Abstract
The anti-tumor effect of monocyte-derived DC (MoDC) vaccine was studied in lung cancer model with feasible but weak Ag-specific immune response and incomplete blocking of tumor growth. To overcome this limitation, the hematopoietic stem cell-derived DC (SDC) was cultured and the anti-tumor effect of MoDC & SDC was compared in mouse lung cancer minimal residual model (MRD). Therapeutic DCs were cultured from either CD34+ hematopoietic stem cells with GM-CSF, SCF and IL-4 for 14 days (SDC) or monocytes with GM-CSF and IL-4 for 7 days (MoDC). DCs were injected twice by one week interval into the peritoneum of mice that are inoculated with Lewis Lung Carcinoma cells (LLC) one day before the DC injection. Anti-tumor responses and the immune modulation were observed 3 weeks after the final DC injection. CD11c expression, IL-12 and TGF-β secretion were higher in SDC but CCR7 expression, IFN-γ and IL-10 secretion were higher in MoDC. The proportion of CD11c+CD8a+ cells was similar in both DC cultures. Although both DC reduced the tumor burden, histological anti-tumor effect and the frequencies of IFN-γ secreting CD8+ T cells were higher in SDC treated group than in MoDC. Conclusively, although both MoDC and SDC can induce the anti-tumor immunity, SDC may be better module as anti-tumor vaccine than MoDC in mouse lung cancer.
The development of anti-tumor immune therapies through the activation of antigen-specific immune function has the advantage that the side effects can be significantly reduced and the treatment effect of personalized therapy is excellent. Among these, dendritic cells (DCs) have been studied as anti-tumor vaccine since the late 1990's (1). DCs are the most potent antigen-presenting cells (APCs) and are equipped with the necessary co-stimulatory, adhesion and histocompatibility molecules for initiation of a primary immune response (2-4). DC-based vaccination trials in human solid cancers have aimed to activate the immune system to recognize and destroy tumor cells. The safety and the feasibility of anti-cancer DC vaccines have been confirmed in several clinical trials (5-9). Nevertheless, reports on DC therapy for lung cancer patients were limited (10-12).
Lung cancer is a leading cause of cancer-related death in both men and women worldwide (13). Although various therapeutic modalities, including surgical resection, radiation therapy, and chemotherapy, have been developed, the disease is rarely curable and the prognosis is dismal, with an overall 5-year survival rate of only 15% (14). Therefore the development of effective therapies is clearly needed for treatment of lung cancer. In our previous study (15), we verified the possibility of anti-tumor effect of cultured DCs as an adjuvant therapy for minimal residual disease state of lung cancer.
Although feasible anti-tumor effect of monocyte-derived DC (MoDC) vaccine was resulted in our previous study (15), antigen specific immune responses were weak with partial blocking of tumor growth. To improve the efficiency of anti-tumor effect using DC vaccine for lung cancer, we tested CD34+ hematopoietic stem cell derived DC (SDC) as an alternative in mouse model in this study. SDC can be applied in many clinical researches because hematopoietic stem cells generate a large number of mature DCs in vitro and able to differentiate into various stages. Ward et al. (16) reported that CD34+ DC generated with GM-CSF, IL-4, Flt-3L and SCF, capable of inducing naive T-cell responses and can be expanded to clinically useful numbers. There are reports that CD34+ DCs from core blood have anti-tumor activity (17-19). Our SDC were generated from CD34+-mouse bone marrow cell and compared to MoDC for their DC characters and in vivo anti-tumor effect.
Specific pathogen-free female C57BL/6 mice, 5~6 weeks old, were purchased from the Dae-Han BioLink (EumSung, Korea). The mice were provided with water and food, adlibitum and quarantined under 12 h light: 12 h dark photoperiod in the animal care facility of the Samsung Biomedical Research Institute, Samsung Medical Center, Seoul, Korea. Animal care was performed following the ILAR guideline. The mice were acclimated for at least one week before any experiments were conducted.
