Abstract
Immune cells express toll-like receptors (TLRs) and respond to molecular patterns of various pathogens. CpG motif in bacterial DNA activates innate and acquired immune systems through binding to TLR9 of immune cells. Several studies reported that CpG can directly regulate B cell activation, differentiation, and Ig production. However, the role of CpG in B cell growth and Ig production is not fully understood. In this study, we analyzed the effect of CpG on the kinetics of mouse B cell viability, proliferation, and Igs production. Overall, CpG enhanced mouse B cell growth and production of Igs in a dose-dependent manner. Unlike LPS, 100 nM CpG (high dose) did not support TGF-β1-induced IgA and IgG2b production. Moreover, 100 nM CpG treatment abrogated either LPS-induced IgM or LPS/TGF-β1-induced IgA and IgG2b production, although B cell growth was enhanced by CpG under the same culture conditions. We subsequently found that 10 nM CpG (low dose) is sufficient for B cell growth. Again, 10 nM CpG did not support TGF-β1-induced IgA production but, interestingly enough, supported RA-induced IgA production. Further, 10 nM CpG, unlike 100 nM, neither abrogated the LPS/TGF-β1-nor the LPS/RA-induced IgA production. Taken together, these results suggest that dose of CpG is critical in B cell growth and Igs production and the optimal dose of CpG cooperates with LPS in B cell activation and differentiation toward Igs production.
Toll-like receptors (TLRs) are representative pattern recognition receptors (PRRs), which recognize specific pathogen-associated molecular patterns (PAMPs) such as bacterial diacyl/triacyl lipopeptides, peptidoglycan, lipoteichoic acid (LTA), lipopolisaccharide (LPS), flagellin, unmethylated DNA (CpG), viral ssRNA/dsRNA, and fungal zymosan. In general, it is well known that TLRs are expressed on innate immune cells, macrophages and dendritic cells, and trigger innate immune response (1). However, recent studies have revealed that PAMPs-PRRs responses link between innate and adaptive immune system (2).
It has been demonstrated that BCR cross-linking with Ag, B cell-T cell (CD40-CD40L) interaction, and optimal TLR stimulation of B cells are sufficient for B cell activation, proliferation, isotype switching, and differentiation to memory B cells and plasma cells expressing high-affinity antibodies (Abs) (3,4). On the other hand, cytokines produced by other immune cells such as T cells and macrophages directly help B cell to activate and undergo isotype switching. In addition, it has been suggested that dendritic cells (DCs) stimulation with TLR ligands, CpG and poly I:C, specifically induces APRIL production by DCs and this APRIL activates B cells in T cell-independent (TI) pathway (5).
Immunoglobulin (Ig) class switch recombination (CSR) is a mechanism that causes the production of antibodies to change from IgM or IgD to the other Ab isotypes which determine functions of humoral immunity. T cell-dependent (TD) B cell Ab responses require approximately a week, which is too much of a delay to defense fast-replicating pathogens such as pathogenic E. coli and influenza virus. To overcome this limitation, extrafollicular B cells rapidly undergo CSR, seemingly through TLR-mediated TI pathway (6). As a result, class switched-IgG, IgE, and IgA as well as IgM are produced. Consistent with the concept, it is demonstrated that naïve B cells proliferate and secrete Ab to a variety of TLR agonists in vitro, in the absence of B cell receptor cross-linking or T cell help (7). Mouse and human B cells are known to express various TLRs, TLR1~9 and TLR11 (4,7-9).
The CpG motifs of bacterial DNA, an agonist of TLR9, plays an important role in early Ab responses against pathogens. CpG DNA induces mouse and human to B cell to proliferate and secrete Ig in vitro and in vivo (10-12). In addition, CpG DNA markedly augments the proliferation and generation of plasma cells from naïve and memory B cells and dramatically increases plasma cell generation from germinal center B cells in human (13). Several studies have demonstrated that CpG directly induces expression of the transcription factor T-bet which requires CpG-induced IgG2a expression but inhibits IgG1 and IgE switching in B cells (14-16). In addition, CpG directly stimulates B cells to undergo isotype switching to IgG2a (17) and CpG redirects Igs production by mouse B cells to "Th1-like" Ig isotypes (IgG2a, IgG2b, and IgG3) while suppressing Th2 isotypes (IgG1 and IgE) (18).
