This article has been corrected. See "Erratum: Mesenchymal Stem Cell Lines Isolated by Different Isolation Methods Show Variations in the Regulation of Graft-versus-host Disease" in Volume 14 on page 66.
Abstract
Since the discovery of the immunomodulation property of mesenchymal stem cells (MSCs) about a decade ago, it has been extensively investigated whether MSCs can be used for the treatment of immune-related diseases, such as graft-versus-host disease (GvHD). However, how to evaluate the efficacy of human MSCs for the clinical trial is still unclear. We used an MHC-mismatched model of GvHD (B6 into BALB/c). Surprisingly, the administration of the human MSCs (hMSCs) could reduce the GvHD-related mortality of the mouse recipients and xenogeneically inhibit mouse T-cell proliferation and IFN-γ production in vitro. We recently established a new protocol for the isolation of a homogeneous population of MSCs called subfractionation culturing methods (SCM), and established a library of clonal MSC lines. Therefore, we also investigated whether MSCs isolated by the conventional gradient centrifugation method (GCM) and SCM show different efficacy in vivo. Intriguingly, clonal hMSCs (hcMSCs) isolated by SCM showed better efficacy than hMSCs isolated by GCM. Based on these results, the MHC-mismatched model of GvHD may be useful for evaluating the efficacy of human MSCs before the clinical trial. The results of this study suggest that different MSC lines may show different efficacy in vivo and in vitro.
Graft-versus-host disease (GvHD) is a potentially life-threatening complication of allogeneic hematopoietic cell transplantation (HCT), in which donor lymphocytes destroy host organs. Glucocorticoids, such as prednisone, are the standard care of acute and chronic GvHD, with a response rate of 30~50% (1,2). However, the outcome of patients with severe steroid refractory GvHD is poor (3). Recently, several antibodies have been tried for the treatment of steroid refractory GvHD, but the outcome is still limited (4,5). Therefore, more effective treatments are needed for steroid-refractory GvHD.
Mesenchymal stem cells (MSCs) are present in almost all adult tissues and organs, such as bone marrow (BM), adipose tissues, umbilical cord blood, adult muscle, corneal stroma, or the dental pulp of teeth (6,7). MSCs possess multi-lineage differentiation into osteoblasts, chondrocytes, adipocytes, and neural cells and cannot serve as effective antigen-presenting cells to promote immune responses because of lacking major histocompatibility complex II (MHC II) expression (6,8). In addition, MSCs show immunomodulatory and anti-inflammatory effects on various activated lymphoid cells, including T cells, B cells, natural killer cells, and dendritic cells (9-13). Although the molecular mechanism of the immunosuppressive effects of MSCs is not completely understood, it has been extensively investigated whether MSCs can be used for cell-based therapy in immune-related diseases such as steroid-refractory GvHD (14-16). Le Blanc et al. (15) showed that MSCs reduce incidence of GvHD after allogeneic HCT in phase 2 study. However, another clinical trial failed to demonstrate MSC efficacy in phase 3. There was a statistical improvement over placebo in patients with GI and liver GvHD, but the other group of GvHD patients showed no improvement after MSC treatment (17). In this point, one of the important factors to consider is that all MSCs isolated from different adult donors are not identical.
