Abstract
Innate immune cells sense and respond to the cytoplasmic infection of bacterial pathogens through NLRP3, NLRC4 or AIM2 inflammasome depending on the unique molecular pattern of invading pathogens. The infection of flagellin- or type III secretion system (T3SS)-containing Gram-negative bacteria such as Salmonella enterica serovar Typhimurium (S. typhimurium) or Pseudomonas aeruginosa (P. aeruginosa) triggers NLRC4-dependent caspase-1 activation leading to the secretion of proinflammatory cytokines such as interleukin-1-beta (IL-1β) and IL-18. Previous studies have shown that apoptosis-associated speck-like protein containing a CARD (ASC) is also required for Salmonella-induced caspase-1 activation, but it is still unclear how ASC contributes to the activation of NLRC4 inflammasome in response to S. typhimurium infection. In this study, we demonstrate that S. typhimurium triggers the formation of ASC oligomer in a potassium depletion-independent manner as determined by in vitro crosslinking and in situ fluorescence imaging. Remarkably, inhibition of potassium efflux failed to block Salmonella-promoted caspase-1 activation and macrophage cell death. These results collectively suggest that ASC is substantially oligomerized to facilitate the activation of caspase-1 in response to S. typhimurium infection. Contrary to NLRP3 inflammasome, intracellular potassium depletion is not critical for NLRC4 inflammasome signaling by S. typhimurium.
Inflammasome is a cytoplasmic multiprotein complex mediating the maturation of inactive procaspase-1 into active caspase-1 and the subsequent processing of proinflammatory cytokines interleukin-1 beta (IL-1β) and IL-18 (1). Assembly of inflammasome complex is initiated when the upstream pyrin domain (PYD)- or caspase recruitment domain (CARD)-containing nucleotide-bindng oligomerization domain (NOD)-like receptors (NLRs) or absent in melanoma 2 (AIM2) sense abnormal cytoplasmic molecular patterns derived from microbes or endogenous danger signal (2). Then, PYD-containing NLRs or AIM2 triggers the oligomerization of apoptosis-associated speck-like protein containing a CARD (ASC) to form ASC pyroptosome, resulting in caspase-1 activation and pyroptotic cell death (3,4). However, it is still unclear whether ASC and its oligomerization are required for CARD-containing NLRC4 inflammasome activation.
Accumulating evidences have proposed that inflammasome plays a pivotal role in host defense against a wide range of microbial pathogens by recognizing a unique pattern of microbes and by promoting caspase-1/IL-1β signaling pathway (5,6). For example, NLRP3 inflammasome is activated by a variety of microbial infection such as Listeria monocytogenes, Staphylococcus aureus, influenza virus and Candida albicans, but the detailed cytoplasmic pathogen-associated molecular pattern (PAMP) to activate NLRP3 is poorly characterized (5,6). On the other hand, it has been clarified that both bacterial flagellin and type III secretion system (T3SS) are important for the activation of NLRC4 inflammasome upon infected mainly with Gram-negative bacteria including Salmonella typhimurium and Pseudomonas aeruginosa (7-9). In addition, specific neuronal apoptosis inhibitor protein (Naip) family recognizes bacterial flagellin or T3SS rod component such as PrgJ and facilitates the activation of NLRC4 inflammasome (10,11).
NLRP3 associates with ASC through the PYD homotypic interaction to form NLRP3 inflammasome in response to a wide range of pathogen infections or danger signals released from tissue injury (5). In the case of NLRP3 inflammasome, ASC oligomerization is the critical step for caspase-1 activation, IL-1β secretion and pyroptotic cell death (2). Moreover, potassium efflux is necessary for NLRP3- or AIM2-dependent ASC oligomerization and the subsequent inflammasome activation (3,12). In contrast to NLRP3 or AIM2, NLRC4 contains CARD domain, which associates directly and specifically with procaspase-1 through CARD-CARD interaction (13). In this regard, the initial study demonstrated that overexpression of truncated NLRC4 promoted the robust caspase-1 activation in the absence of ASC (13). However, ASC-deficient macrophages showed a defect in caspase-1 activation in response to S. typhimurium infection (14,15). It was also reported that S. typhimurium infection did not promote caspase-1 activation but caused a normal cell death in ASC-deficient macrophages (14,16). Thus, it is still elusive how ASC is implicated in the NLRC4-dependent caspase-1 activation. In this study, we examined whether ASC oligomerization is required for NLRC4 inflammasome activation by S. typhimurium infection.
