Abstract
α-Mangostin is a xanthon derivative contained in the fruit hull of mangosteen (Garcinia mangostana L.), and the administration of α-Mangostin inhibited the growth of transplanted colon cancer, Her/CT26 cells which expressed Her-2/neu as tumor antigen. Although α-Mangostin was reported to have inhibitory activity against sarco/endoplasmic reticulum Ca2+ ATPase like thapsigargin, it showed different activity for autophagy regulation. In the current study, we found that α-Mangostin induced autophagy activation in mouse intestinal epithelial cells, as GFP-LC3 transgenic mice were orally administered with 20 mg/kg of α-Mangostin daily for three days. However, the activation of autophagy by α-Mangostin did not significantly increase OVA-specific T cell proliferation. As we assessed ER stress by using XBP-1 reporter system and phosphorylation of eIF2α, thapsigargin-induced ER stress was significantly reduced by α-Mangostin. However, coadministration of thapsigargin with α-Mangostin completely blocked the antitumor activity of α-Mangostin, suggesting ER stress with autophagy blockade accelerated tumor growth in mouse colon cancer model. Thus the antitumor activity of α-Mangostin can be ascribable to the autophagy activation rather than ER stress induction.
α-Mangostin is a xanthone derivative included in the fruit hull of mangosteen (Garcinia mangostana L.), and recently it was reported that α-Mangostin induced apoptosis of several cancer cells via sarcoplasmic/endoplasmic Ca2+ ATPase (SAECA) inhibition and autophagy activation (1,2). Although α-Mangostin has an inhibitory effect on SAECA like thapsigargin, a sesquiterpene lactone, initially isolated from a plant, Thapsia garganica, it was different from thapsigargin in that α-Mangostin activated autophagy pathway, whereas thapsigargin induced autophagy arrest (3). Disruption of endoplasmic reticulum (ER) Ca2+ homeostasis which was mediated by the inhibition of SAECA, resulted in Ca2+ deprivation in ER, raised cytosolic Ca2+ concentration, and consequently apoptosis (4).
Autophagy, especially macroautophagy, is a lysosome-dependent degradation pathway for homeostatic regulation of cells which involved in degradation of cytosolic protein and organelles (5). Several studies have shown that ER stress induced autophagy flow via JNK activation or PERK/eIF2α phosphorylation (6,7). In the current study, the administration of α-Mangostin efficiently inhibited tumor growth in correlation with previous reports showing the anticancer activity of α-Mangostin. Although the role of ER stress in cancer has been studied in depth (8,9), the effect of ER stress-mediated autophagy under ER stress upon anticancer activity was not studied well. Thus we tried to find out the effect of ER stress with autophagy regulators, including α-Mangostin and thapsigargin, on antitumor activity. Interestingly, despite the similar inhibitory effect on SAECA, α-Mangostin did not increase ER stress in vitro, whereas α-Mangostin reduced ER stress induced by thapsigargin treatment. Collectively, these results suggested that autophagy activation by α-Mangostin could overcome ER stress mediated by Ca2+ ATPase blockade by itself. However, autophagy arrest by thapsigargin completely inhibited the antitumor effect by α-Mangostin, suggesting that autophagy activation in cancer might be an important strategy to achieve successful antitumor activity in the presence of ER stress.
BALB/c mice and C57BL/6 mice (Orient-Bio Ltd, Charles River Laboratories, Seoul, Korea) were purchased at 6 weeks of age and used in all of the experiments. GFP-LC3 mice were from Riken with consent on their use from Dr. Mizushima, Noboru in Tokyo Medical and Dental University (10). Mice were fed with standard lab. chow and water ad libitum. The animals were maintained in the KNU animal facility at 20~22℃ under 40~60% relative humidity and a 12 h/12 h (light/dark) cycle for at least 7 days prior to the experiment. All experiments were approved by the Institutional Animal Care and Use Committee of Kangwon National University. In addition, the ethical guidelines described in the KFDA Guide for the Care and Use of Laboratory Animals was followed throughout the experiments.
Mouse colon carcinoma CT26 (American Type Culture Collection, Manassas, VA), and human Her-2/neu expressing CT26 (Her-2/CT26) were used (11). To establish subcutaneous (s.c.) tumors, 2×105 Her-2/CT26 tumor cells were injected on the left flank of BALB/c mice. The tumor growth was measured by caliper 3 times a week.