Autologous cells are generally preferred to allogeneic or xenogeneic cells for transplantation due to concerns regarding immunosafety and disease transmission. Several studies have reported that xenogeneic MSCs can be used as cell therapy for disease treatment. Human MSCs (hMSCs) were used for cartilage repair in pigs or rabbits and for stroke treatment in a rat model (18-20). Bruck et al. (21) tested in vivo efficacy of hMSCs using a humanized GvHD mouse model (hMSCs into NOD/SCID). However, humanized mouse modeling was not simple because human PBMC should be freshly prepared for each experiment. In addition, injection of human BM-derived MSCs did not prevent xenogeneic GvHD in a humanized mouse model (21). To find a method evaluating the in vivo efficacy of hMSCs before clinical trial will be useful for successful treatment of GvHD patients.
Previously, we established a new isolation method for clonal hMSC (hcMSCs) lines, which is called the subfractionation culturing method (SCM) (22). We showed that different hcMSC lines exhibit a different in vitro immunomodulatory property although they were isolated from the same mouse BM and showed the same cell surface marker expression (23). We investigated whether in vivo xenogeneic efficacy of BM-derived MSC lines, isolated by either GCM or SCM, can be evaluated using the MHC-mismatched mouse GVHD model system and whether the MSC lines show different in vivo efficacy in the regulation of GVHD.
C57BL/6J (B6) and Balb/c were purchased from Central Lab Animal Inc. (Seoul, Korea). Five-week-old C57BL/6J (B6) female mice were used as donors of BM and splenocytes. Ten-week-old BALB/c male mice were used as recipients. All mice were maintained under specific pathogen-free conditions.
Anti-CD3 (2C11), anti-CD28 (37.451), IFN-γ ELISA kit, and recombinant mouse and human IFN-γ and TNF-α were purchased from BD Bioscience (Franklin Lakes, NJ, USA).
MSCs were characterized by flow cytometry according to the cell surface antigens and the differentiation potential into adipocytes, chondrocytes, and osteocytes (22,23). Used cell surface antibodies for mouse: anti-CD34 (RAM34), CD44 (IM7), CD45 (30F11), CD73 (TY/11.8), CD105 (FAB1320F), CD117 (2B8), MHC II (2G9), Sca-1 (D7). Used cell surface antibodies for human: anti-CD34 (581), CD44 (Bu52), CD45 (2D1), CD73 (AD2), CD90 (5E10), CD105 (266), CD117 (104D2), MHC II (G466).
Mouse MSC lines (mcMSC2, mcMSC2, and mcMSC3) were isolated from BM of C3H/HeN. All human MSC lines were isolated from BM of different healthy donors. Using conventional isolation method (GCM for gradient centrifugation method), hMSC1, hMSC3, hMSC4, and hMSC6 were isolated from 4 different donors. MSCs named by hcMSC1, hcMSC2, hcMSC4, hcMSC5, and hcMSC6, were isolated from 5 different donors according to SCM (22). The hMSC6 and hcMSC6 were isolated from BM of a single donor by GCM and SCM, respectively. All MSCs were cultured with low glucose-DMEM supplemented with 20% FBS and 1% penicillin/streptomycin. Human MSCs of passage 5 to 13 were used in this study.
Recipient mice were irradiated in a dose of 8.5 Gy from 4MV LINAC (Siemens, Berlin, Germany). BM and spleen cells from B6 female mice were injected 24 hours after the irradiation. BM cells were flushed from the femur and tibia of each donor mouse with phosphate-buffered saline (PBS). A single-cell suspension of donor BM and splenocytes was filtered through a sterile nylon mesh, washed, and resuspended in PBS. Then, 5×106 BM cells and 5×106 splenocytes were admixed in PBS and injected intravenously in a volume of 200 µl into the lateral tail vein. One and three days later, 5×105 MSCs were injected intravenously and survival rates, weight, clinical symptoms, and immune cells in blood were monitored. Kaplan-Meier analyses were used to the statistical significance of survival curves and p-value was described in the results.