Thus, CpG directly affects B cell proliferation, isotype switching, and differentiation. Nevertheless, the detailed role of CpG in B cell growth and Ig production and the cooperative effects between CpG and other TLR agonists on B cell activation is still not fully understood. In this study, we analyzed the effect of CpG on the kinetics of mouse B cell viability, proliferation, and Igs production. In essence, we found that doses of TLR9 agonist CpG and TLR4 agonist LPS are important in B cell proliferation and differentiation and that these two PAMPs can cooperate leading to Igs production.
BALB/c mice were purchased from Damool Science (Daejeon, Korea) and maintained on an 8:16 h light:dark cycle in an animal environmental control chamber. Eight- to twelve-week-old mice were used, and animal care was in accordance with the institutional guidelines of Institutional Animal Care and Use Committee of Konyang University.
Untouched mouse spleen resting B cells were obtained by depletion of CD43+ cells using anti-CD43 microbeads and high-gradient magnetic cell separation (MACS; Miltenyi Biotec, Bergisch Gladbach, Germany) according to manufacturer's instructions. Briefly, BALB/c mouse spleen cell suspensions were washed with HBSS (WelGENE, Daegu, Korea) and treated with 0.83% ammonium chloride to lyse red blood cells. Spleen cells were treated with anti-mouse CD43 microbeads and depleted with LS column and MACS Separator (Miltenyi Biotec). The purity of resting B cells (CD43-B220+) assessed by FACSCalibur (BD Biosciences, San Jose, CA, USA) following staining of the cells with anti-CD43 FITC (eBioscience, San Diego, CA, USA) and anti-B220 PE (BD Biosciences). Cells were cultured at 37℃ in a humidified CO2 incubator (Forma Scientific, Marietta, OH, USA) in RPMI-1640 medium (WelGENE) supplemented with 10% fetal bovine serum (PAA Laboratories, Etobicoke, ON, Canada). Cells were stimulated with CpG ODN1826 (Invivogen, San Diego, CA, USA), LPS (12.5µg/ml, E. coli 0127:B8, Sigma, St. Louis, MO, USA), TGF-β1 (0.2 ng/ml, R&D Systems, Minneapolis, MN, USA), and retinoic acid (20 nM, Sigma).
Cell viability was determined by EZ-Cytox cell viability assay kit (Daeil Lab Service Co, Seoul, Korea) according to the manufacturer's instructions. Briefly, 20µl of EZ-Cytox kit reagent was added to each cell cultured well of a 96-well microplate and then incubated at 37℃ in a humidified CO2 incubator for 3 h. After incubation, optical density (OD) was measured at a wavelength of 450 nm using an Absorbance Microplate Reader (BioTek Instruments, Inc., Winooski, VT, USA). For cell proliferation assay, purified mouse resting B cells were labeled with CFSE (eBioscience) and then added with stimuli (CpG, LPS, TGF-β1, and retinoic acid). Dilution of CFSE was measured by counting 10,000 cells with a FACSCalibur.
Antibodies produced in B cell cultures were detected by using isotype-specific ELISA. Affinity purified anti-isotype specific antibodies were added at 1.2µg/ml in 0.05 M sodium bicarbonate buffer (pH 9.3) to 96-well U bottomed polyvinyl microplates (Falcon, Becton Dickinson & Co., Oxnard, CA, USA). Plates were washed with PBS containing 0.05% Tween-20 (PBST) followed by overnight incubation at 4℃, and blocked for 1 h with 0.5% BSA solution. After washing, 50µl of standard myeloma proteins and culture supernatants were added to each well and incubated for 1 h at 37℃. After washing, horseradish-peroxidase (HRPO) conjugated anti-isotype specific antibodies (Southern Biotechnology, Birmingham, AL, USA) were added to each well and incubated for 1 h. Plates were then washed and TMB substrate (BD Biosciences) was added. After incubation, 0.05 M sulfuric acid was added to each well and colorimetric reaction was measured at 450 nm with an Absorbance Microplate Reader.
To elucidate the direct effect of CpG on B cell activation and differentiation, we used untouched resting B cells (CD43-B220+) from mouse spleen in this study. As shown in Fig. 1A, purity of the resting B cells assessed by flow cytometric analysis was higher than 97%. To determine the basic property of CpG in mouse B cell activation, we first examined the effect of CpG on mouse B cell growth. As shown in Fig. 1B, CpG constantly increased cell growth up to 2 days and dose-dependently sustained cell viability on day 3 of culture. Overall cell viability on day 3 was lower than day 2. This seems due to the exhaustion of nutrients in the culture media. In addition, we observed that CpG induces cell proliferation in a dose-dependent manner (Fig. 1C). Thus, CpG can act as a potent B cell mitogen and this is consistent with an earlier report that CpG induces B cell cycle entry and cell survival (19). Since CpG strongly increased B cell proliferation, it was important to explore whether it affects Igs production. As shown in Fig. 1D, CpG dose-dependently increased all Ig isotypes production and this function was consistent with results from cell growth.