Complete medium (CM) consist of RPMI 1640 with 10% Fetal Bovine Serum, 2 mM glutamine and 100 U/ml penicillin plus 100µg/ml streptomycin (all from GIBCO laboratories, Grand Island, NY, USA). Following antibodies for flow cytometric phenotyping were purchased from BD (SanDiego, CA); fluorescein isothiocyanate (FITC)-or phycoerythrin (PE)-labeled monoclonal Abs for MHC I/II, CD11c, CD8a, CD54 and CCR7. ELISA sets for cytokines including IL-10, IL-12, TGF-β and IFN-γ was purchased from BD (Sunnyvale, CA). For isolation of hematopoietic stem cells, a lineage cell depletion kit was obtained from Miltenyi Biotech Inc (Bergisch Gladbach, Germany). C57BL/6 syngeneic Lewis Lung Carcinoma (LLC) cell line was purchased from American type culture collection (ATCC) (Rockville, MD, USA). The following hybridoma cell lines were also purchased from ATCC; GK1.5 for anti-L3T4, 53.672 for anti-Lyt-2, RA3 for anti-B220, and J11d for anti-B cells/neutrophils.
Mononuclear cells (MNCs) from bone marrow were obtained from the tibia and femur of C57BL/6 mouse sacrificed by cervical dislocation. The viability of red blood cell (RBC) free-MNCs was routinely demonstrated to be over 95% by trypan blue exclusion. To separate the myeloid lineage cells, RBC free-MNCs were treated with antibodies against L3T4, Lyt-2, B220, and B cell/neutrophil with complement. Purified cells (1×106/ml) were incubated with GM-CSF and IL-4 (1×103 units/ml each) at 37℃ for 7 d in a humidified CO2 incubator for MoDC differentiation. To generate SDCs, CD34+ cells were isolated from the bone marrow cells by MACS lineage cell depletion kit and cultured with GM-CSF, SCF and IL-4 for 14 days. Eighteen hours before cell harvest, tumor cell lysate (50µg protein/ml) was added to culture media. Tumor cell lysate was prepared from cultured LLC cells by a freezing-thawing process that was repeated six times in liquid nitrogen (-180℃) and an incubator (37℃). Protein quantification of the tumor cell lysate was performed using centrifuged (1,500 rpm, 15 min) supernatant and the Bradford method (Bio-Rad Protein Assay; Bio-Rad Laboratories, Hercules, CA, USA). Cultured cells were characterized as therapeutic DCs by surface marker phenotyping and cytokine secretion into the culture supernatant.
Syngeneic LLC cells were inoculated intravenously into C57BL/6 mice on day 0. Starting from the day after the tumor cell injection, antigen pulsed therapeutic-DCs were injected twice by one week interval into the peritoneum of mice. This way the minimal residual disease status was simulated by having the tumor cells in the blood without established burdens. Each experimental group was composed of 5~7 mice and the control group was treated with saline. Animals were sacrificed at three weeks after the final DC injection to observe tumor growth in the lung and to analyze the systemic immune modulation. The treatment schedule is summarized in Fig. 1. Pulmonary tumor burden and IFN-γ secreting CD8+ T cell proportion (ELISPOT) were detected from the splenocytes of mice in each group.
Phenotype of cultured cells was analyzed by direct immunefluorescence staining of cell surface antigens using FITC or PE conjugated antibodies against MHC I/II, CD11c, CD8a, CD54 and CCR7. Single cells of cultured DC were incubated with fluorescence-labeled surface antibodies in PBS with 0.1% sodium azide and 1% FBS (PBS-CS) for 40 min at 4℃. Within 1 h of the antibody labeling, cells in the 500µl PBS-CS were analyzed by flow cytometry (FACS Vantage; BD, CA, USA).
The concentrations of IL-10, IL-12, TGF-β and IFN-γ in culture supernatant of DC were measured using commercial ELISA kits (BD, Sunnyvale, CA).
Three weeks after the last DC injection, tumor bearing mice were sacrificed. In order to investigate in vivo anti-tumor effect of DC vaccine, lung with heart of the mice were eliminated. To quantify the effect on the tumor establishment and growth, tumor lesions bigger than 2 mm were counted under a microscope (×100). Then these lungs were paraffin embedded and stained with H&E to observe the histo-pathological alterations. Histological observation was performed under the microscope (Leica DM 3000). The picture was presented with ×100 magnification.
The ELISPOT assay was adopted to detect and enumerate individual cells that secrete IFN-γ protein in vitro upon exposure to antigen. ELISPOT IFN-γ assay kit were purchased from AID (AID, Strassberg, Germany) and assays were performed according to the manufacturer's instruction. In brief, using magnetic bead cell separation system (MACS™ Miltenyi Biotec, Germany), CD3+ T cells were purified from the splenocytes (2×105 cells) and stimulated in vitro with tumor lysate on a 96-well pre-coated plate. The plate was incubated for 20 h at 37℃ with 5% CO2. After washing, each well was added with detection antibody and was incubated for 2 h at room temperature. The plate was incubated with alkaline phosphatase conjugate and developed with BCIP/NBT substrate solution. Visible spots were enumerated using an automated AID ELISPOT reader (AID, Strassberg, Germany) and the default program.