Bone marrow-derived DC was prepared as described previously (12). Bone marrow cells were incubated with 10 ng/ml of GM-CSF for 7 days and BM-DC were incubated with Ovalbumin (OVA, 100 ng/ml) for 18 hrs. These cells were further incubated with α-Mangostin (1 uM) for four hours before i.v. injection into mice adoptively transferred with carboxylfluorescein diacetate succinimidyl ester (CFSE)-labeled OT-I cells. To prepare CFSE-labeled OT-I cells, OVA-specific CD8+ T cells (>90% of which were Vα2-positive) were isolated from OT-I mice using magnetic beads. These cells were labeled with 5 uM CFSE and i.v. transferred into their syngenic C57BL/6 mice. Two days after DC vaccination, lymphoid cells from the lymph nodes or spleen of the recipient mice were stained with phycoerythrin (PE)-conjugated anti-V α2 and anti-CD8 antibodies and then analyzed by flow cytometry.
All Abs for flow cytometry were from BD Biosciences (San Jose, CA) including anti-CD8-APC and anti-Vα2-PE Ab. For the western blotting, anti-phospho-eIF2α, anti-β-actin and anti-rabbit/mouse HRP antibodies (Cell Signaling Technology) were used.
GFP-LC3 mice were sacrificed, and the small intestine was dissected and promptly washed with PBS. The tissue was fixed in formalin for 18 hours. Fixed tissue was embedded in OCT and sectioned on a cryotome into 8µm sections. Slides were washed with PBS and directly applied to attach coverglass. Images of the sections were collected using Zeiss confocal system (13).
To assess cellular ER stress, we checked the splicing of XBP1, the target of ER stress sensor kinase IRE1α, which was well-known to be activated by ER stress (14). We used the CT26 cells, and transfected them with XBP1-venus DNA (kindly provided by Masayuki Miura, RIKEN, Japan) by lipofectamine 2000 (Invitrogen) following the manufacturer's instructions (14). To induce the ER stress in the CT26 cells, DMSO as negative control or thapsigargin (200 nM; Sigma) were added after plating cells in 96-well plate (2×104 cells/200µl/well) and serially diluted α-Mangostin or DMSO as negative control were added, respectively. After 24 hrs incubation at 37℃, 5% CO2, cells were lysed in digitonin buffer (50 mM Tris-HCl (pH7.5), 1 mM EDTA, 10 mM EGTA and 10µM digitonin) at room temperature for 30 min. We used the GEMINI EM (Molecular Device) to measure fluorescence of the spliced XBP1 (emission at 520 nm; excitation at 485 nm).
CT26 cells were cultured and treated with thapsigargin (200 nM) in the presence of DMSO or α-Mangostin. Western blot analysis was performed using mAbs to phosphor-eIF2α or β-actin as previously reported (14).
The pericarps of G. mangostana L. were collected in Indonesia. The separation and identification of α-Mangostin was conducted as described previously (15). The purity of α-Mangostin was determined to be >95% based on HPLC-UV analysis.
α-Mangostin is a xanthon derivative contained in the fruit hull of mangosteen (Garcinia mangostana L.), and was reported to induce apoptotic effect with Thapsigargin-like Ca2+-ATPase inhibitory effect (16). Likewise, α-Mangostin induced apoptosis in several human cancer cells (17-19). However, recently it was reported that α-Mangostin induced autophagic cell death, but not apoptosis in some brain cancer cell lines as assessed by DNA ladder fragmentation and caspase activities (1). Based on the previous reports supporting the anti-tumor effect of α-Mangostin, we decided to access whether oral administration of α-Mangostin can influence on the growth of colon cancer cells in mouse transplant model. To do this, groups of mice were subcutaneously injected with 2×105 cells of colon cancer cells, CT26 expressing Her-2/neu (Her2/CT26 cells), and orally administered with 20 mg/kg of α-Mangostin daily starting one day after tumor challenge. As expected, mice treated with α-Mangostin have significantly smaller tumor mass than control mice treated with DMSO (Fig. 1), supporting previous report showing anti-tumor effect of α-Mangostin as tested against Her2/CT26 cells.