Bone marrow cells were isolated from femurs of C57BL/6J mice and differentiated for one week in a L929-conditioned DMEM/F-12 medium supplemented with 10% FBS. To induce NLRP3 inflammasome activation, bone marrow derived macrophages (BMDMs) were primed with LPS (0.25 µg/ml) for 4 h and treated with nigericin. THP-1-ASC-GFP cells were grown in RPMI 1640 supplemented with 10% FBS, 2 mM L-glutamine, 10 mM HEPES, 1 mM sodium pyruvate, 1.5 g/l sodium bicarbonate and 0.05 mM 2-mercaptoethanol. Before treatment or infection, THP-1-ASC-GFP cells were primed with phorbol-12-myristate-13-acetate (PMA) (0.4 µM, 3 h) and grown for 16 h.
S. typhimurium (kindly gifted from Dr. Yoon SS, Yonsei University College of Medicine) were grown overnight at 37℃ with aeration, and then diluted (1:20) and grown for additional 2 h. Murine BMDMs or human THP-1 cells stably expressing ASC-GFP (THP-1-ASC-GFP) were infected with S. typhimurium at the indicated MOI (multiplicity of infection) for 30 min, washed three times to remove extracellular bacteria, and incubated with the gentamicin (100 µg/ml)-containing medium for 150 min before harvest.
Anti-mouse/human caspase-1 antibody was obtained from Santa Cruz (Santa Cruz, CA) or kindly gifted from Dr. Emad Alnemri (Thomas Jefferson University). Anti-mouse/human IL-1β antibody was purchased from R&D (Minneapolis, MN) and Cell Signaling (Beverly, MA). Anti-ASC antibody was from Santa Cruz. LPS, nigericin and glybenclamide were obtained from Sigma (St. Louis, MO). z-VAD-fluoromethylketone was from Bachem (Torrence, CA) or BD (San Jose, CA). All the culture medium and supplements were purchased from Invitrogen (Grand Isalnd, NY).
Harvested cells were lysed in a buffer containing 20 mM HEPES, pH 7.5, 0.5% NP-40, 50 mM KCl, 150 mM NaCl, 1.5 mM MgCl2, 1 mM EGTA and protease inhibitors. Cell lysates were fractionated on SDS-PAGE, transferred onto PVDF membranes (Bio-Rad), and then Western-blotted using appropriate antibodies as indicated. Cell culture supernatants were precipitated by methanol/chloroform as described previously (4,17) and then immunoblotted with appropriate antibodies.
Chemical crosslinking was performed using discuccinimidyl suberate (DSS) as described previously (18). Precipitated pellets and soluble lysates were simultaneously immunoblotted using anti-ASC antibody. To quantify in situ ASC oligomerization, ASC specks were counted in THP-1-ASC-GFP cells using a fluorescence microscope (Olympus IX71/DP71). More than 150 cells were counted for ASC speck formation in each well.
ASC oligomerization is essential for NLRP3- or AIM2-dependent caspase-1 activation, IL-1β secretion and pyroptosis (3,4). Although the detailed mechanism is not well understood, intracellular K+ depletion is a critical event for ASC oligomerization and ASC-dependent caspase-1 activation (3,19). Indeed, the inhibition of K+ efflux completely blocks NLRP3- or AIM2-mediated caspase-1 activation triggered by pore-forming toxin, alum crystal, cytoplasmic DNA and Francisella tularensis infection (12,19,20).
To explore whether S. typhimurium infection induces ASC oligomerization-dependent caspase-1 activation, we first examined the effect of K+ efflux on the caspase-1 activation by S. typhimurium infection in mouse BMDMs. Salmonella-induced caspase-1 activation was clearly inhibited by pan caspase inhibitor zVAD (Fig. 1A). Seemingly, prevention of K+ efflux by raising extracellular K+ concentration as 120 mM partially attenuated Salmonella-induced caspase-1 activation (Fig. 1A). However, an inhibitor of ATP-sensitive K+ channels, glybenclamide, failed to reduce caspase-1 activation by S. typhimurium infection.
In contrast, inhibition of K+ efflux by increasing extracellular K+ concentration or glybenclamide completely suppressed caspase-1 maturation and IL-1β secretion in response to nigericin, a well-known K+ ionophore to stimulate NLRP3 inflammasome (15) (Fig. 1B). These observations suggest that K+ efflux is critical for the activation of NLRP3 inflammasome, but not of NLRC4 inflammasome, at least by S. typhimurium infection.
To clarify the differential role of K+ efflux in the NLRC4- or NLRP3-induced caspase-1 activation, we determined the ASC oligomerization triggered by both inflammasomes. Nigericin, a NLRP3 inflammasome stimulator, induced a robust ASC oligomerization in BMDMs as determined by crosslinking experiment (Fig. 2A). This ASC oligomerization was not abrogated by zVAD, which clearly attenuated caspase-1 activation by nigericin, indicating that ASC oligomerization is an upstream event for caspase-1 processing. Inhibition of K+ efflux by increasing extracellular K+ concentration or glybenclamide efficiently blocked nigericin-induced ASC oligomerization as expected (Fig. 2A).
