Abstract
Background
Biodegradable polymers have increasingly been recognized for various biological applications in recent years. Here we examined the immunostimulatory activities of the novel poly(aspartic acid) conjugated with L-lysine (PLA).
Methods
PLA was synthesized by conjugating L-lysine to aspartic acid polymer. PLA-nanoliposomes (PLA-NLs) were prepared from PLA using a microfluidizer. The immunostimulatory activities of PLA-NLs were examined in mouse bone marrow-derived dendritic cells (BM-DCs).
Results
PLA-NLs increased the number of BM-DCs when added to cultures of GM-CSF-induced DC generation on day 4 after the initiation of cultures. Examination of the phenotypic properties showed that BM-DCs generated in the presence of PLA-NLs are more mature in terms of the expression of MHC class II molecules and major co-stimulatory molecules than BM-DCs generated in the absence of PLA-NLs. In addition, the BM-DCs exhibited enhanced capability to produce cytokines, such as IL-6, IL-12, TNF-α and IL-1β. Allogeneic mixed lymphocyte reactions also confirmed that the BMDCs were more stimulatory on allogeneic T cells. PLA- NL also induced further growth of immature BM-DCs that were harvested on day 8.
The importance of polymers with biodegradability and biocompatibility has increasingly been recognized for various biological applications. Biodegradable polymers based on both natural and synthetic polymers are widely used as viscosity agents, emulsifying agents, and carriers for delivery of drugs or active ingredients in medical, cosmetic, and other industrial fields (1,2).
Poly-glutamic acid (PGA), for instance, is an anionic polymer that is composed of D- and L-glutamic acid units (3,4). PGA is produced by Bacillus subtilis during fermentation of soybeans. Recent reports have demonstrated immune modulatory activities of PGA or its derivatives (5-7). Nanoparticles composed of PGA and L-phenylalanine ethylester served as an adjuvant that was able to activate dendritic cells and enhance adaptive immune responses against antigens carried by the nanoparticles (8,9).
Poly(aspartic acid), which is one of the poly(amino acid)s and biodegradable synthetic polymers, is a water-soluble polyamide containing carboxylic pendants that can be obtained from the hydrolysis of polysuccinimide (10,11), the thermal polycondensation product of L-aspartic acid (12,13). Due to their amphiphilic uniqueness, amino acid polymers containing both hydrophilic and hydrophobic components can be used to stabilize dispersions, emulsions, and polymer blends (14,15).
L-lysine is one of the most important amino acids and has been known to have various biological functions including regulation of cytokine synthesis and lymphocyte proliferation (16-18). L-lysine is also important to the collagen structure in connective tissue in skin, ligaments, cartilage, vertebral discs, joint linings, capillary walls, bones and teeth (16). L-lysine components conjugated to the polymer backbone have specific biological functions in vivo and a unique pH-sensitive property (19,20). Poly (L-lysine) has been widely used as a gene delivery carrier due to its strong ability to protect DNA from nuclease attack (21).
Liposomes, spherical nanoparticles with an aqueous compartment, possess the unique ability to effectively deliver both water-soluble compounds (in the aqueous phase) and lipid-soluble compounds (in the lipid bilayer) (22,23). Moreover, liposomes rapidly penetrate the dermal barrier, making the delivery of water-soluble compounds as effective as that of lipid-soluble compounds (24). In contrast with other drug carriers such as DMSO (25), liposomes are entirely non-toxic to human skin since they are composed of naturally occurring biodegradable compartments (26).
In this work, novel liposomes composed of biodegradable polymers based on poly(aspartic acid) conjugated with L-lysine (PLA-NLs) were prepared from PLA using a microfluidizer. The immunomodulatory activities of PLA-NLs on bone marrow derived-dendritic cells (BM-DCs) were then investigated. The present study showed that PLA-NLs induce the generation and functional activities of BM-DCs.
Polymers of L-aspartic acid and L-lysine-conjugated poly (aspartic acid), PLA, were prepared as described previously (2,27). The powdery product was filtered out and dried under vacuum at 40℃ for three days to obtain PLA as a yellowish white powder (yield 81%).
PLA-NLs were prepared from PLA as described in Table I. The phases described in Table I, A, B and B-1, were dissolved at 70℃, respectively. The dissolved A phase was added to the mixed phase of B and B-1 and then this mixed solution was passed through a microfluidizer (M110F, Microfluidic, USA) three times at 1000 bar to prepare PLA-NLs.