Although autophagy induction by α-Mangostin in several cell lines were shown previously (1), there was no report showing the influence of α-Mangostin on autophagy activation in vivo. Thus, to assess the influence of orally administered α-Mangostin on autophagy activation in vivo, GFP-LC3 transgenic mice, which have been widely used to detect autophagosome by LC3-II dot formation (10), was adopted. GFP-LC3 transgenic mice were orally administered with 20 mg/kg of α-Mangostin daily for three days and GFP puncta formation by LC3-II conversion in small intestinal villi and crypt epithelial cells including goblet cells and Paneth cells were monitored by confocal microscopy at 18 hrs after final administration (Fig. 2). α-Mangostin induced autophagic activation as determined by LC3-II conversion mainly in Paneth cells as well as in Goblet cells, suggesting that orally administered α-Mangostin induced autophagy activation in small intestinal epithelial cells in vivo.
Autophagy-mediated activation of dendritic cell (DC) was known to be essential for a successful Ag presentation (20), especially for cross-presentation of Ag (21). Thus we accessed whether α-Mangostin would increase the cross-priming of CD8+ T cells by DC. To do this, bone-marrow derived DCs pulsed with OVA protein (0.1 mg/ml) were prepared, and incubated for four hrs with α-Mangostin (1µM) or DMSO as control prior to i.v. transfer into mice (5×105 cells/mouse) following adoptive transfer with CFSE-labeled OT-I CD8+ T cells. To monitor the effect of α-Mangostin, the dilution of CFSE on OT-I T cells by DC/OVA or DC/OVA/α-Mangostin was compared at 3 days after DC vaccination (Fig. 3). Despite the importance of autophagy on Ag presentation, activation of autophagy by α-Mangostin did not significantly increase OVA-specific T cell proliferation, suggesting that the antitumor activity by α-Mangostin might not be due to the augmentation of tumor Ag-specific CTL responses.
Recently it was suggested that ER stress caused by accumulation of unfolded protein in the ER was associated with a wide range of diseases including cancer, cardiac disease, neurodegenerative diseases, and intestinal inflammation (22). Although α-Mangostin was also reported as SAECA inhibitor like thapsigargin, a well-known ER stress inducer (4,16), we wondered whether the treatment of α-Mangostin on thapsigargin-treated cells would influence on ER stress in vitro. In this regard, autophagy activation was recently reported to ameliorate ER stress (23), and autophagy activation by α-Mangostin was reported to possibly ameliorate ER stress induced by thapsigargin treatment.
We used ER stress reporter system, which expresses fluorescence by ER stress-induced XBP1 splicing (14), to monitor cellular ER stress. Mouse colon cancer CT26 cells were transfected with XBP1-venus reporter, and incubated with 200 ng/ml of thapsigargin for 4 hrs in the presence or absence of the different concentration of α-Mangostin. The treatment of CT26 cells with 200 nM of thapsigargin induced XBP1 splicing monitoring by GFP expression, suggesting ER stress induction (Fig. 4A). Interestingly, ER stress-induced XBP1 splicing was significantly reduced by co-treatment of cells with 100 ng/ml and 250 ng/ml of α-Mangostin. These results suggest that the treatment of cells with α-Mangostin can reduce ER stress caused by thapsigargin.
To confirm the reduction of thapsigargin-mediated ER stress by α-Mangostin, CT26 cells were treated with 200 ng/ml of thapsigargin with or without 250 ng/ml of α-Mangostin and assessed phosphorylation of eIF2α by western blot analysis. PERK-eIF2α is another UPR pathway activated by ER stress (Fig. 4B and C). Treatment of cells with thapsigargin increased eIF2α phosphorylation, but α-Mangostin alone did not. Consistent with reduced XBP1 splicing in thapsigargin treated CT26 cells after α-Mangostin treatment, α-Mangostin reduced eIF2α phosphorylation by thapsigargin, suggesting that α-Mangostin inhibits ER stress induction by thapsigargin.
Previously, it was reported that thapsigargin specifically inhibited autophagy flow by blocking autophagosome fusion with lysosome (3). It is quite interesting because both thapsigargin and α-Mangostin were known as inhibitors for SERCA, and they block the pumping of calcium into the sarcoplasmic and endoplasmic reticulum and result in ER stress induction (4,16). As shown in Fig. 4, α-Mangostin did not induce ER stress in the concentration we used, whereas thapsigargin significantly increased ER stress. To assess the consequence of coadministration of α-Mangostin and thapsigargin on tumor growth, groups of mice were subcutaneously injected with 2×105 cells of Her2/CT26 cells, and α-Mangostin and thapsigargin was administered every day starting one day before tumor injection (Fig. 5). When the growth of tumors was monitored, we found that the growth retardation observed in mice treated with α-Mangostin alone disappeared in mice coadministered with thapsigargin. On the contrary, coadministration of α-Mangostin with thapsigargin rather increased tumor growth as compared with DMSO-treated mice. These result suggested that autophagic arrest by systemic treatment of thapsigargin in vivo inhibited antitumor activity of α-Mangostin.