Next, we determined whether ASC is substantially oligomerized upon S. typhimurium infection. Similar to nigericin stimulation, S. typhimurium also induced the oligomerization of ASC, although it was weaker than nigericin (Fig. 2B). Interestingly, glybenclamide treatment did not reduce either ASC oligomerization or caspase-1 activation in response to S. typhimurium infection (Fig. 2B). Instead, prevention of K+ efflux by glybenclamide slightly increased Salmonella-mediated ASC oligomerization and caspase-1 processing. These data suggest that ASC is oligomerized to facilitate the activation of NLRC4 inflammasome upon S. typhimurium infection, but K+ efflux is dispensable not only for caspase-1 activation but for ASC oligomerization by S. typhimurium.
To verify this observation, we infected human monocytic THP-1-ASC-GFP cells with S. typhimurium. In consistency with the above results in mouse BMDMs, S. typhimurium promoted the intracellular formation of ASC specks, previously termed as ASC pyroptosome (3), as well as the subsequent secretion of active caspase-1 and IL-1β (Fig. 3A). Prevention of K+ efflux by glybenclamide significantly attenuated the formation of ASC speck in response to nigericin, but not to S. typhimurium infection (Fig. 3B). These findings verified the differential role of K+ efflux in the NLRP3- or NLCR4-dependent ASC oligomerization.
Previous studies have shown that S. typhimurium infection induced caspase-1- and NLRC4-dependent pyroptotic cell death (8,16). However, Salmonella-induced cell death was not abrogated in ASC-deficient macrophages, indicating ASC is essential for caspase-1 activation but dispensable for pyroptosis in response to S. typhimurium. Here, we examined whether K+ depletion might affect Salmonella-induced cell death by measuring LDH release into extracellular medium. S. typhimurium infection caused a relatively weaker ASC oligomerization compared to nigericin treatment (Fig. 3). Unlike the above result, S. typhimurium infection and nigericin stimulation were equipotent in promoting macrophage cell death (Fig. 4), indicating that ASC oligomerization is not directly correlated with the macrophage cell death by S. typhimurium. In addition, prevention of K+ efflux by raising extracellular K+ concentration or glybenclamide showed no modulatory effect on macrophage cell death by S. typhimurium infection (Fig. 4A and C). However, nigericin-promoted cell death was significantly reduced by the inhibition of K+ efflux (Fig. 4B and C). These data indicate that intracellular K+ depletion plays a critical role in macrophage cell death by NLRP3 inflammasome, but not by Salmonella-promoted NLRC4 inflammasome.
Bacterial flagellin and T3SS rod proteins are detected by NLRC4 to assemble NLRC4 inflammasome complex mediating caspase-1 activation (7,8,11), although it is poorly clarified whether ASC is also assembled with the NLRC4 inflammasome. Nonetheless, it is evident that both NLRC4 and ASC are critical for Salmonella-mediated caspase-1 activation. A recent study proposed that NLRP3 is also responsible for sensing Salmonella through a flagellin-independent manner and both NLRP3 and NLRC4 triggers ASC-dependent caspase-1 activation (21).
In this study, we demonstrated that S. typhimurium promoted ASC oligomerization. However, ASC oligomerization by S. typhimurium was less potent than that by NLRP3-stimulating nigericin (Fig. 2 and 3). Considering that NLRP3 could also be activated by S. typhimurium infection (21), it is inferred that this weaker ASC oligomerization is only through PYD-containing NLRP3, but not through CARD-containing NLRC4. However, it was also demonstrated that the formation of ASC oligomerized foci by S. typhimurium infection was partially impaired not only in Nlrp3-/- but in Nlrc4-/- macrophages (21), indicating both NLR proteins might contribute to the ASC oligomerization. It remains thus to be clarified in detail which NLR protein is engaged in the ASC oligomerization by S. typhimurium and how NLRP3 recognizes S. typhimurium infection.