The analyzed values are reported as the mean value±standard error (SE). Each experimental group was composed of 5~7 mice, and the same experimental protocol was repeated at least two times with similar results. Statistical significance was determined by two-tailed Student's t-test. p-values<0.05 were interpreted to represent statistically significant differences.
Generated therapeutic DCs were verified through cytokine secretion and phenotype expression. From the SDC supernatant, immune-stimulatory cytokine IL-12 was measured significantly higher level than MoDC (424.82±87.2 vs. 73.51±6.15 pg/ml for SDC vs. MoDC group, 574.38±191 vs. 66.06±2.38 pg/ml for SDC/lysate vs. MoDC/lysate group respectively) (Fig. 2). On the other hand, in the MoDC supernatant, IFN-γ as an immune-stimulatory cytokine were determined significantly higher level than SDC (399.79±105.59 vs. 2,601.31±680.13 pg/ml for SDC vs. MoDC group, 352.69±21.19 vs. 2,090.26±329.86 pg/ml for SDC/lysate vs. MoDC/lysate group respectively) (Fig. 2). Although secretion of immune-inhibitory cytokine IL-10 or TGF-β was observed from each DC group (IL-10 in MoDC group vs. TGF-β in SDC group), there were amounts of immune-stimulatory cytokines which are considered as counterbalance. Over 90% MoDC & SDC were expressed MHC I/II molecules (Fig. 3A) (The data of MHC I does not shown). However, a DC marker CD11c expression was prominent in SDC (Table I). The proportion of CD11c+CD8a+ cell, known as Th1-inducible DC subset was similar in two DC types (Table I) (Fig. 3B). Although the CD54 expression was similar in both DCs, CCR7 expression was prominent in MoDC (Table I) suggesting the better migratory function of MoDC (Fig. 3C). The characters of both SDC and MoDC revealed that both DCs could induce anti-tumor immunity with different mechanisms.
To quantify the effect on the tumor establishment and growth, we eliminated lung with heart of the mice and observed tumor lesions under a microscope. After the DC treatment in LLC injected mice, countable tumor burdens (over than 2 mm under a ×100 microscope) were decreased compared to saline treated group (Fig. 4A). However, no statistical significance was found between the MoDC and SDC as well as with/without tumor lysate group. Despite the fact that statistically significant differences through eye observation were not found, histological alterations were observed from the H&E stained lung sections (Fig. 4B). In DC treated group, a significant amount of tumor was reduced. Moreover, SDC treated group pulsed with LLC lysate shows definite tumor regression. This H&E staining shows SDC/lysate induce more powerful anti-tumor immunity than MoDC/lysate in mouse lung cancer.
To confirm SDC/lysate induce more systemic anti-tumor immunity than MoDC/lysate, we checked effector T cells in splenic lymphocytes from LLC bearing mice. To measure the anti-tumor effector T cells, ELISPOT assay counting IFN-γ secreting CD8+ T cells was operated (Fig. 5). The frequency of IFN-γ secreting CD8+ T cells in SDC treated group was significantly higher than that in MoDC treated group (58.7±8.3 vs. 11.7±1.8 spots for SDC/lysate vs. MoDC/lysate treated group, respectively). It shows SDC/lysate induce naïve T cells to effector T cells which lead to regress residual tumor in mouse lung. The therapeutic responses were associated with induction of IFN-γ secreting CD8+ T cells.
In spite of the aggressive treatment with surgery, radiation, and chemotherapy, the long-term survival for patients with lung cancer still remains low. Even patients with early stage disease often succumb to lung cancer due to the development of metastasis, indicating the need for effective approaches for the systemic therapy of this condition (20). Based on the antigen specificity of the immune system and the safety profile of cancer vaccines, the effective immunotherapy would be an ideal adjuvant, following initial clinical responses to definitive therapy (21). Even though there have been numerous clinical trials for various types of cancer, there are few DC vaccine trials in patients with lung cancer, and many aspects related to the immunotherapy are unknown. Our previous study suggests the possible anti-tumor effect of cultured DCs as an adjuvant therapy for minimal residual disease state of lung cancer (15). Nevertheless, MoDC vaccine in previous study shows weak antigen-specific immune response and incomplete tumor regression. In order to increase the efficiency of anti-tumor response using DC therapy, we introduced other culture methods as alternatives. Hematopoietic stem cell (HSC) as an origin