Human peripheral blood mononuclear cells (PBMCs) were isolated from 2 healthy donors, and 1×105 PBMCs of each donor were co-cultured in a flat bottom 96-well plate for mixed lymphocyte reaction (MLR). To investigate the effect of hcMSCs on T-cell proliferation, an indicated cell number of hcMSCs were co-cultured with PBMCs for 5 days, e.g. at a ratio of 1:20 or 1:100 means that 2,000, 4,000, 104, or 4×104 MSCs were co-cultured with 2×105 PBMCs. Also, 2×105 PBMCs were stimulated with 1 µg/ml PHA for 3 days. Mouse splenocytes were isolated from B6 and BALB/c mice, and 1×105 splenocytes of each mouse were co-cultured for MLR. Then, 2×105 splenocytes were stimulated with 1 µg/ml anti-CD3 and anti-CD28 antibodies in a flat bottom 96-well plate for 3 days. 1 µCi 3H was added during the last 16 h of culture and the T-cell proliferation was determined by thymidine incorporation. In the presence or absence of indicated MSCs, 1×106 PBMCs or mouse splenocytes were stimulated with either 1 µg/ml PHA or 1 µg/ml anti-CD3 and anti-CD28 antibodies, respectively. After 48 h, supernatants were collected for IFN-γ ELISA. Student's t tests were used to determine statistical significance (SigmaPlot software, SPSS Inc., Chicago, USA). p-value was described in the results.
To examine whether human MSCs increase the expression of indoleamine 2, 3-dioxygenase (IDO), TGF-β and GAPDH in the presence of mouse recombinant cytokines, 1×106 hMSCs were cultured with either mouse or human recombinant IFN-γ (20 ng/ml) and TNF-α (10 ng/ml) in a 10-cm-diameter dish. After 24 h, RNA was isolated 2 µg of RNA was converted into cDNA using QuantiTect Reverse Transcription Kit (Qiagen, USA). Using PCR Premix kit, PCR was performed with following primers (Bioneer, Korea): IDO (F 5'-CGC TGT TGG AAA TAG CTT C-3' and R 5'-CAG GAC GTC AAA GCA CTG AA-3'), TGF-β(F 5'-CAG ATC CTG TCC AAG CTG-3' and R 5'-TCG GAG CTC TGA TGT GTT-3'), and GAPDH (F 5'-CCA CTG GCG TCT TCA CCA C-3' and R 5'-CCT GCT TCA CCA CCT TCT TG-3').
Several important factors, including the time point of MSCs injection, the infusion number of MSCs, the source of MSCs, and the GvHD animal model, should be considered for the successful prevention of GvHD. In order to establish a reliable protocol for GvHD prevention, we tested well-established an MHC-mismatched mouse GvHD (B6 into BALB/c) model (Fig. 1A). First, we attempted to determine the efficacy of MSCs in a GvHD mouse model by applying mouse clonal MSCs (mcMSC), named mcMSC1. The GvHD-induced mice were intravenously infused with 5×105 mcMSC1 twice at a 1-day interval, and mcMSC1 significantly reduced allogeneic GvHD mortality (p=0.001) (Fig. 1B). The mcMSC1 inhibited T-cell proliferation and IFN-γ production in vitro (Fig. 1C). We isolated two other mcMSC lines from BM of C3H/HeN mice and tested whether both mcMSC lines showed the same in vivo efficacy. Intriguingly, GvHD development was prevented by mcMSC3 (p=0.003) but not by mcMSC2 (p=0.897) (Fig. 2A), although both lines significantly inhibited T-cell proliferation and IFN-γ production in vitro (Fig. 2B). These results suggest that in vitro immunomodulatory activity is not a good indicator to predict in vivo efficacy.
It has been reported that adult stem cells do not induce immunoreactivity even to xenografts (19,24,25). Previously, we showed that hcMSCs fail to reduce mouse allogeneic GvHD-related mortality in an MHC-matched GvHD model (B6 into BALB/b) (26). Surprisingly, hcMSC1 successfully reduced the GvHD-related mortality (p=0.005) in MHC-mismatched model of GvHD (B6 into BALB/c) (Fig. 3A). The hcMSC1 inhibited human T-cell proliferation and IFN-γ production in a dose-dependent manner (Fig. 3B). To examine how xenogeneic hcMSC1 can prevent the mouse GvHD-induced mortality, we next investigated whether hcMSCs can suppress mouse T cell proliferation and IFN-γ production in vitro. The hcMSC1 suppressed the mouse allogeneic MLR and IFN-γ production when mouse T cells were stimulated with anti-CD3 and anti-CD28 antibodies (Fig. 3C).