Interestingly, Salmonella-induced ASC oligomerization was independent of intracellular K+ depletion (Fig. 2 and 3). Previous studies have shown that Salmonella-induced caspase-1 activation was not abrogated by raising extracellular K+ concentration (22). In contrast, one recent report demonstrated that high extracellular K+ concentration as 140 mM prevented caspase-1 activation upon S. typhimurium infection (23). In our data, 120 mM KCl, but not 60 mM KCl, partially attenuated caspase-1 activation by S. typhimurium, but glybenclamide, another inhibitor of K+ efflux, did not reduce Salmonella-induced caspase-1 activation and ASC oligomerization. Based on these observations, K+ depletion is not likely to contribute to the activation of NLRC4 inflammasome by S. typhimurium. It is possible that extremely high concentration of K+ in extracellular medium might regulate caspase-1 maturation in a NLRC4 inflammasome-independent manner. In addition, prevention of K+ efflux had no effect on macrophage cell death by S. typhimurium.
In this study, our results demonstrate that NLRC4 inflammasome signaling pathway in response to S. typhimurium is ASC oligomerization-dependent, but intracellular K+ depletion-independent. Considering that glybenclamide, an inhibitor of K+ efflux, has been used to treat non-insulin dependent type II diabetes, the deregulation of K+ efflux-sensitive NLRP3 inflammasome activity might be implicated in the progression of metabolic diseases. By contrast, K+ efflux-independent NLRC4 inflammasome seems to provide a host innate immune defense against pathogenic bacterial infection.
Figures and Tables
Figure 1
Prevention of potassium efflux blocks the caspase-1 activation by nigericin, but not by Salmonella. (A) LPS-primed BMDMs were untreated or pretreated with zVAD (10 µM), KCl or glybenclamide (glyben, 50 µM) for 30 min as indicated, and then infected with S. typhimurium (MOI 30) as described in Materials and methods. Cultural supernatants (Sup) or soluble lysates (Lys) were immunoblotted with anti-caspase-1 antibody. (B) LPS-primed BMDMs were pretreated with the indicated inhibitors as in (A) and stimulated with nigericin (Niger, 5 µM) for 45 min. Supernatants or lysates were immunoblotted with the appropriate antibodies as indicated.
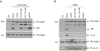
Figure 2
Prevention of potassium efflux blocks the ASC oligomerization by nigericin, but not by Salmonella. (A) LPS-primed BMDMs were pretreated with the indicated inhibitors and stimulated with nigericin (5 µM) for 45 min. The cross-linked pellets (Pell), soluble lysates (Lys) and supernatants (Sup) were immunoblotted with anti-caspase-1 or anti-ASC antibodies. (B) LPS-primed BMDMs were infected with S. typhimurium (Sal, MOI 30) or treated with nigericin (5 µM) in the presence or absence of glybenclamide, and assayed as described in (A).
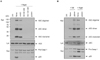
Figure 3
Potassium efflux-independent ASC oligomerization by Salmonella infection. (A) PMA-primed THP-1-ASC-GFP cells were infected with S. typhimurium (Sal) at a MOI of 10 or 30 or stimulated with nigericin (10 µM). The number of ASC pyroptosome was counted using a fluorescent microscope and the ASC speck-containing cells were represented as a relative percentage compared to the total cell number. Cultural supernatants or lysates were immunoblotted with anti-caspase-1 or anti-IL-1β antibodies. (B) PMA-primed THP-1-ASC-GFP cells were infected with S. typhimurium (MOI 30) or treated with nigericin (5µM), and assayed as described in (A). The asterisk indicates a significant difference compared to only nigericin-treated cells (n=3, p<0.05).
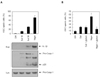
Figure 4
Differential effect of potassium efflux on inflammasome-mediated macrophage cell death. LPS-primed mouse BMDMs were infected with S. typhimurium (MOI 30, A and C) or stimulated with nigericin (5 µM, B and C) together with the pretreatment of the indicated inhibitors. Cell death was determined by LDH release into extracellular medium as described in Materials and methods. Asterisks indicate significant differences compared to only nigericin-treated cells (B, n=3, p<0.005; C, n=3, p<0.001).
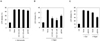
ACKNOWLEDGEMENTS
This work was supported in part by Basic Science Research Program through NRF funded by the MEST (2010-10849), by the National R&D program for Cancer Control, Ministry for Health, Welfare and Family affairs, Rep of Korea (1020190), and by a new faculty research seed money grant of Yonsei University College of Medicine for 2012 (8-2012-0066).
References
1. Strowig T, Henao-Mejia J, Elinav E, Flavell R. Inflammasomes in health and disease. Nature. 2012. 481:278–286.