Autophagy is a cellular process for the removal of abnormal intracellular organelles, pathogens and the recycling of cytoplasmic material to obtain energy (24). This pathway also has been involved in immune responses, including antigen processing and presentation pathway (20,21,24,25). Autophagy mediated intracellular lysosome-dependent degradation of abnormal cellular macromolecules and pathogens, and resulted in the accumulation of autophagolysosomes in the cytosol (24). During the formation of autophagolysosome, LC3 and ATG12, two ubiquitin-like protein systems, play an important role for the autophagosomal membrane formation and expansion (26). The conversion of soluble LC3-I to the membrane bound LC3 II is often used to monitor autophagy activation in the cell and GFP-LC3 constructs have been used successfully to track autophagy activation in vivo and in vitro (26). In the current study, we adopted GFP-LC3 mice to monitor whether oral administration of α-Mangostin would induce autophagy activation, and the increased GFP dots in intestinal epithelium suggesting the activation of autophagy.
The unfolded protein response (UPR) is an evolutionary conserved process to ER stress induced by accumulation of misfolded proteins in ER lumen (27). UPR pathway is known to be important to the development and survival of cells, especially for highly secretory cells or cells that synthesize large quantities of proteins (27). In mammals, the UPR is mediated by three transmembrane proteins that reside in the ER (27): PKR-like ER-resident kinase (PERK), activated transcription factor 6 (ATF6) and inositol requiring enzyme 1 (IRE1) pathways. The accumulation of misfolded proteins within the ER attracts the chaperone protein, Grp78 and activates UPRs to facilitate protein folding, attenuate translation, and activate ER-associate degradation (ERAD) pathway. Briefly, ER stress-mediated dissociation of PERK from Grp78 leads to transphosphorylation of PERK and subsequently increases phosphorylation of eIF2α, which attenuate translation of CAP-dependent protein. At the same time, phosphorylated eIF2α induces transcription of ATF4 which activates a number of UPR genes including Grp78, CHOP and GADD34. ATF6 upon release from a Grp78 translocates to the Golgi and then induces transcription of several UPR genes including X-box protein 1 (XBP1), CHOP and GRP78. The most evolutionary conserved of the UPRs is IRE1. Activated IRE1 can splice the Xbp1 mRNA into the active form of Xbp1 (XBP1s) which regulates important genes involved in ER translocation, expansion and ERAD activation. Activated IRE1 also has kinase activity, and signals through TRAF2 that in turn activates JNK and its downstream targets that could promote inflammatory response and/or apoptosis. In the current study, we used XBP-1 reporter which express GFP fluorescence upon XBP-1 splicing by IRE-1 during ER stress. In addition, we detected phosphorylation of eIF2α to assess alteration of ER stress status in cells treated with thapsigargin and α-Mangostin. Consequently, we found that ER stress induced by thapsigargin can be reduced by cotreatment of α-Mangostin. In addition, although α-Mangostin was shown to inhibit SAECA like thapsigargin, it did not induce ER stress under concentration we used. Since thapsigargin specifically inhibits the fusion of autophagosome with lysosome, the last step in autophagic pathway (3), it might induce the accumulation of ER stress by the defect in autophagic elimination of ER stress.
