Abstract
Emerging evidence suggests that gap formation and opening of the endothelial junctions during leukocyte extravasation is actively controlled to maintain the integrity of the vascular barrier. While the role for endothelial cells to this process has been well defined, it is not clear whether leukocytes are also actively contributing to endothelial barrier function. We have recently showed that extravasating leukocytes deposit microparticles on the subendothelium during the late stages of extravasation, which is LFA-1 dependent. Using multiphotonintravital microscopy (MP-IVM) of mouse cremaster muscle vessels in the current work, we show that microparticle formation and deposition maintains the integrity of the microvascular barrier during leukocyte extravasation. Inhibition of neutrophil-derived microparticle formation resulted in dramatically increased vascular leakage. These findings suggest that deposition of microparticles during neutrophil extravasation is essential for maintaining endothelial barrier function and may result in temporal difference between neutrophil extravasation and an increase in vascular leakage.
Recent intravital imaging studies revealed that LFA-1 (CD11a/CD18) and Mac-1 (CD11b/CD18) play distinct roles in leukocyte adhesion and crawling (1-3). A study showed that, in neutrophils, endothelial adhesion was mediated by LFA-1 and that crawling was mediated by Mac-1 (1). However, in monocytes and lymphocytes, crawling was shown to be LFA-1 dependent (2,4). These data indicate that the transmigration of different leukocyte subsets through the endothelium may be distinctly regulated by LFA-1 and/or Mac-1. In the final stage of migration through the endothelial cells and basement membrane during leukocyte extravasation, the leukocytes must detach uropod from the basolateral side of the endothelial layer and/or basement membrane. During uropod detachment of the elongated leukocytes, microparticles are deposited at the subendothelium, and this represents the final step in leukocyte extravasation (5). Ligation of apical endothelial surface receptors, including ICAM-1 during the early stages of leukocyte transendothelial migration has been shown to trigger rearrangement of the interendothelial junctions and increased vascular permeability (6). However, to avoid tissue edema, vascular barrier integrity must be maintained during active leukocyte transendothelial migration. This involves rapid resealing of the vascular endothelial layers upon detachment of the extravasating leukocytes. Leukocytes migrating across endothelium during final detachment from the vascular wall were found to form and deposit microparticles on the basolateral endothelial surface. Thus we examined whether microparticle deposition affects the integrity of the vascular barrier during leukocyte transendothelial migration. We used MP-IVM to investigate the role for microparticle deposition during leukocyte extravasation. We further specifically depleted neutrophils and monocytes to determine the role for the specific circulating leukocyte subsets in this process. Our data suggest that the CD18+ microparticles generated from the tip of neutrophil uropods during detachment contribute to the resealing of the vasculature, thus helping maintain endothelial barrier integrity.
CD18-mCFP knock-in mice were generated at the Gene Targeting and Transgenic Core facility at the University of Rochester (5). C57BL/6 and CCR2 knockout mice (7) were maintained in a specific pathogen-free environment at the University of Rochester's animal facility. The Institutional Review Board of the University of Rochester approved all animal experiments.
The cremaster muscles of C57BL/6 mice were superfused with physiological salt solution alone or containing a cocktail of chemokines (30 nM CXCL2, 12.5 nM CXCL12, and 1 nM CCL2) in the presence of 500 µg of CD11a blocking Ab (M17/4) or control IgG at 37℃ for 2 h. Following euthanasia, the vascular wash-out was done with 10 ml of physiological salt solution buffer and then the cremaster muscle was removed from mouse and minced. The minced tissue was treated with 3 ml of 0.5% Collagenase II (Invitrogen) in HBSS containing 3 mM CaCl2 at 37℃ for 1 h. Whole cells were collected by centrifugation at 4,000 g for 20 min and labeled with Alexa488-anti-mouse CD31 Ab at 4℃ overnight. The supernatant was subjected to ultracentrifugation to collect the microparticles and labeled with PE conjugated anti-mouse CD18 Ab (M18/2) (8). Then, the ratio of CD18+ microparticles to CD31+ whole cells was calculated using flow cytometry.
Mouse cremaster venules were imaged with MP-IVM as described (5). Texas Red dextran (70,000 Mw, Invitrogen) was i.v. injected (20 mg/kg) via femoral vein to label the blood vessels prior to imaging. During MP-IVM, the core body temperature of the mice was maintained using a warming pad set to 37℃. The blood vessels were stimulated by superfusion of chemokine (1 nM CXCL2) or bacterial chemoattractant (1 µM fMLP). To investigate the extravascular blocking effect of LFA-1 on leukocyte extravasation and vascular leakage, 500 µg of CD11a blocking Ab was superfused onto exteriorized mouse cremaster.
To investigate the contribution of leukocyte subsets to vascular leakage, leukocyte subsets were depleted and then vascular leakage was measured as described below. Intraperitoneal injection (25 µg/day for 3 days) of anti-Ly6C/Ly6G Ab (RB6-8C5, Gr1) was performed to deplete neutrophils and inflammatory monocytes, and anti-Ly6G Ab (1A8) was injected to deplete only neutrophils (9-11). Monocyte was depleted by i.v. injection of clodronate liposomes (48 h (200 µl), 24 h (200 µl), and 6 h (100 µl)) before MP-IVM (12,13). CCR2 knockout mice were used to investigate the contribution of inflammatory monocytes to vascular leakage (10,14). Except CCR2 knockout mice, depletions of other leukocyte subsets were done in C57BL/7 mice. These protocols have been shown to deplete more than 90% of the targeted leukocyte subset in mice.