of DC culture has advantage that the culture yield can be increased by more than 30 to 60 times depending on the culture protocol and/or incubation period. Also the HSC has the potential to differentiate into the various type of cell. Authors reported the HSC-derived DC vaccine effect on the renal cell carcinoma and breast cancer patients (7). No report for the effects of SDC on lung cancer treatment was found. The characters of SDC cultured in this protocol allow expectations that SDC may work to induce the anti-tumor immunity, since these cells produce significantly more IL-12 and lower IL-10 than MoDC (Fig. 2). Unlike SDC, cultured MoDC produced significantly higher IFN-γ and lower TGF-β than SDC (Fig. 2) which also may work as anti-tumor effector by different mechanism. Indeed, the proportion of cells expressing CD11c and CD8a, an immunogenic DC subset inducing Th1 response in mouse (22,23), was similar in both DC culture (Fig. 3) with similar response to inhibiting tumor growth (Fig. 4A). One day after inoculation of LLC cells, therapeutic-DCs were injected twice by one week interval into the peritoneum of tumor cell injected mice. Thus the effect of DC could be analyzed as an adjuvant treated after removing the tumor burden. Although DC vaccines inhibit the tumor growth in the lung, there were no statistical significances between the effect of MoDC and SDC when we observed tumor burden over 2 mm size under the microscope (Fig. 4A). This phenomenon was considered because we missed the presence of tumor under 2 mm size. For this reason, we observed lung tissue through H&E staining to determine histo-pathological changes (Fig. 4B). The entire DC treated group revealed the remarkable tumor regression. Affirmatively, no small tumorigenic changes other than countable tumors were observed in the tumor lysate- pulsed SDC treated lung. Also SDC significantly induced antigen-specific IFN-γ secreting CD8+ T cells than MoDC did (Fig. 5). Collectively, although both MoDC and SDC can induce the anti-tumor response, SDC considered as efficient inducer of anti-tumor immunity than MoDC in the mouse lung cancer.
Figures and Tables
Figure 1
Treatment schedule. D-0: LLC cells were inoculated 1×105/mouse i.v. D-1 and 8: In order to minimal residual model, therapeutic-DCs were injected twice by one week interval into the peritoneum of tumor cell inoculated-mice. D-29: Mouse sacrifice and immune monitoring. Each experimental group was composed of 5~7 mice.
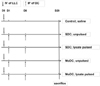
Figure 2
Characterization of cultured DCs. DCs cultured from either CD34+ cells with GM-CSF, SCF and IL-4 for 14 days (SDC) or antibody-panned monocytes with GM-CSF and IL-4 for 7 days (MoDC). Each DC type was pulsed with LLC lysate (SDC/lysate and MoDC/lysate). Cytokines IL-12, IL-10, IFN-γ and TGF-β produced into the culture supernatant were measured by ELISA and expressed as pg/ml/1×106 cells. Statistical significance (p<0.05) was compared by asterisks between the SDC and MoDC. Statistical differences (p<0.05) between the lysate pulsed DCs were expressed by + signs.
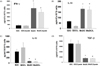
Figure 3
Immunogenic phenotypes of cultured DCs were analyzed by flow cytometry. Dendritic cell marker CD11c as well as MHC II, CD8a and CCR7 with CD54 were measured to characterize and differentiate the cultured SDC and MoDC.
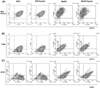
Figure 4
Anti-tumor effect of DCs for LLC formed in lung. Tumor bearing mice were sacrificed after three weeks from the last DC injection. (A) Circle represents number of tumor size over 0.2 mm in a mouse lung. Number and red line show mean value±standard error (SE). Same value represents one circle. Each experimental group was composed of 5~7 mice. (B) Pulmonary tissues of tumor-bearing mice were H&E stained.
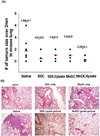
Figure 5
Induction of tumor antigen-specific IFN-secreting cells. Tumor antigen-specific alterations in immunological parameters were analyzed with splenic lymphocytes obtained from the tumor-bearing mice treated with DC vaccine. As an effector molecule of therapeutic response, the proportion of IFN-secreting CD8+ T cells was observed by ELISPOT assay. Asterisk indicate the statistical significance (p<0.05) compared with MoDC.
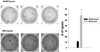
ACKNOWLEDGEMENTS
This study was mainly supported by the grant from the Korean Ministry of Knowledge Economy (#10021360) and in part by Samsung Biomedical Research Institute grants (#SBRI C-A8-501-2).
References
1. Steinman RM. The dendritic cell system and its role in immunogenicity. Annu Rev Immunol. 1991. 9:271–296.