Interestingly, recent studies suggested that UPR mediated by ER stress was known to be linked with autophagy activation (6,7). The activation of autophagy pathway by ER stress includes the following pathways: IRE1-mediated JNK activation and PERK/eIF2α phosphorylation (6,7). Although both of UPR and autophagy are important for cellular homeostasis, unfolded protein responses triggered by ER stress have been shown in several tumors, particularly in area with hypoxia, and they help tumor cells survive in stressful tumor microenvironment (8). Thus, the outcome of autophagy activation by small molecule drugs inducing autophagy activation on the survival of tumor was difficult to predict. Indeed, there was controversy about the pro-apoptotic or anti-apoptotic roles of autophagy (6,21). Further, in several recent experiments done by others, it was suggested that ER stress can induce autophagy and the induction of autophagy by ER stress is beneficial to relieve ER stress and consequently ameliorate the disease progress (23). However, the relationship between the two processes has not been fully elucidated yet. In the current study, activation of autophagy by α-Mangostin significantly inhibited tumor growth, suggesting it exerted proapoptotic function against tumor cells. Likewise, thapsigargin, a well-known ER stress inducer, which was recently proven to block autophagy flow, completely blocked the anti-tumor activity by α-Mangostin. Collectively, these results suggest that autophagy activation by α-Mangostin might be important for the successful antitumor activity.
Figures and Tables
Figure 1
Administration of α-Mangostin induced antitumor effect. BALB/c mice were s.c. injected with of Her2/CT26 cells (2×105 cells/mouse). One day after tumor challenge, mice were orally administrated with 20 mg/kg of α-Mangostin daily. The growth of solid tumor was measured by caliper three times a week. *p<0.05 and **p<0.01 (Student's t-test; n=5/group). Tumor growth was monitored and mean values for tumor volume±SE are shown.
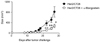
Figure 2
Autophagy activation of α-Mangostin in the murine intestine. (A) Transgenic GFP-LC3 mice were orally administrated with 20 mg/kg of α-Mangostin (b and d) or DMSO (a and c) daily for three days. To analyze the conversion of LC3-I to LC-3-II, GFP puncta formation in small intestinal villi (a and b) and crypt epithelial cells (c and d), were detected by confocal microscopy at 18 hrs after final administration with α-Mangostin. Scale bar: 10µm. (B) Quantification of autophagy dots/cells shown in (A). The value of mean and standard error is indicated. **p<0.01 (Student's t-test).
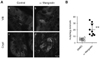
Figure 3
Ag-specific CD8+ T cell proliferation was not increase according to α-Mangostin administration. (A) Bone-marrow derived DCs were mixed with OVA protein (0.1 mg/ml). The DCs plus OVA protein were treated with α-Mangostin (1 uM) or control DMSO for 4 hrs. One day after adoptive transfer with CFSElabeled OT-I CD8+ T cells, mice were intravenously given with 5×105 cells/mouse of DC/OVA-DMSO or DC/OVA-α-Mangostin. Three days after DC vaccination, the CFSE dilution in OT-I cells was detected by flow cytometer. (B) The percentage of divided cells in total CFSE+ OT-I T cells shown in (A). The value of mean and standard error is indicated (n=3/group). n.s., not significant.
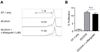
Figure 4
ER stress was significantly reduced by α-Mangostin treatment. (A) CT26 cells transfected with XBP1-venus reporter were treated with serially diluted α-Mangostin in the absence or presence of 200 nM of thapsigargin for 24 hrs. As negative control, DMSO was used. After incubation, the fluorescence of the spliced XBP1 in the treated cells was measured by fluorescence microplate reader (emission at 520 nm; excitation at 485 nm). (B) Western blot analysis of phosphorylated eIF2α in CT26 cells treated with 200 nM of thapsigargin with or without 250 ng/ml of α-Mangostin. (C) Quantification of phosphorylated eIF2α band density shown in (B).
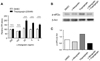
Figure 5
Antitumor effect of α-Mangostin was abrogated by ER stress induction by thapsigargin treatment. BALB/c mice were s.c. injected with 2×105 cells of Her2/CT26 cells. One day before tumor challenge, thapsigargin alone or thapsigargin together with α-Mangostin were administrated via oral route daily starting one day before tumor challenge. The growth of tumor was measured by caliper. *p<0.05 as compared with DMSO-treated group (Student's t-test; n=5/group).
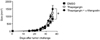
ACKNOWLEDGEMENTS
This work was supported by a 2010 Research Grant from Kangwon National University, by the Ministry for Health, Welfare and Family affairs, Republic of Korea (C1007254), and by a National Research Foundation of Korea (NRF) grant funded by the Korean government (MEST) (No. 2011-0009018).
References
1. Chao AC, Hsu YL, Liu CK, Kuo PL. α-Mangostin, a dietary xanthone, induces autophagic cell death by activating the AMP-activated protein kinase pathway in glioblastoma cells. J Agric Food Chem. 2011. 59:2086–2096.