LPS is a well-known mitogen for mouse B cells and a stimulant for TLR4 (20,21). Therefore, we next compared the effects of CpG and LPS on B cell growth and Igs production. Along with LPS, we adopted TGF-β1 to determine whether CpG can support TGF-β1-induced IgA expression. TGF-β1 is a well-known IgA class switching factor in mouse B cells (22,23). Here, we used 100 nM CpG because this dosage showed great B cell viability and proliferation as shown in Fig. 1B and C. CpG enhanced cell viability and proliferation and this effect was greater than that of LPS on day 2 of culture (Fig. 2A and B). In addition, we found that these two stimuli have neither additive nor synergetic effect on cell viability. As previously shown by other (24), TGF-β1 markedly inhibited the increase of cell viability and proliferation caused by LPS on day 2 of culture (Fig. 2A and B). However, TGF-β1 did not inhibit CpG-induced cell viability and had a little effect on inhibiting CpG-induced cell proliferation. This different effect of TGF-β1 between LPS and CpG is likely to arise because mitogenic potency of CpG is greater than that of LPS. As for Ig production, CpG was less stimulatory than LPS, especially IgM and IgG2b isotypes (Fig. 2C). Interestingly, CpG decreased LPS-induced IgM production (Fig. 2C) although the cell viability and proliferation were not decreased under the same conditions (Fig. 2A and B). On the other hand, CpG was unable to support TGF-β1-induced IgA and IgG2b production (Fig. 2C). This result is sharply contrasted to that of LPS. In addition, CpG also down-regulated TGF-β1-induced IgA/IgG2b production in the presence of LPS. We assumed that this effect of CpG is attributed to its excessive mitogenic property. To resolve these unexpected results, we decided to test low doses of CpG in cell viability and cell proliferation. As shown in Fig. 3, we observed that 10 nM CpG looks sufficient for supporting cell viability and proliferation. Therefore, 10 nM CpG was used for further experiments.
Next, we tested the effect of 10 nM CpG on Igs production in parallel with LPS. We used TGF-β1 and retinoic acid (RA) as anti-proliferative agents to alleviate the excessive proliferative activity of CpG. It was reported that RA specifically induces IgA expression (25,26). As shown in Fig. 4, 10 nM CpG was still less stimulatory than LPS in IgM and IgA production as seen in high concentration of CpG. LPS supported TGF-β1-induced IgA secretion but not CpG (Fig. 4). Interestingly, CpG supported RA-induced IgA production. This different action of CpG may be caused by different anti-proliferative effects of TGF-β1 and RA to properly regulate B cell growth and differentiation into cells producing Abs. In addition, unlike 100 nM CpG, 10 nM CpG did not reduce LPS/TGF-β1-induced IgA production. Moreover, when RA was added on day 0, CpG substantially increased LPS/RA-induced IgA production (Fig. 4). These results suggest that CpG can regulate the mouse B cell proliferation and differentiation and that optimum dosage of CpG can cooperate with LPS to produce Igs.
In the present study, we found that CpG, a TLR9 stimulant, shows a powerful mitogenic potency for mouse spleen B cells, which was greater than LPS, a well-known mouse B cell polyclonal activator. Nevertheless, CpG was less stimulatory than LPS in all Ig isotypes production. Surprisingly, CpG down-regulated the LPS-stimulated Igs production. It is assumed that this function of CpG is attributed to the excessive mitogenic property. When low concentration of CpG was used, it supported RA-induced IgA production and did not down-regulate LPS/TGF-β1-induced IgA production. Moreover, CpG further augmented LPS/RA-induced IgA production. In conclusion, our results indicate that the optimal dose of TLR9 agonist CpG and TLR4 agonist LPS cooperate to direct stimulate B cells to proliferate and differentiate into cells producing Igs.
ACKNOWLEDGMENTS
This research was supported by Basic Science Research Program through the National Research Foundation of Korea (NRF) funded by the Ministry of Education, Science and Technology (MEST) (No. 2010-0025648) and partially supported by the grant of Myunggok Medical Research Institute in Konyang University. Y.-H.K. is an awardee of fellowship provided by Gang-Won Human Resources Development Foundation (gangwon mirae injae).
References
1. Akira S, Uematsu S, Takeuchi O. Pathogen recognition and innate immunity. Cell. 2006; 124:783–801. PMID: 16497588.