It has been suggested that the immunosuppressive effect exerted by MSCs requires prestimulation by proinflammatory cytokines, such as IFN-γ and TNF-α (27,28). These cytokines can activate MSCs to produce nitric oxide (NO), IDO, and chemokines which can recruit T cells and suppress them locally (29,30). Therefore, we investigated whether mouse or human cytokines activate hcMSC1. As shown in Fig. 3D, mouse cytokines, such as IFN-γ and TNF-α, did not induce IDO expression in 2 different hcMSC lines, although human cytokines strongly induced IDO expression (Fig. 3D). The production of TGF-β by hcMSCs was constitutively maintained even after the treatment with IFN-γ and TNF-α of both species. These results were consistent with our previous data (26), implying that hMSCs generally do not respond to mouse IFN-γ and TNF-α. Collectively, hcMSCs which can inhibit mouse T-cell proliferation and IFN-γ production are likely to alleviate mouse allogeneic GvHD. Mouse IFN-γ and TNF-α may not be involved in the immunomodulatory effects of human MSCs in xenogeneic mouse hosts.
As mentioned above, we newly established MSC isolation method called SCM to successfully isolate clonal stem cells (22,23). We examined whether MSCs isolated by different methods exhibit a different in vivo immunomodulatory activity. MHC-mismatched GvHD (B6 into BALB/c) mice were intravenously infused with hMSC3 and hcMSC4, which were isolated from 2 different donors by GCM and SCM, respectively. Both MSC lines significantly inhibited mouse T-cell proliferation in vitro. The hMSC3 (p=0.032) alleviated the GvHD, whereas hcMSC4 (p=0.329) did not show statistically significance. To make sure, we repeated the experiments using other MSC lines isolated from different donors. GvHD-related death was rather delayed by SCM-isolated hcMSC2 (p=0.014) but not by GCM-isolated hMSC1 (p=0.124) (Fig. 4C). Both MSC lines inhibited human T-cell proliferation (Fig. 4D). On the other hand, hcMSC2 significantly inhibited mouse T-cell proliferation, whereas hMSC1 did not (Fig. 4E). These results suggest that MSC efficacy in GvHD treatment may be donor-dependent. To obtain further evidence, we next isolated MSCs according to different methods from a single donor and injected into GvHD mice. The hcMSC6 (p=0.005) isolated by SCM alleviated GvHD more potently than hMSC6 (p=0.469) isolated by GCM (Fig. 5A). Both MSC lines inhibited human T-cell proliferation. However, mouse IFN-γ production was significantly inhibited by hcMSC6 but not by hMSC6 (Fig. 5B). Thus, MSC efficacy may differ according to donors and/or to MSC lines of the same donor.
In this study, we evaluated the efficacy of human BM-derived MSCs in xenogeneic GVHD. We found that hcMSCs showing suppressive effects on both human and mouse T-cell proliferation and IFN-γ production in vitro did alleviate an MHC-mismatched model of GvHD (B6 into BALB/c). Using this model, we revealed, for the first time, that different MSC lines show different in vivo efficacy.