Vascular leakage was measured from unstimulated and stimulated cremaster venules. Briefly, Texas Red dextran was i.v. injected into mice. The images of Texas Red signals (dextran leakage) from the cremaster venules were acquired with MP-IVM. The fluorescence ratio (FR) of extravascular Texas Red (EX) to intravascular Texas Red (IN) was calculated by quantifying the mean fluorescence intensity in restricted extravascular and intravascular regions. The mean EX was divided by the mean IN for each time point (FREX/IN). FREX/IN was normalized to time 0 (FRt0) as (FREX/IN)/(FRt0) (15). (FREX/IN)/(FRt0) was integrated to measure the accumulated vascular leakage of dextran at each time point. Volocity software (PerkinElmer) was used to measure Texas Red intensity.
We have recently showed that leukocytes become extremely elongated and deposit microparticles on the subendothelium during extravasation through inflamed vessels in an LFA-1-dependent manner (5). Hereby, we hypothesized that leukocyte-derived microparticles play a protective role, and prevent vascular leakage during leukocyte extravasation, by either forming a physical barrier on the subendothelium or inducing signaling to regulate vascular barrier function.
To investigate this hypothesis, we first quantified the number of β2 integrin (CD18+) containing microparticles formed per endothelial cell of the vasculature in chemokines-stimulated tissue. The number of CD18+ microparticles isolated from chemokines-stimulated cremaster tissue was normalized to the total number of CD31+ cells isolated from the same tissue. Superfusion of LFA-1 blocking Ab over the extravascular regions of the cremaster muscle dramatically decreased microparticle formation, compared to control IgG superfusion (Fig. 1A). The physical properties of microparticles derived from migrating leukocytes were determined to be <1 µm using flow cytometry (Fig. S1). Therefore, we further examined the effects of microparticle formation on vascular barrier during CXCL2-induced neutrophil migration across the vascular wall. We calculated the vascular leakage in exteriorized mouse cremaster venules by measuring Texas Red fluorescence intensity in the interstitial area adjacent to blood vessels of CD18-mCFP mice, which were i.v. injected with Texas Red dextran. Inhibition of the LFA-dependent microparticle formation by superfusion of the LFA-1 blocking Ab significantly increased dextran leakage (~4.2 fold), compared to the unstimulated condition (Fig. 1B and C). We confirmed that both intravascular leukocyte adhesion and transendothelial migration were not affected by extravascular inhibition of LFA-1 function (Fig. 1D and E). These findings suggest that the observed increase in dextran leakage under this condition was specifically due to decreased formation and deposition of microparticles on the subendothelium.
Neutrophil extravasation is often linked to vascular leakage (16-18) leading to tissue injury in inflammatory models (19,20). Therefore, we expanded our study to determine which subset of circulating leukocytes was primarily responsible for the protective effect on vascular barrier function through deposition of microparticles during extravasation. To examine this, vascular leakage during leukocyte extravasation (induced by fMLP) was further assessed after specific depletion of both/either intravascular neutrophils and/or monocytes, with and without inhibition of LFA-1 (by extravascular superfusion of LFA-1 blocking Ab). Consistent with previous studies (21), Ab-mediated neutrophil depletion showed complete preservation of vascular barrier function independently of inhibition of LFA-1 mediated microparticle formation (Fig. 2). These results suggest that neutrophil extravasation is accompanied by increased vascular leakage and further imply that microparticle deposition by extravasating neutrophils affects vascular leakage. In contrast, inhibition of microparticle formation after depletion of intravascular inflammatory monocytes failed to prevent vascular leakage in stimulated vessels (Fig. 2). Consistently, depletion of both neutrophils and inflammatory monocytes also prevented the increase in vascular leakage during leukocyte extravasation (Fig. 2). Therefore, during leukocyte extravasation in inflammation, neutrophilderived microparticles play an important role in maintaining vascular barrier function. Interestingly, depletion of intravascular monocytes using clodronate liposomes also prevented vascular leakage regardless of extravascular inhibition of LFA-1 or not (Fig. 2). As monocytes have been shown to facilitate neutrophil trafficking (12,22), it is possible that the clodronate liposome-mediated depletion of monocytes may decrease vascular leakage by inhibiting transendothelial migration of neutrophils leading to reduced microparticle formation by extravasating neutrophils. In conclusion, these findings provide new evidence for a functional role of neutrophil-derived microparticles in preserving endothelial barrier integrity during leukocyte extravasation.
ACKNOWLEDGEMENTS
This project was financially supported through grant from the American Heart Association (11SDG7520018 to Y-M.H.). The authors have no conflicting financial interests.
References
1. Phillipson M, Heit B, Colarusso P, Liu L, Ballantyne CM, Kubes P. Intraluminal crawling of neutrophils to emigration sites: a molecularly distinct process from adhesion in the recruitment cascade. J Exp Med. 2006; 203:2569–2575. PMID: 17116736.