2. Palm NW, Medzhitov R. Pattern recognition receptors and control of adaptive immunity. Immunol Rev. 2009; 227:221–233. PMID: 19120487.


3. Ruprecht CR, Lanzavecchia A. Toll-like receptor stimulation as a third signal required for activation of human naive B cells. Eur J Immunol. 2006; 36:810–816. PMID: 16541472.


4. Pone EJ, Xu Z, White CA, Zan H, Casali P. B cell TLRs and induction of immunoglobulin class-switch DNA recombination. Front Biosci. 2012; 17:2594–2615. PMID: 22652800.


5. Hardenberg G, Planelles L, Schwarte CM, van Bostelen L, Le Huong T, Hahne M, Medema JP. Specific TLR ligands regulate APRIL secretion by dendritic cells in a PKR-dependent manner. Eur J Immunol. 2007; 37:2900–2911. PMID: 17899538.


6. Fagarasan S, Honjo T. T-Independent immune response: new aspects of B cell biology. Science. 2000; 290:89–92. PMID: 11021805.


7. Gururajan M, Jacob J, Pulendran B. Toll-like receptor expression and responsiveness of distinct murine splenic and mucosal B-cell subsets. PLoS One. 2007; 2:e863. PMID: 17848994.


8. Peng SL. Signaling in B cells via Toll-like receptors. Curr Opin Immunol. 2005; 17:230–236. PMID: 15886111.


9. Bekeredjian-Ding I, Jego G. Toll-like receptors--sentries in the B-cell response. Immunology. 2009; 128:311–323. PMID: 20067531.
10. Krieg AM, Yi AK, Matson S, Waldschmidt TJ, Bishop GA, Teasdale R, Koretzky GA, Klinman DM. CpG motifs in bacterial DNA trigger direct B-cell activation. Nature. 1995; 374:546–549. PMID: 7700380.


11. Hartmann G, Krieg AM. Mechanism and function of a newly identified CpG DNA motif in human primary B cells. J Immunol. 2000; 164:944–953. PMID: 10623843.


12. Hartmann G, Weeratna RD, Ballas ZK, Payette P, Blackwell S, Suparto I, Rasmussen WL, Waldschmidt M, Sajuthi D, Purcell RH, Davis HL, Krieg AM. Delineation of a CpG phosphorothioate oligodeoxynucleotide for activating primate immune responses in vitro and in vivo. J Immunol. 2000; 164:1617–1624. PMID: 10640783.
13. Jung J, Yi AK, Zhang X, Choe J, Li L, Choi YS. Distinct response of human B cell subpopulations in recognition of an innate immune signal, CpG DNA. J Immunol. 2002; 169:2368–2373. PMID: 12193703.


14. Liu N, Ohnishi N, Ni L, Akira S, Bacon KB. CpG directly induces T-bet expression and inhibits IgG1 and IgE switching in B cells. Nat Immunol. 2003; 4:687–693. PMID: 12766768.


15. Peng SL, Li J, Lin L, Gerth A. The role of T-bet in B cells. Nat Immunol. 2003; 4:1041. PMID: 14586416.


16. Rifkin IR, Marshak-Rothstein A. T-bet: the Toll-bridge to class-switch recombination? Nat Immunol. 2003; 4:650–652. PMID: 12830144.


17. Jegerlehner A, Maurer P, Bessa J, Hinton HJ, Kopf M, Bachmann MF. TLR9 signaling in B cells determines class switch recombination to IgG2a. J Immunol. 2007; 178:2415–2420. PMID: 17277148.


18. Lin L, Gerth AJ, Peng SL. CpG DNA redirects class-switching towards "Th1-like" Ig isotype production via TLR9 and MyD88. Eur J Immunol. 2004; 34:1483–1487. PMID: 15114682.