A number of studies have analyzed the ability of MSCs to prevent GvHD in mice (31). However, the results were controversial. A study showed that in an MHC-mismatched GvHD (B6 into BALB/c), B6 BM-derived MSCs infused on day 0 did not decrease in vivo T-cell activation and failed to decrease GvHD incidence or severity, although these MSCs strongly inhibited T-cell proliferation in vitro (32). Another study showed that BALB/c BM-derived MSCs infused on day 0 failed to decrease GvHD incidence or severity in a BALB/c (H-2d/d) into B6 (H-2b/b) GvHD model, whereas MSCs given either on day 2 or on day 20 after BM transplantation improved survival and decreased GvHD symptoms (33). In addition, adipocyte-derived MSCs (Ad-MSCs) from B6D2F1 mice given on days 0, 7, and 14 after BM transplantation improved survival and decreased GvHD in an MHC-haploidentical model (B6 (H-2b/b) into B6D2F1 (H-2b/d)). However, AdMSCs given on days 14, 21, and 28 failed to prevent GvHD mortality (34). All results demonstrated that timing, the number of MSC infusions, the source of MSCs, and the GvHD mouse model play important roles in GvHD prevention. Using the MHC-mismatched model of GvHD (B6 into BALB/c), BM-MSCs could not alleviate GvHD when we injected MSCs once on day 1 after BM transplantation (data not shown). Multiple injections of MSCs on days 1 and 3 significantly improved GvHD. Nonetheless, MSCs appeared to just prolong survival but fail to cure GvHD. MSCs should be injected more than twice to improve the survival rate when injected 5×105 MSCs. It has recently been published that placenta-derived MSCs (PD-MSCs) control GvHD in an MHC-mismatched GvHD model (B6 into BALB/c) (35). Mice receiving PD-MSCs on day 0 after BM transplantation showed significantly increased survival rates. However, the authors did not present the mechanism by which PD-MSCs can control GvHD. A single injection of 1×106 PD-MSCs showed better efficacy compared to BM-MSCs which were injected twice. It may be speculated that PD-MSCs are very young MSCs and thus show better efficacy than adult BM-MSCs. All of these results demonstrated that 1) human MSCs can alleviate mouse allogeneic GvHD in an MHC-mismatched GvHD model (B6 into BALB/c) which can be useful for the in vivo efficacy evaluation of human MSCs, 2) human MSCs, which can reduce the GvHD mortality, are able to inhibit mouse T-cell proliferation and IFN-γ production, 3) efficacy of MSCs may be dependent on donors, sources, or isolation methods. MSCs with better quality can be obtained by the SCM because more homogenous MSCs can be isolated by SCM rather than GCM.
This study, for the first time, showed that different MSC lines, no matter which isolation method was used, may possess different in vivo efficacy in the regulation of GvHD. Therefore, pre-evaluation of MSC products should be performed in an animal model, such as the MHC-mismatched GvHD model (B6 into BALB/c) before a clinical trial on the treatment of GvHD.
Figures and Tables
Figure 1
In vivo efficacy model for MSC. (A) BALB/c mice were irradiated in a dose of 8.5 Gy. Twenty-four hours after irradiation, 5×106 BM and 5×106 spleen cells from B6 female mice were injected. On days 1 and 3 after transplantation, 5×105 MSCs were injected intravenously. (B) Survival rates were monitored. (C) Lymphocytes from the spleen and lymph node were stimulated with anti-CD3 and anti-CD28 antibodies in the presence or absence of mcMSC1, and mcMSC1 was co-cultured with lymphocytes at a ratio of 1:20 or 1:100 (MSCs: lymphocytes). T cell proliferation and IFN-γ production were measured by [3H]-thymidine incorporation and ELISA, respectively. T cell proliferation and IFN-γ assays were repeated three times and similar results were obtained. L, lymphocytes; M, MSCs; BM, bone marrow, ***p<0.005, *p<0.05.
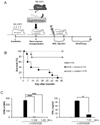
Figure 2
Different in vivo efficacy of mouse cMSC lines. (A) The mcMSC2 and mcMSC3 isolated from BM of C3H/HeN mice were introduced into the GvHD mice. The percentage of survival was plotted. The weight changes of the BALB/c recipients were measured over the experiment period. (B) Both MSC lines were co-cultured with lymphocytes in vitro which were stimulated with anti-CD3 and anti-CD28 antibodies. Mouse T-cell proliferation and IFN-γ production were measured by [3H]-thymidine incorporation and ELISA, respectively. T cell proliferation and IFN-γ assays were repeated three times and similar results were obtained. ***p<0.005, *p<0.05.