2. Shulman Z, Shinder V, Klein E, Grabovsky V, Yeger O, Geron E, Montresor A, Bolomini-Vittori M, Feigelson SW, Kirchhausen T, Laudanna C, Shakhar G, Alon R. Lymphocyte crawling and transendothelial migration require chemokine triggering of high-affinity LFA-1 integrin. Immunity. 2009; 30:384–396. PMID: 19268609.


3. Sumagin R, Prizant H, Lomakina E, Waugh RE, Sarelius IH. LFA-1 and Mac-1 define characteristically different intralumenal crawling and emigration patterns for monocytes and neutrophils in situ. J Immunol. 2010; 185:7057–7066. PMID: 21037096.


4. Auffray C, Fogg D, Garfa M, Elain G, Join-Lambert O, Kayal S, Sarnacki S, Lauvau CumG A, Geissmann F. Monitoring of blood vessels and tissues by a population of monocytes with patrolling behavior. Science. 2007; 317:666–670. PMID: 17673663.


5. Hyun YM, Sumagin R, Sarangi PP, Lomakina E, Overstreet MG, Baker CM, Fowell DJ, Waugh RE, Sarelius IH, Kim M. Uropod elongation is a common final step in leukocyte extravasation through inflamed vessels. J Exp Med. 2012; 209:1349–1362. PMID: 22711877.


6. Sumagin R, Kuebel JM, Sarelius IH. Leukocyte rolling and adhesion both contribute to regulation of microvascular permeability to albumin via ligation of ICAM-1. Am J Physiol Cell Physiol. 2011; 301:C804–C813. PMID: 21653902.


7. Boring L, Gosling J, Chensue SW, Kunkel SL, Farese RV Jr, Broxmeyer HE, Charo IF. Impaired monocyte migration and reduced type 1 (Th1) cytokine re sponses in C-C chemokine receptor 2 knockout mice. J Clin Invest. 1997; 100:2552–2561. PMID: 9366570.
8. Dalli J, Norling LV, Renshaw D, Cooper D, Leung KY, Perretti M. Annexin 1 mediates the rapid anti-inflammatory effects of neutrophil-derived microparticles. Blood. 2008; 112:2512–2519. PMID: 18594025.


9. Daley JM, Thomay AA, Connolly MD, Reichner JS, Albina JE. Use of Ly6G-specific monoclonal antibody to deplete neutrophils in mice. J Leukoc Biol. 2008; 83:64–70. PMID: 17884993.


10. Soehnlein O, Zernecke A, Eriksson EE, Rothfuchs AG, Pham CT, Herwald H, Bidzhekov K, Rottenberg ME, Weber C, Lindbom L. Neutrophil secretion products pave the way for inflammatory monocytes. Blood. 2008; 112:1461–1471. PMID: 18490516.


11. Woodfin A, Voisin MB, Beyrau M, Colom B, Caille D, Diapouli FM, Nash GB, Chavakis T, Albelda SM, Rainger GE, Meda P, Imhof BA, Nourshargh S. The junctional adhesion molecule JAM-C regulates polarized transendothelial migration of neutrophils in vivo. Nat Immunol. 2011; 12:761–769. PMID: 21706006.