19. Yi AK, Chang M, Peckham DW, Krieg AM, Ashman RF. CpG oligodeoxyribonucleotides rescue mature spleen B cells from spontaneous apoptosis and promote cell cycle entry. J Immunol. 1998; 160:5898–5906. PMID: 9637502.
20. Janossy G, Snajdr J, Simak-Ellis M. Patterns of B-lymphocyte gene expression elicited by lipopolysaccharide mitogen. Immunology. 1976; 30:799–810. PMID: 1088414.
21. Poltorak A, He X, Smirnova I, Liu MY, Van Huffel C, Du X, Birdwell D, Alejos E, Silva M, Galanos C, Freudenberg M, Ricciardi-Castagnoli P, Layton B, Beutler B. Defective LPS signaling in C3H/HeJ and C57BL/10ScCr mice: mutations in Tlr4 gene. Science. 1998; 282:2085–2088. PMID: 9851930.
22. Kim PH, Kagnoff MF. Transforming growth factor beta 1 increases IgA isotype switching at the clonal level. J Immunol. 1990; 145:3773–3778. PMID: 2246513.
23. Coffman RL, Lebman DA, Shrader B. Transforming growth factor beta specifically enhances IgA production by lipopolysaccharide-stimulated murine B lymphocytes. J Exp Med. 1989; 170:1039–1044. PMID: 2788703.


24. Lebman DA, Edmiston JS. The role of TGF-beta in growth, differentiation, and maturation of B lymphocytes. Microbes Infect. 1999; 1:1297–1304. PMID: 10611758.
25. Tokuyama H, Tokuyama Y. Endogenous cytokine expression profiles in retinoic acid-induced IgA production by LPS-stimulated murine splenocytes. Cell Immunol. 1995; 166:247–253. PMID: 7497526.