2. Furukawa K, Shibusawa K, Chairungsrilerd N, Ohta T, Nozoe S, Ohizumi Y. The mode of inhibitory action of alpha-mangostin, a novel inhibitor, on the sarcoplasmic reticulum Ca(2+)-pumping ATPase from rabbit skeletal muscle. Jpn J Pharmacol. 1996. 71:337–340.


3. Ganley IG, Wong PM, Gammoh N, Jiang X. Distinct autophagosomal-lysosomal fusion mechanism revealed by thapsigargin-induced autophagy arrest. Mol Cell. 2011. 42:731–743.


4. Thastrup O, Cullen PJ, Drøbak BK, Hanley MR, Dawson AP. Thapsigargin, a tumor promoter, discharges intracellular Ca2+ stores by specific inhibition of the endoplasmic reticulum Ca2(+)-ATPase. Proc Natl Acad Sci USA. 1990. 87:2466–2470.


6. Ogata M, Hino S, Saito A, Morikawa K, Kondo S, Kanemoto S, Murakami T, Taniguchi M, Tanii I, Yoshinaga K, Shiosaka S, Hammarback JA, Urano F, Imaizumi K. Autophagy is activated for cell survival after endoplasmic reticulum stress. Mol Cell Biol. 2006. 26:9220–9231.


