKC-θ pathways in T cells. (A) Detection of PKC isoforms by Western blot in Jurkat T cells. (B) Knock-down of PKC isoforms by specific siRNA. Jurkat T cells were transfected with siRNA targeting specific PKC isoforms. At the indicated time points, the expression of PKC isoforms was analyzed by Western blot. Blots were also probed with antibody to β-actin to confirm an equal loading. (C) Knock-down of PKC-θ inhibits phorbol ester-induced swiprosin-1 expression in Jurkat T cells. After 72 h of siRNA transfection against indicated PKC isoforms, cells were treated with or without PMA (200 nM) for 6 h and the expression of swiprosin-1 was analyzed by RT-PCR (top) and quantitative RT-PCR (bottom). Amplification of GAPDH was used as an internal control. Data represent three separate experiments. The data shown in the bar graphs represent mean±SD values of triplicate experiments. *p<0.01 versus scrambled siRNA. SC, scrambled.
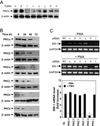
Figure 4
Swiprosin-1 expression is regulated by the PKC-θ pathway in Jurkat T and human primary PBLs. (A and B) Jurkat T cells (A) and primary PBLs (B) were stimulated with anti-CD3/CD28, and then the cells were harvested and the mRNA levels were determined as described by Fig. 1A. The expression of PKC isoforms in primary T cells was analyzed by Western blot. Data represent three separate experiments. The data shown in the bar graphs represent mean±SD values of triplicate experiments. *p<0.01 versus scrambled siRNA. SC, scrambled.
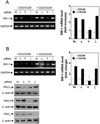
Figure 5
Swiprosin-1 expression is regulated by the NF-κB but not by the NF-AT. (A) Jurkat T cells were transiently transfected with NF-κB or NF-AT luciferase reporter constructs. After 24 h, the cells were treated with PMA, A23187, or PMA/A23187 and incubated for 12 h. NF-κB- or NF-AT-dependent transcriptional activities were determined by luciferase activity assay. Data are representative of three separate experiments. The data shown in bar graphs represent mean±SD values of triplicate experiments. (B) Jurkat T cells were pretreated for 30 min with NF-κB inhibitors including MG132 (1µM), and CAPE (10µM) or NF-AT inhibitor CsA (1µg/ml), and then the cells were further stimulated for 6 h with PMA (left) or PMA/A23187 (right). The mRNA levels were determined as described in Fig. 1A. Data represent three separate experiments.
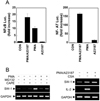
ACKNOWLEDGEMENTS
This work was supported by the Cell Dynamics Research Center (2012-0000766), Korea Healthcare technology R&D Project (A100159) funded by the Ministry for Health, Welfare & Family Affairs, Bio & Medical Technology Development Program (2011-0030157), and Basic Research Program (2012-R1A2A1A03002115) through a National Research Foundation (NRF) grant funded by the Ministry of Education, Science and Technology (MEST).
References
1. Vuadens F, Rufer N, Kress A, Corthésy P, Schneider P, Tissot JD. Identification of swiprosin 1 in human lymphocytes. Proteomics. 2004. 4:2216–2220.


2. Avramidou A, Kroczek C, Lang C, Schuh W, Jäck HM, Mielenz D. The novel adaptor protein Swiprosin-1 enhances BCR signals and contributes to BCR-induced apoptosis. Cell Death Differ. 2007. 14:1936–1947.


3. Ramesh TP, Kim YD, Kwon MS, Jun CD, Kim SW. Swiprosin-1 regulates cytokine expression of human mast cell line HMC-1 through actin remodeling. Immune Netw. 2009. 9:274–284.


4. Blagoev B, Ong SE, Kratchmarova I, Mann M. Temporal analysis of phosphotyrosine-dependent signaling networks by quantitative proteomics. Nat Biotechnol. 2004. 22:1139–1145.


5. Mielenz D, Vettermann C, Hampel M, Lang C, Avramidou A, Karas M, Jäck HM. Lipid rafts associate with intracellular B cell receptors and exhibit a B cell stage-specific protein composition. J Immunol. 2005. 174:3508–3517.


7. Meng X, Wilkins JA. Compositional characterization of the cytoskeleton of NK-like cells. J Proteome Res. 2005. 4:2081–2087.


8. Thylur RP, Kim YD, Kwon MS, Oh HM, Kwon HK, Kim SH, Im SH, Chun JS, Park ZY, Jun CD. Swiprosin-1 is expressed in mast cells and up-regulated through the protein kinase C beta I/eta pathway. J Cell Biochem. 2009. 108:705–715.


9. Hayashi K, Altman A. Protein kinase C theta (PKCtheta): a key player in T cell life and death. Pharmacol Res. 2007. 55:537–544.
10. Freeley M, Long A. Regulating the regulator: Phosphorylation of PKC θ in T Cells. Front Immunol. 2012. 3:227.


11. Kwon MJ, Wang R, Ma J, Sun Z. PKC-θ is a drug target for prevention of T cell-mediated autoimmunity and allograft rejection. Endocr Metab Immune Disord Drug Targets. 2010. 10:367–372.


12. Manicassamy S, Yin D, Zhang Z, Molinero LL, Alegre ML, Sun Z. A critical role for protein kinase C-theta-mediated T cell survival in cardiac allograft rejection. J Immunol. 2008. 181:513–520.


13. Murphy KM, Reiner SL. The lineage decisions of helper T cells. Nat Rev Immunol. 2002. 2:933–944.


14. Shahabi NA, McAllen K, Sharp BM. Stromal cell-derived factor 1-alpha (SDF)-induced human T cell chemotaxis becomes phosphoinositide 3-kinase (PI3K)-independent: role of PKC-theta. J Leukoc Biol. 2008. 83:663–671.


15. Tan SL, Parker PJ. Emerging and diverse roles of protein kinase C in immune cell signalling. Biochem J. 2003. 376:545–552.


16. Michalczyk I, Sikorski AF, Kotula L, Junghans RP, Dubielecka PM. The emerging role of protein kinase Cθ in cytoskeletal signaling. J Leukoc Biol. 2013. 93:319–327.


17. Manicassamy S, Gupta S, Huang Z, Sun Z. Protein kinase C-theta-mediated signals enhance CD4XMLLink_XYZ T cell survival by up-regulating Bcl-xL. J Immunol. 2006. 176:6709–6716.


18. Isakov N, Altman A. Protein kinase C(theta) in T cell activation. Annu Rev Immunol. 2002. 20:761–794.
19. Altman A, Villalba M. Protein kinase C-theta (PKC theta): a key enzyme in T cell life and death. J Biochem. 2002. 132:841–846.
20. Boschelli DH. Small molecule inhibitors of PKCTheta as potential antiinflammatory therapeutics. Curr Top Med Chem. 2009. 9:640–654.


21. Lanzavecchia A. Understanding the mechanisms of sustained signaling and T cell activation. J Exp Med. 1997. 185:1717–1719.


23. Rao A, Luo C, Hogan PG. Transcription factors of the NFAT family: regulation and function. Annu Rev Immunol. 1997. 15:707–747.
24. Chan AC, Desai DM, Weiss A. The role of protein tyrosine kinases and protein tyrosine phosphatases in T cell antigen receptor signal transduction. Annu Rev Immunol. 1994. 12:555–592.