26. Tokuyama Y, Tokuyama H. Retinoids as Ig isotype-switch modulators. The role of retinoids in directing isotype switching to IgA and IgG1 (IgE) in association with IL-4 and IL-5. Cell Immunol. 1996; 170:230–234.
Figure 1
Effect of CpG on mouse B cell growth and Igs production. (A) Purified mouse spleen resting B cell population (CD43-B220+) was measured by flow cytometric analysis. Data shown are representative of all the experiments carried out in this study. (B) Resting B cells were stimulated with the indicated doses of CpG (0 nM, open triangle; 20 nM, closed triangle; 40 nM, open circle; 60 nM, closed circle; 80 nM, open square; 100 nM, closed square). After 2 and 3 days of culture, cell viability was measured by EZ-Cytox cell viability assay. Data are averages of duplicate samples with ranges (bars). (C) Culture conditions were the same as in (B). After 2 and 3 days of culture, cell proliferation was measured by the dilution of CFSE. The percentage indicates cells (%) with low level of CFSE intensity. (D) Culture conditions were the same as in (B). After 7 days of culture, supernatants were harvested and the levels of Igs production were determined by isotype-specific ELISA. Data represent means±SEM of triplicate samples.
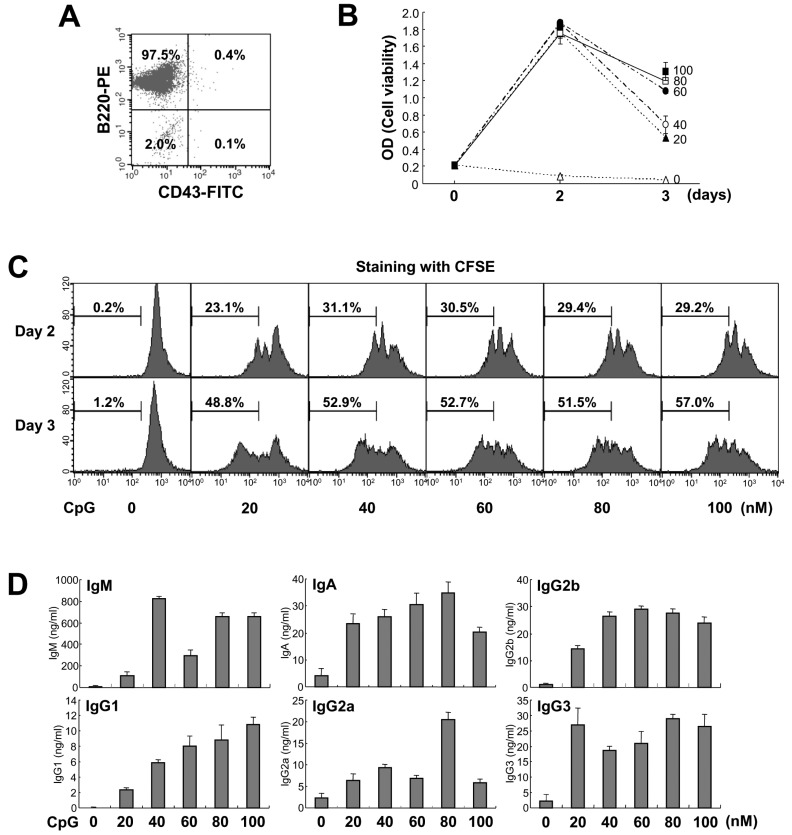
Figure 2
Comparison of effects of CpG and LPS on B cell growth and Igs production. Mouse spleen resting B cells were stimulated with CpG (100 nM), LPS (12.5µg/ml), and TGF-β1 (0.2 ng/ml). (A) After 2 and 3 days of culture, cell viability was measured by EZ-Cytox