12. Kreisel D, Nava RG, Li W, Zinselmeyer BH, Wang B, Lai J, Pless R, Gelman AE, Krupnick AS, Miller MJ. In vivo two-photon imaging reveals monocyte-dependent neutrophil extravasation during pulmonary inflammation. Proc Natl Acad Sci U S A. 2010; 107:18073–18078. PMID: 20923880.


13. Van Rooijen N, Sanders A. Liposome mediated depletion of macrophages: mechanism of action, preparation of liposomes and applications. J Immunol Methods. 1994; 174:83–93. PMID: 8083541.
14. Geissmann F, Jung S, Littman DR. Blood monocytes consist of two principal subsets with distinct migratory properties. Immunity. 2003; 19:71–82. PMID: 12871640.


15. Kim JV, Kang SS, Dustin ML, McGavern DB. Myelomonocytic cell recruitment causes fatal CNS vascular injury during acute viral meningitis. Nature. 2009; 457:191–195. PMID: 19011611.


16. Bjöork J, Hedqvist P, Arfors KE. Increase in vascular permeability induced by leukotriene B4 and the role of polymorphonuclear leukocytes. Inflammation. 1982; 6:189–200. PMID: 6179872.


17. Rosengren S, Ley K, Arfors KE. Dextran sulfate prevents LTB4-induced permeability increase, but not neutrophil emigration, in the hamster cheek pouch. Microvasc Res. 1989; 38:243–254. PMID: 2481803.


18. Wedmore CV, Williams TJ. Control of vascular permeability by polymorphonuclear leukocytes in inflammation. Nature. 1981; 289:646–650. PMID: 7464931.


19. Herwald H, Cramer H, Möorgelin M, Russell W, Sollenberg U, Norrby-Teglund A, Flodgaard H, Lindbom L, Bjöorck L. M protein, a classical bacterial virulence determinant, forms complexes with fibrinogen that induce vascular leakage. Cell. 2004; 116:367–379. PMID: 15016372.


20. Sekido N, Mukaida N, Harada A, Nakanishi I, Watanabe Y, Matsushima K. Prevention of lung reperfusion injury in rabbits by a monoclonal antibody against interleukin-8. Nature. 1993; 365:654–657. PMID: 8413628.


21. Petri B, Kaur J, Long EM, Li H, Parsons SA, Butz S, Phillipson M, Vestweber D, Patel KD, Robbins SM, Kubes P. Endothelial LSP1 is involved in endothelial dome formation, minimizing vascular permeability changes during neutrophil transmigration in vivo. Blood. 2011; 117:942–952. PMID: 21030556.


22. Maus U, von Grote K, Kuziel WA, Mack M, Miller EJ, Cihak J, Stangassinger M, Maus R, Schlöondorff D, Seeger W, Lohmeyer J. The role of CC chemokine receptor 2 in alveolar monocyte and neutrophil immigration in intact mice. Am J Respir Crit Care Med. 2002; 166:268–273. PMID: 12153956.