The average size of PLA-NL-1 and PLA-NL-2 was 70 nm and 200 nm, respectively, when measured using a particle size analyzer (ELS-Z, Photal, Japan).
PLA-NL-1 was a nanoliposome made of lecithin and PLA-NL-2 was a nanoliposome made of phosphatidyl serine.
Cells were cultured in Dulbecco's modified Eagle's medium (Hyclone Laboratories, Inc., UT, USA) supplemented with 10% heat-inactivated fetal bovine serum (Hyclone), 100 U/ml penicillin and 100 µg/ml streptomycin (Hyclone), and 50 µM 2-mercaptoethanol (SIGMA-ALDRICH, Inc., St. Louis, MO, USA) at 37℃ in a 5% CO2 atmosphere.
BM-DCs were generated from total bone marrow cells as described previously (28). Briefly, bone marrow cells obtained from femurs of C57BL/6 mice were cultured in 6-well plates (5×106/well) in culture medium supplemented with 40 ng/ml GM-CSF (CreaGene, Seongnam, Korea). At days 3 and 4 after the initiation of the culture, nonadherent cells were discarded by gentle shaking and replacing the culture medium with fresh medium containing GM-CSF. BM-DCs were harvested by gentle pipetting on day 8. In experiments examining the effects of PLA-NLs, PLA-NLs were added to the culture on day 4 after the initiation of the culture, and the resultant BM-DCs were harvested on day 8 and used for phenotypic analysis and functional assays.
BM-DCs harvested on day 8 were cultured in the presence of different concentrations of PLA-NLs in 96-well microtiter plates (2×104 cells/well). DNA synthesis was measured by [3H]-thymidine (PerkinElmer) incorporation (0.5 µCi/well) for the final 16 h of the 2-day culture period. The cells were harvested onto glass fiber filter paper using a cell harvester. The filters were washed, dried, and then counted in a microbeta liquid scintillation counter (Wallac, USA).
BM-DCs were treated with mitomycin C (SIGMA-ALDRICH) for 20 min at 37℃, washed three times with culture medium, and then adjusted to 5×104 cells/ml. Responder T cells were isolated from spleens of BALB/c mice by a nylon wool-enrichment technique and adjusted to 5×105 cells/ml. Each 100 µl of cell suspension was mixed and cultured in 96-well microtiter plates. DNA synthesis was measured by [3H]-thymidine (PerkinElmer) incorporation (0.5 µCi/well) for the final 16 h of the 3-day culture period. The cells were harvested onto glass fiber filter paper using a cell harvester. The filters were washed, dried, and then counted in a microbeta liquid scintillation counter (Wallac, USA).
BM-DCs were cultured in the presence of different concentrations of PLA-NLs with OVA-containing nanoparticles (50 µg/ml as OVA) in 12-well microtiter plates (1×106 cells/well/2 ml). Nanoparticles containing OVA were prepared using a homogenization/solvent evaporation method using poly(lactic-co-glycolic acid), as described previously (29). After 24-h incubation, the culture supernatants were collected, and the amounts of IL-12, IL-6, IL-1β and TNF-α were measured using commercial immunoassay kits (BD Biosciences, San Diego, CA, USA) according to the manufacturer's instructions.
Cells were stained with monoclonal antibodies recognizing murine cell surface markers as described previously (30), and flow cytometric analysis was performed on a FACSCanto II (BD Biosciences). The monoclonal antibodies, anti-ICAM-1 (clone 3E2), anti-I-Ab (clone AF6-120.1), anti-B7-1 (clone 16-10A1), anti-B7-2 (clone GL1), and isotype-matched control antibodies were purchased from BD Biosciences. Dead cells were gated out by their low forward angle light scatter intensity. In most analyses, 10,000 cells were scored.
To examine the effects of PLA-NLs on the growth and maturation of BM-DCs, PLA-NLs were added to the cultures of BM cells on day 4 after the initiation of the culture, and the resultant BM-DCs were harvested on day 8. As shown in Fig. 1A, addition of PLA-NL-1 (0.1 mg/ml) to cultures of BM cells increased the number of BM-DCs that were harvested on day 8 by approximately 76% compared to the GM-CSF only control. PLA-NL-2 (0.1 mg/ml) also increased the number of BM-DCs by approximately 74% compared to the GM-CSF only control (Fig. 1). Addition of 1 mg/ml of PLA-NLs to BM cells appeared to be toxic to the cells.