cell viability assay. Data are averages of duplicate samples with ranges (bars). (B) After 2 and 3 days of culture, cell proliferation was measured by the dilution of CFSE. (C) After 7 days of culture, supernatants were harvested and the levels of Igs production were determined by isotype-specific ELISA. Data represent means±SEM of triplicate samples. *p<0.05.
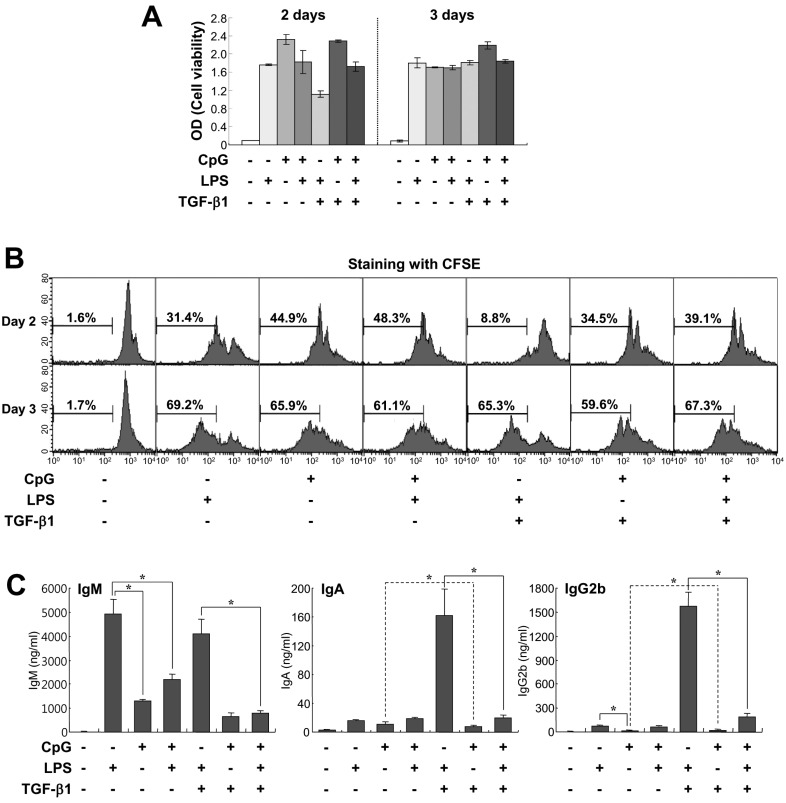
Figure 3
Effects of lower doses of CpG on B cell viability and proliferation. Mouse spleen resting B cells were stimulated with the indicated doses of CpG (0 nM, open circles; 2.5 nM, closed triangles; 5 nM, open squares; 10 nM, closed circles; 20 nM, open triangles; 40 nM, closed squares). After 1, 2, 3, and 4 days of culture, cell viability was measured by EZ-Cytox cell viability assay (A), and cell proliferation was measured by the dilution of CFSE (B). CFSE intensity (%) of panel B represents the percentage of cells with a low level of CFSE intensity. Data are averages of duplicate samples with ranges (bars).
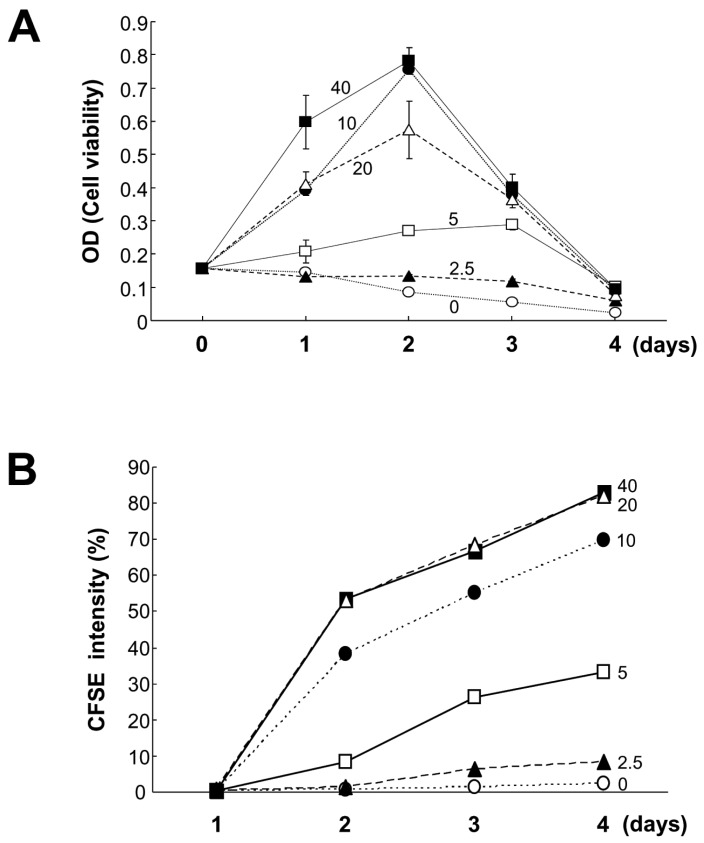
Figure 4
Effect of low dose CpG on TGF-β1- and RA-induced Igs production. Mouse spleen resting B cells were stimulated with CpG (10 nM) and LPS (12.5µg/ml). TGF-β1 (0.2 ng/ml) and retinoic acid (RA, 20 nM) were added on day 0 (D0) or day 1 (D1). After 7 days of culture, supernatants were harvested and the levels of IgM and IgA production were determined by isotype-specific ELISA. Data represent means±SEM of triplicate samples. *p<0.05.
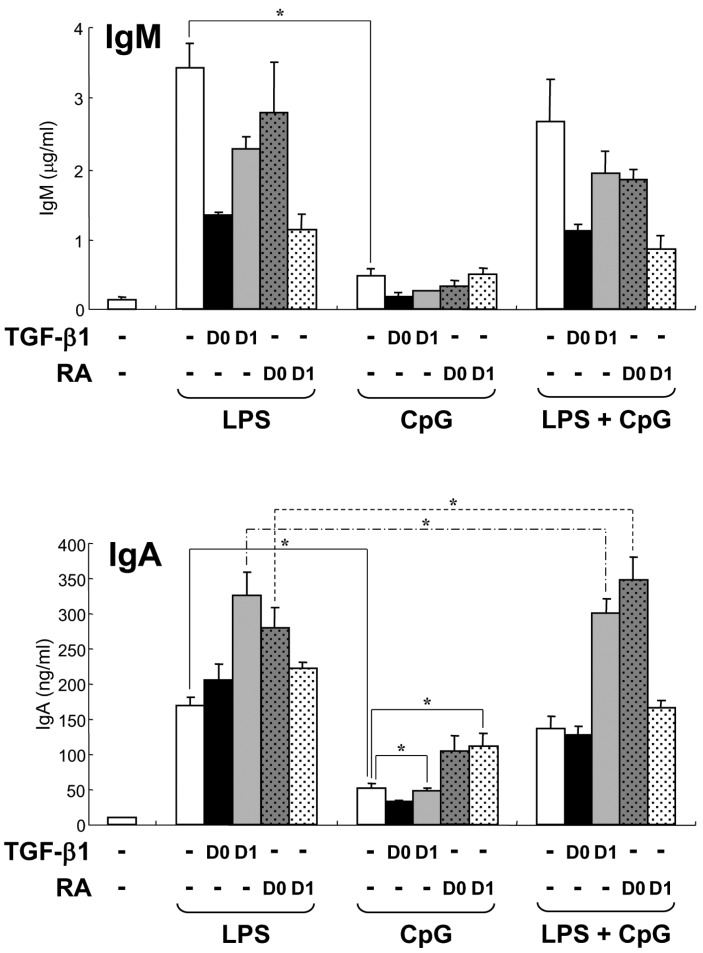