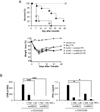
Figure 3
In vivo efficacy of human cMSCs. (A) The hcMSC1 isolated from the BM of healthy donors were injected into the GvHD mice. The percentage of survival was plotted. (B) PBMCs from 2 different donors were co-cultured with hcMSC1 in indicated doses for 5 days. Human T-cell proliferation was measured by [3H]-thymidine incorporation. PBMC were stimulated with PHA for 48 h in the presence of hcMSC1, and IFN-γ production were measured in culture media by ELISA. (C) The hcMSC1 were co-cultured with lymphocytes from 2 different mouse strains for 5 days. Mouse T-cell proliferation was measured by [3H]-thymidine incorporation. BALB/c lymphocytes were stimulated with anti-CD3 and anti-CD28 antibodies for 48 h in the presence of hcMSC1, and IFN-γ production were measured in culture media by ELISA. T cell proliferation and IFN-γ assays were repeated twice. (D) The hcMSC1 and hMSC1 were stimulated either mouse or human recombinant IFN-γ and TNF-α for 24 h, and the mRNA expressions of human IDO, TGF-β, and GAPDH were measured by RT-PCR. *p<0.05, **p<0.01.
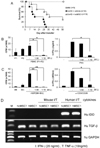
Figure 4
In vivo efficacy of human MSC lines isolated from different donors. (A) The hMSC3 and hcMSC4 isolated from the BM of 2 different donors by GCM and SCM, respectively, were injected into the GvHD mice. The percentage of survival was plotted. (B) Both human MSCs were co-cultured with lymphocytes from 2 different mouse strains for 5 days. Mouse T-cell proliferation was measured by [3H]-thymidine incorporation. (C) The hMSC1 and hcMSC2 isolated from BM of 2 other donors by GCM and SCM, respectively, were injected into the GvHD mice. The percentage of survival was plotted. (D) PBMCs from 2 different donors were co-cultured with hMSC1 or hcMSC2 in indicated doses for 5 days. Human T-cell proliferation was measured by [3H]-thymidine incorporation. (E) The hMSC1 or hcMSC2 were co-cultured with lymphocytes from 2 different mouse strains for 5 days. BALB/c lymphocytes were stimulated with anti-CD3 and anti-CD28 antibodies for 3 days in the presence of either hMSC1 or hcMSC2. Mouse T-cell proliferation was measured by [3H]-thymidine incorporation. T cell proliferation assays were repeated twice and similar results were obtained. *p<0.05, **p<0.01
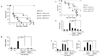
Figure 5
In vivo efficacy of human MSC lines isolated from a single donor. (A) The hMSC6 and hcMSC6 isolated from the BM of a single donor by GCM and SCM, respectively, were injected into the GvHD mice. The percentage of survival was plotted. (B) PBMCs were stimulated with PHA for 3 days in the presence of either hMSC6 or hcMSC6. Human T-cell proliferation was measured by [3H]-thymidine incorporation. (C) BALB/c lymphocytes were stimulated with anti-CD3 and anti-CD28 antibodies for 48 h in the presence of either hMSC6 or hcMSC6. IFN-γ productions were measured in culture media by ELISA. T cell proliferation and IFN-γ assays were repeated twice and similar results were obtained. *p<0.05, ***p<0.005
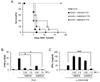
ACKNOWLEDGEMENTS
This work was supported by the Bio & Medical Technology Development Program (NRF-2011-0019634 and NRF-2011-0019637) and the Women Scientists Program (NRF-2011-0013452) of the National Research Foundation by the Korean government (MEST), and by a grant from Inha University (45089-01).
References
1. Goker H, Haznedaroglu IC, Chao NJ. Acute graft-vs-host disease: pathobiology and management. Exp Hematol. 2001; 29:259–277.