2. Banchereau J, Steinman RM. Dendritic cells and the control of immunity. Nature. 1998. 392:245–252.


3. Paglia P, Chiodoni C, Rodolfo M, Colombo MP. Murine dendritic cells loaded in vitro with soluble protein prime cytotoxic T lymphocytes against tumor antigen in vivo. J Exp Med. 1996. 183:317–322.


4. Palucka K, Banchereau J. Dendritic cells: a link between innate and adaptive immunity. J Clin Immunol. 1999. 19:12–25.
5. Paczesny S, Banchereau J, Wittkowski KM, Saracino G, Fay J, Palucka AK. Expansion of melanoma-specific cytolytic CD8+ T cell precursors in patients with metastatic melanoma vaccinated with CD34+ progenitor-derived dendritic cells. J Exp Med. 2004. 199:1503–1511.


6. Kim JH, Lee Y, Bae YS, Kim WS, Kim K, Im HY, Kang WK, Park K, Choi HY, Lee HM, Baek SY, Lee H, Doh H, Kim BM, Kim CY, Jeon C, Jung CW. Phase I/II study of immunotherapy using autologous tumor lysate-pulsed dendritic cells in patients with metastatic renal cell carcinoma. Clin Immunol. 2007. 125:257–267.


7. Baek S, Kim CS, Kim SB, Kim YM, Kwon SW, Kim Y, Kim H, Lee H. Combination therapy of renal cell carcinoma or breast cancer patients with dendritic cell vaccine and IL-2: results from a phase I/II trial. J Transl Med. 2011. 9:178.