2. Hong S, Park S, Yu JW. Pyrin domain (PYD)-containing inflammasome in innate immunity. J Bacteriol Virol. 2011. 41:133–146.


3. Fernandes-Alnemri T, Wu J, Yu JW, Datta P, Miller B, Jankowski W, Rosenberg S, Zhang J, Alnemri ES. The pyroptosome: a supramolecular assembly of ASC dimers mediating inflammatory cell death via caspase-1 activation. Cell Death Differ. 2007. 14:1590–1604.


4. Fernandes-Alnemri T, Yu JW, Datta P, Wu J, Alnemri ES. AIM2 activates the inflammasome and cell death in response to cytoplasmic DNA. Nature. 2009. 458:509–513.


5. Elinav E, Strowig T, Henao-Mejia J, Flavell RA. Regulation of the antimicrobial response by NLR proteins. Immunity. 2011. 34:665–679.


6. Franchi L, Muñoz-Planillo R, Núñez G. Sensing and reacting to microbes through the inflammasomes. Nat Immunol. 2012. 13:325–332.


7. Miao EA, Alpuche-Aranda CM, Dors M, Clark AE, Bader MW, Miller SI, Aderem A. Cytoplasmic flagellin activates caspase-1 and secretion of interleukin 1beta via Ipaf. Nat Immunol. 2006. 7:569–575.