Figure 1
Microparticles derived from extravasating leukocytes regulate vascular leakage in an LFA-1-dependent manner. (A) The numbers of CD18+ microparticles and CD31+ cells were determined in chemokines-stimulated cremaster superfused with either the IgG control or the CD11a blocking Ab and in unstimulated cremaster. The ratio of CD18+ microparticles over CD31+ cells was calculated. MP, microparticles. *p=0.0004. **p=0.0006. (B) Vascular leakage was measured and calculated using MP-IVM from CXCL2-stimulated venules in the superfusion of control IgG or CD11a blocking Ab. Vascular leakage was averaged over five points measured outside of the venules every 30 sec based on the initial Texas Red intensity. *p<0.0001. a.u., Arbitrary unit. (C) A representative image of vascular leakage (Texas Red dextran intensity) after extravascular inhibition of LFA-1 showed significant increase in fluorescence compared to IgG. The still images were taken at 30 min of treatment with CXCL2 and the superfusion of the IgG or CD11a blocking Ab. CD18-mCFP mice were i.v. injected with Texas Red dextran, and the cremaster venules were imaged. Red: Texas Red dextran, Cyan: CD18+ leukocytes. (D & E) The cremaster venules of CD18-mCFP mice were stimulated with CXCL2 (1 nM), and the adherent cells in the vasculature were then counted before (pre-Ab: white) and 30 min after (post-Ab: black) superfusion with either the CD11a blocking Ab or control IgG (D). The extravasating leukocytes were counted in the interstitial regions surrounding stimulated venules with LFA-1 Ab or control IgG added to the superfusate (E). The number of extravasating cells was calculated per 100 µm for 30 min.
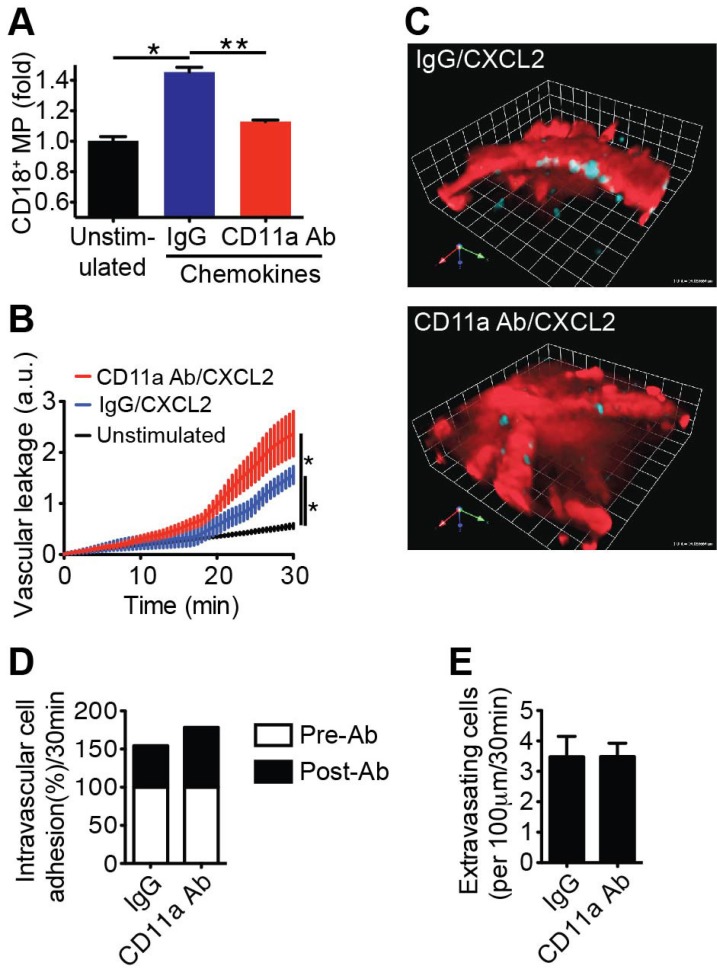
Figure 2
Microparticles derived from extravasating neutrophils regulate vascular barrier function. Vascular leakage was measured using MP-IVM from fMLP-stimulated venules of the leukocyte subset depleted mice after extravascular superfusion of either CD11a Ab or IgG control. Vascular leakage was averaged over 5 points measured outside of the venules every 30 sec based on the initial Texas Red intensity. *p<0.05, **p<0.005. The vascular leakage was then compared under each condition 30 min after stimulation. a.u., Arbitrary unit. Unstim., Unstimulated; No depl., No depletion; Neu. depl., Neutrophil depletion by i.v. injection of anti-Ly-6G Ab; Infl. Mo. depl., Inflammatory monocyte depletion in CCR2 KO mice; Neu./Infl. Mo. depl., Neutrophil and inflammatory monocyte depletion by i.v. injection of anti-Ly-6G/Ly-6C (Gr1) Ab; Mo. depl., Monocyte depletion by i.v. injection of clodronate liposomes.
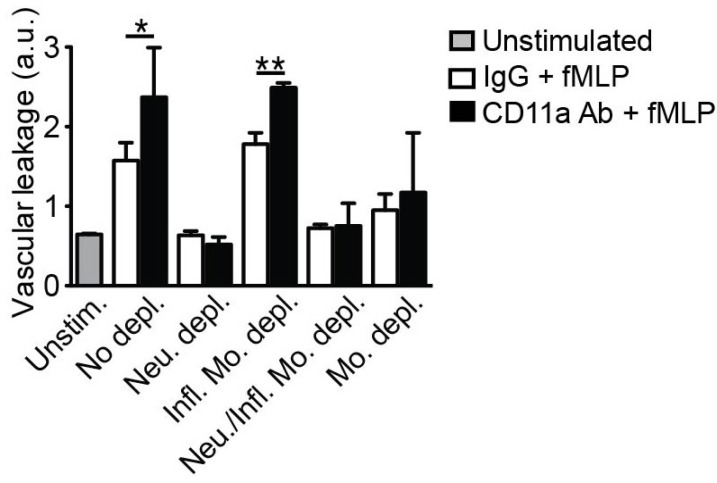