8. Ragde H, Cavanagh WA, Tjoa BA. Dendritic cell based vaccines: progress in immunotherapy studies for prostate cancer. J Urol. 2004. 172:2532–2538.


9. Iwashita Y, Tahara K, Goto S, Sasaki A, Kai S, Seike M, Chen CL, Kawano K, Kitano S. A phase I study of autologous dendritic cell-based immunotherapy for patients with unresectable primary liver cancer. Cancer Immunol Immunother. 2003. 52:155–161.


10. Hirschowitz EA, Foody T, Kryscio R, Dickson L, Sturgill J, Yannelli J. Autologous dendritic cell vaccines for non-small-cell lung cancer. J Clin Oncol. 2004. 22:2808–2815.


11. Hege KM, Carbone DP. Lung cancer vaccines and gene therapy. Lung Cancer. 2003. 41 Suppl 1:S103–S113.


12. Fong L, Hou Y, Rivas A, Benike C, Yuen A, Fisher GA, Davis MM, Engleman EG. Altered peptide ligand vaccination with Flt3 ligand expanded dendritic cells for tumor immunotherapy. Proc Natl Acad Sci U S A. 2001. 98:8809–8814.


13. Parkin DM, Bray F, Ferlay J, Pisani P. Global cancer statistics, 2002. CA Cancer J Clin. 2005. 55:74–108.


14. Spira A, Ettinger DS. Multidisciplinary management of lung cancer. N Engl J Med. 2004. 350:379–392.


15. Lee SJ, Kim MJ, In SH, Baek S, Lee H. Immunocell Therapy for Lung Cancer: Dendritic Cell Based Adjuvant Therapy in Mouse Lung Cancer Model. Immune Netw. 2005. 5:36–44.


16. Ward KA, Stewart LA, Schwarer AP. CD34+-derived CD11c+ + + BDCA-1+ + CD123+ + DC: expansion of a phenotypically undescribed myeloid DC1 population for use in adoptive immunotherapy. Cytotherapy. 2006. 8:130–140.


17. Encabo A, Solves P, Mateu E, Sepúlveda P, Carbonell-Uberos F, Miñana MD. Selective generation of different dendritic cell precursors from CD34+ cells by interleukin-6 and interleukin-3. Stem Cells. 2004. 22:725–740.


18. Guo G, Chen S, Zhang J, Luo L, Yu J, Dong H, Xu H, Su Z, Wu L. Antitumor activity of a fusion of esophageal carcinoma cells with dendritic cells derived from cord blood. Vaccine. 2005. 23:5225–5230.


19. Xu RL, Tang Y, Ogburn PL, Malinowski K, Madajewicz S, Santiago-Schwarz F, Fan Q. Implication of delayed TNF-alpha exposure on dendritic cell maturation and expansion from cryopreserved cord blood CD34+ hematopoietic progenitors. J Immunol Methods. 2004. 293:169–182.


20. Baleeiro RB, Anselmo LB, Soares FA, Pinto CA, Ramos O, Gross JL, Haddad F, Younes RN, Tomiyoshi MY, Bergami-Santos PC, Barbuto JA. High frequency of immature dendritic cells and altered in situ production of interleukin-4 and tumor necrosis factor-alpha in lung cancer. Cancer Immunol Immunother. 2008. 57:1335–1345.


21. Hirschowitz EA, Foody T, Hidalgo GE, Yannelli JR. Immunization of NSCLC patients with antigen-pulsed immature autologous dendritic cells. Lung Cancer. 2007. 57:365–372.


22. Moser M, Murphy KM. Dendritic cell regulation of TH1-TH2 development. Nat Immunol. 2000. 1:199–205.


23. Martín P, del Hoyo GM, Anjuère F, Ruiz SR, Arias CF, Marín AR, Ardavín C. Concept of lymphoid versus myeloid dendritic cell lineages revisited: both CD8alpha(-) and CD8alpha(+) dendritic cells are generated from CD4(low) lymphoid-committed precursors. Blood. 2000. 96:2511–2519.