2. Menillo SA, Goldberg SL, McKiernan P, Pecora AL. Intraoral psoralen ultraviolet A irradiation (PUVA) treatment of refractory oral chronic graft-versus-host disease following allogeneic stem cell transplantation. Bone Marrow Transplant. 2001; 28:807–808.


4. Rager A, Frey N, Goldstein SC, Reshef R, Hexner EO, Loren A, Luger SM, Perl A, Tsai D, Davis J, Vozniak M, Smith J, Stadtmauer EA, Porter DL. Inflammatory cytokine inhibition with combination daclizumab and infliximab for steroid-refractory acute GVHD. Bone Marrow Transplant. 2011; 46:430–435.


5. Schub N, Günther A, Schrauder A, Claviez A, Ehlert C, Gramatzki M, Repp R. Therapy of steroid-refractory acute GVHD with CD52 antibody alemtuzumab is effective. Bone Marrow Transplant. 2011; 46:143–147.


6. Prockop DJ. Marrow stromal cells as stem cells for nonhematopoietic tissues. Science. 1997; 276:71–74.


7. Branch MJ, Hashmani K, Dhillon P, Jones DR, Dua HS, Hopkinson A. Mesenchymal stem cells in the human corneal limbal stroma. Invest Ophthalmol Vis Sci. 2012; 53:5109–5116.


8. Tse WT, Pendleton JD, Beyer WM, Egalka MC, Guinan EC. Suppression of allogeneic T-cell proliferation by human marrow stromal cells: implications in transplantation. Transplantation. 2003; 75:389–397.


9. Di Nicola M, Carlo-Stella C, Magni M, Milanesi M, Longoni PD, Matteucci P, Grisanti S, Gianni AM. Human bone marrow stromal cells suppress T-lymphocyte proliferation induced by cellular or nonspecific mitogenic stimuli. Blood. 2002; 99:3838–3843.


10. Jiang XX, Zhang Y, Liu B, Zhang SX, Wu Y, Yu XD, Mao N. Human mesenchymal stem cells inhibit differentiation and function of monocyte-derived dendritic cells. Blood. 2005; 105:4120–4126.


11. Sotiropoulou PA, Perez SA, Gritzapis AD, Baxevanis CN, Papamichail M. Interactions between human mesenchymal stem cells and natural killer cells. Stem Cells. 2006; 24:74–85.


12. Corcione A, Benvenuto F, Ferretti E, Giunti D, Cappiello V, Cazzanti F, Risso M, Gualandi F, Mancardi GL, Pistoia V, Uccelli A. Human mesenchymal stem cells modulate B-cell functions. Blood. 2006; 107:367–372.


13. Glennie S, Soeiro I, Dyson PJ, Lam EW, Dazzi F. Bone marrow mesenchymal stem cells induce division arrest anergy of activated T cells. Blood. 2005; 105:2821–2827.


14. Le Blanc K, Rasmusson I, Sundberg B, Gotherstrom C, Hassan M, Uzunel M, Ringden O. Treatment of severe acute graft-versus-host disease with third party haploidentical mesenchymal stem cells. Lancet. 2004; 363:1439–1441.


15. Le Blanc K, Frassoni F, Ball L, Locatelli F, Roelofs H, Lewis I, Lanino E, Sundberg B, Bernardo ME, Remberger M, Dini G, Egeler RM, Bacigalupo A, Fibbe W, Ringden O. Developmental Committee of the European Group for Blood and Marrow Transplantation. Mesenchymal stem cells for treatment of steroid-resistant, severe, acute graft-versus-host disease: a phase II study. Lancet. 2008; 371:1579–1586.


16. Ringden O, Uzunel M, Rasmusson I, Remberger M, Sundberg B, Lonnies H, Marschall HU, Dlugosz A, Szakos A, Hassan Z, Omazic B, Aschan J, Barkholt L, Le Blanc K. Mesenchymal stem cells for treatment of therapy-resistant graft-versus-host disease. Transplantation. 2006; 81:1390–1397.


18. Li WJ, Chiang H, Kuo TF, Lee HS, Jiang CC, Tuan RS. Evaluation of articular cartilage repair using biodegradable nanofibrous scaffolds in a swine model: a pilot study. J Tissue Eng Regen Med. 2009; 3:1–10.


19. Liao W, Xie J, Zhong J, Liu Y, Du L, Zhou B, Xu J, Liu P, Yang S, Wang J, Han Z, Han ZC. Therapeutic effect of human umbilical cord multipotent mesenchymal stromal cells in a rat model of stroke. Transplantation. 2009; 87:350–359.