8. Franchi L, Amer A, Body-Malapel M, Kanneganti TD, Ozören N, Jagirdar R, Inohara N, Vandenabeele P, Bertin J, Coyle A, Grant EP, Núñez G. Cytosolic flagellin requires Ipaf for activation of caspase-1 and interleukin 1beta in salmonella-infected macrophages. Nat Immunol. 2006. 7:576–582.


9. Miao EA, Mao DP, Yudkovsky N, Bonneau R, Lorang CG, Warren SE, Leaf IA, Aderem A. Innate immune detection of the type III secretion apparatus through the NLRC4 inflammasome. Proc Natl Acad Sci U S A. 2010. 107:3076–3080.


10. Kofoed EM, Vance RE. Innate immune recognition of bacterial ligands by NAIPs determines inflammasome specificity. Nature. 2011. 477:592–595.


11. Zhao Y, Yang J, Shi J, Gong YN, Lu Q, Xu H, Liu L, Shao F. The NLRC4 inflammasome receptors for bacterial flagellin and type III secretion apparatus. Nature. 2011. 477:596–600.


12. Fernandes-Alnemri T, Yu JW, Juliana C, Solorzano L, Kang S, Wu J, Datta P, McCormick M, Huang L, McDermott E, Eisenlohr L, Landel CP, Alnemri ES. The AIM2 inflammasome is critical for innate immunity to Francisella tularensis. Nat Immunol. 2010. 11:385–393.


13. Poyet JL, Srinivasula SM, Tnani M, Razmara M, Fernandes-Alnemri T, Alnemri ES. Identification of Ipaf, a human caspase-1-activating protein related to Apaf-1. J Biol Chem. 2001. 276:28309–28313.


14. Mariathasan S, Newton K, Monack DM, Vucic D, French DM, Lee WP, Roose-Girma M, Erickson S, Dixit VM. Differential activation of the inflammasome by caspase-1 adaptors ASC and Ipaf. Nature. 2004. 430:213–218.


15. Mariathasan S, Weiss DS, Newton K, McBride J, O'Rourke K, Roose-Girma M, Lee WP, Weinrauch Y, Monack DM, Dixit VM. Cryopyrin activates the inflammasome in response to toxins and ATP. Nature. 2006. 440:228–232.


16. Broz P, von Moltke J, Jones JW, Vance RE, Monack DM. Differential requirement for Caspase-1 autoproteolysis in pathogen-induced cell death and cytokine processing. Cell Host Microbe. 2010. 8:471–483.


17. Hornung V, Bauernfeind F, Halle A, Samstad EO, Kono H, Rock KL, Fitzgerald KA, Latz E. Silica crystals and aluminum salts activate the NALP3 inflammasome through phagosomal destabilization. Nat Immunol. 2008. 9:847–856.


18. Yu JW, Fernandes-Alnemri T, Datta P, Wu J, Juliana C, Solorzano L, McCormick M, Zhang Z, Alnemri ES. Pyrin activates the ASC pyroptosome in response to engagement by autoinflammatory PSTPIP1 mutants. Mol Cell. 2007. 28:214–227.


19. Pétrilli V, Papin S, Dostert C, Mayor A, Martinon F, Tschopp J. Activation of the NALP3 inflammasome is triggered by low intracellular potassium concentration. Cell Death Differ. 2007. 14:1583–1589.


20. Eisenbarth SC, Colegio OR, O'Connor W, Sutterwala FS, Flavell RA. Crucial role for the Nalp3 inflammasome in the immunostimulatory properties of aluminium adjuvants. Nature. 2008. 453:1122–1126.


21. Broz P, Newton K, Lamkanfi M, Mariathasan S, Dixit VM, Monack DM. Redundant roles for inflammasome receptors NLRP3 and NLRC4 in host defense against Salmonella. J Exp Med. 2010. 207:1745–1755.