7. Kouroku Y, Fujita E, Tanida I, Ueno T, Isoai A, Kumagai H, Ogawa S, Kaufman RJ, Kominami E, Momoi T. ER stress (PERK/eIF2alpha phosphorylation) mediates the polyglutamine-induced LC3 conversion, an essential step for autophagy formation. Cell Death Differ. 2007. 14:230–239.


8. Bi M, Naczki C, Koritzinsky M, Fels D, Blais J, Hu N, Harding H, Novoa I, Varia M, Raleigh J, Scheuner D, Kaufman RJ, Bell J, Ron D, Wouters BG, Koumenis C. ER stress-regulated translation increases tolerance to extreme hypoxia and promotes tumor growth. EMBO J. 2005. 24:3470–3481.


9. Blais JD, Addison CL, Edge R, Falls T, Zhao H, Wary K, Koumenis C, Harding HP, Ron D, Holcik M, Bell JC. Perk-dependent translational regulation promotes tumor cell adaptation and angiogenesis in response to hypoxic stress. Mol Cell Biol. 2006. 26:9517–9532.


10. Mizushima N, Yamamoto A, Matsui M, Yoshimori T, Ohsumi Y. In vivo analysis of autophagy in response to nutrient starvation using transgenic mice expressing a fluorescent autophagosome marker. Mol Biol Cell. 2004. 15:1101–1111.


11. Kim YJ, Ko HJ, Kim YS, Kim DH, Kang S, Kim JM, Chung Y, Kang CY. alpha-Galactosylcer-amide-loaded, antigen-expressing B cells prime a wide spectrum of antitumor immunity. Int J Cancer. 2008. 122:2774–2783.


12. Chang SY, Cha HR, Chang JH, Ko HJ, Malissen B, Iwata M, Kweon MN. Lack of retinoic acid leads to increased langerin-expressing dendritic cells in gut-associated lymphoid tissues. Gastroenterology. 2010. 138:1468–1478.


13. Ko HJ, Yang H, Yang JY, Seo SU, Chang SY, Seong JK, Kweon MN. Expansion of Tfh-like cells during chronic Salmonella exposure mediates the generation of autoimmune hypergammaglobulinemia in MyD88-deficient mice. Eur J Immunol. 2012. 42:618–628.


14. Iwawaki T, Akai R, Kohno K, Miura M. A transgenic mouse model for monitoring endoplasmic reticulum stress. Nat Med. 2004. 10:98–102.


15. Quan GH, Oh SR, Kim JH, Lee HK, Kinghorn AD, Chin YW. Xanthone constituents of the fruits of Garcinia mangostana with anticomplement activity. Phytother Res. 2010. 24:1575–1577.


16. Sato A, Fujiwara H, Oku H, Ishiguro K, Ohizumi Y. Alpha-mangostin induces Ca2+-ATPase-dependent apoptosis via mitochondrial pathway in PC12 cells. J Pharmacol Sci. 2004. 95:33–40.


17. Watanapokasin R, Jarinthanan F, Nakamura Y, Sawasjirakij N, Jaratrungtawee A, Suksamrarn S. Effects of α-mangostin on apoptosis induction of human colon cancer. World J Gastroenterol. 2011. 17:2086–2095.


18. Nakagawa Y, Iinuma M, Naoe T, Nozawa Y, Akao Y. Characterized mechanism of alpha-mangostin-induced cell death: caspase-independent apoptosis with release of endonuclease-G from mitochondria and increased miR-143 expression in human colorectal cancer DLD-1 cells. Bioorg Med Chem. 2007. 15:5620–5628.


19. Matsumoto K, Akao Y, Yi H, Ohguchi K, Ito T, Tanaka T, Kobayashi E, Iinuma M, Nozawa Y. Preferential target is mitochondria in alpha-mangostin-induced apoptosis in human leukemia HL60 cells. Bioorg Med Chem. 2004. 12:5799–5806.


20. Lee HK, Mattei LM, Steinberg BE, Alberts P, Lee YH, Chervonsky A, Mizushima N, Grinstein S, Iwasaki A. In vivo requirement for Atg5 in antigen presentation by dendritic cells. Immunity. 2010. 32:227–239.


21. Li Y, Hahn T, Garrison K, Cui ZH, Thorburn A, Thorburn J, Hu HM, Akporiaye ET. The vitamin E analogue α-TEA stimulates tumor autophagy and enhances antigen cross-presentation. Cancer Res. 2012. 72:3535–3545.


22. Kim I, Xu W, Reed JC. Cell death and endoplasmic reticulum stress: disease relevance and therapeutic opportunities. Nat Rev Drug Discov. 2008. 7:1013–1030.


23. Hetz C, Thielen P, Matus S, Nassif M, Court F, Kiffin R, Martinez G, Cuervo AM, Brown RH, Glimcher LH. XBP-1 deficiency in the nervous system protects against amyotrophic lateral sclerosis by increasing autophagy. Genes Dev. 2009. 23:2294–2306.


24. Mizushima N, Levine B, Cuervo AM, Klionsky DJ. Autophagy fights disease through cellular self-digestion. Nature. 2008. 451:1069–1075.