20. Yan H, Yu C. Repair of full-thickness cartilage defects with cells of different origin in a rabbit model. Arthroscopy. 2007; 23:178–187.


21. Bruck F, Belle L, Lechanteur C, de Leval L, Hannon M, Dubois S, Castermans E, Humblet-Baron S, Rahmouni S, Beguin Y, Briquet A, Baron F. Impact of bone marrow-derived mesenchymal stromal cells on experimental xenogeneic graft-versus-host disease. Cytotherapy. 2013; 15:267–279.


22. Song SU, Kim CS, Yoon SP, Kim SK, Lee MH, Kang JS, Choi GS, Moon SH, Choi MS, Cho YK, Son BK. Variations of clonal marrow stem cell lines established from human bone marrow in surface epitopes, differentiation potential, gene expression, and cytokine secretion. Stem Cells Dev. 2008; 17:451–461.


23. Jeon MS, Yi TG, Lim HJ, Moon SH, Lee MH, Kang JS, Kim CS, Lee DH, Song SU. Characterization of mouse clonal mesenchymal stem cell lines established by subfractionation culturing method. World J Stem Cells. 2011; 3:70–82.


24. Saito T, Kuang JQ, Bittira B, Al-Khaldi A, Chiu RC. Xenotransplant cardiac chimera: immune tolerance of adult stem cells. Ann Thorac Surg. 2002; 74:19–24.


25. Liechty KW, MacKenzie TC, Shaaban AF, Radu A, Moseley AM, Deans R, Marshak DR, Flake AW. Human mesenchymal stem cells engraft and demonstrate site-specific differentiation after in utero transplantation in sheep. Nat Med. 2000; 6:1282–1286.


26. Jeon MS, Lim HJ, Yi TG, Im MW, Yoo HS, Choi JH, Choi EY, Song SU. Xenoreactivity of human clonal mesenchymal stem cells in a major histocompatibility complex-matched allogeneic graft-versus-host disease mouse model. Cell Immunol. 2010; 261:57–63.


27. Ren G, Zhang L, Zhao X, Xu G, Zhang Y, Roberts AI, Zhao RC, Shi Y. Mesenchymal stem cell-mediated immunosuppression occurs via concerted action of chemokines and nitric oxide. Cell Stem Cell. 2008; 2:141–150.


28. Dazzi F, Marelli-Berg FM. Mesenchymal stem cells for graft-versus-host disease: close encounters with T cells. Eur J Immunol. 2008; 38:1479–1482.


29. Sato K, Ozaki K, Oh I, Meguro A, Hatanaka K, Nagai T, Muroi K, Ozawa K. Nitric oxide plays a critical role in suppression of T-cell proliferation by mesenchymal stem cells. Blood. 2007; 109:228–234.


30. Katz JB, Muller AJ, Prendergast GC. Indoleamine 2,3-dioxygenase in T-cell tolerance and tumoral immune escape. Immunol Rev. 2008; 222:206–221.


31. Baron F, Storb R. Mesenchymal stromal cells: a new tool against graft-versus-host disease? Biol Blood Marrow Transplant. 2012; 18:822–840.


32. Sudres M, Norol F, Trenado A, Gregoire S, Charlotte F, Levacher B, Lataillade JJ, Bourin P, Holy X, Vernant JP, Klatzmann D, Cohen JL. Bone marrow mesenchymal stem cells suppress lymphocyte proliferation in vitro but fail to prevent graft-versus-host disease in mice. J Immunol. 2006; 176:7761–7767.


33. Polchert D, Sobinsky J, Douglas G, Kidd M, Moadsiri A, Reina E, Genrich K, Mehrotra S, Setty S, Smith B, Bartholomew A. IFN-gamma activation of mesenchymal stem cells for treatment and prevention of graft versus host disease. Eur J Immunol. 2008; 38:1745–1755.