Because increased expression of cell surface molecules could be a marker of dendritic cell activation, we examined the effect of PLA-NLs on the expression levels of MHC class II and co-stimulatory molecules in BM-DCs that were harvested on day 8. As shown in Fig. 2, BM-DCs that were generated in medium containing GM-CSF without PLA-NLs stimulation exhibited characteristics of immature DCs based on staining with I-Ab, B7-1, B7-2 and ICAM-1 molecules. In contrast, the BM-DCs generated in the presence of PLA-NLs exhibited more mature phenotypes. The expression levels of I-Ab, B7-1, B7-2 and ICAM-1 molecules were significantly increased in BM-DCs generated in the presence of PLA-NLs. The effects of PLA-NL-1 and PLA-NL-2 on the phenotypic changes appeared to be similar.
Because PLA-NLs appeared to induce differentiation of BM-DCs, the cytokine producing capability of BM-DCs that were generated in the presence of PLA-NLs was compared with that of control BM-DCs. BM-DCs that were stimulated with OVA-containing poly(lactic-co-glycolic acid) nanoparticles (50 µg/ml as OVA) for 24 h, culture supernatants were collected from the stimulated BM-DCs, and the amounts of IL-6, IL12, IL-1β and TNF-α were measured by ELISAs. As shown in Fig. 3, the BM-DCs that were generated in the presence of PLA-NLs produced significantly enhanced levels of all the cytokines that were examined.
It has previously been shown that DCs cultured in the presence of OVA-containing poly(lactic-co-glycolic acid) nanoparticles produce large amounts of cytokines (31). The present study shows that BM-DCs generated in the presence of PLA-NLs produced even further increased levels of cytokines when stimulated with OVA-containing poly(lactic-co-glycolic acid) nanoparticles.
The allostimulatory capacity of BM-DCs generated in the presence of PLA-NLs was examined in allogeneic mixed lymphocyte reactions. As shown in Fig. 4, control BM-DCs that were generated in medium containing GM-CSF only induced low to moderate proliferation of allogeneic T cells. In contrast, BM-DCs that were generated in the presence PLA-NLs exhibited significantly enhanced allostimulatory capacity. The proliferation of allogeneic T cells was increased by approximately 220% when BM-DCs generated in the presence of PLA-NLs were used as stimulatory cells compared to BM-DCs generated in GM-CSF only. Since all the BM-DCs were treated with mitomycin C and subsequently washed thoroughly to remove mitomycin C, the T cell proliferation must be a reflection of increased BM-DC function.
To examine the effects of PLA-NLs on the further growth of immature BM-DCs, immature BM-DCs were harvested on day 8 and then cultured for 2 days in the presence or absence of PLA-NLs. During this 2-day culture period, GM-CSF was not added to the culture medium. As shown in Fig. 5, BM-DCs that were harvested on day 8 did not proliferate actively when cultured in GM-CSF-free medium. In contrast, BM-DCs that were harvested on day 8 proliferated significantly when cultured in the presence of PLA-NLs (1 mg/ml).
The present study shows that PLA-NLs exert significant immunostimulating activity on DCs. It has been well-established that DCs can be generated from bone marrow stem cells by culturing in the presence of a high dose of GM-CSF (32). To examine the effects of PLA-NLs on the generation of BM-DCs from BM cells, PLA-NLs were added to BM cells cultures together with GM-CSF on day 4 after the initiation of cultures. BM-DCs were harvested on day 8, and the numbers, phenotype and functional characteristics of the cells were examined.
The present study shows that PLA-NLs when added to BM cell cultures together with GM-CSF induce phenotypic and functional maturation of the BM-DCs. In addition, PLA-NLs induced the proliferation of immature DCs. The proliferation-inducing activity of PLA-NLs was observed not only when PLA-NLs were added to BM-cell cultures on day 4 after initiation of culture, but also when PLA-NLs were added to BM-DCs that were harvested on day 8. When added to cultures of immature DCs, PLA-NLs induced phenotypic maturation as evidenced by up-regulation of class II MHC molecules and co-stimulatory molecules such as I-Ab, B7-1, B7-2 and ICAM-1.
The DCs cultured with PLA-NLs (0.1 mg/ml) exhibited more potent allogeneic T cell stimulatory activity and induced the proliferation of GM-CSF-generated BM-DCs. DCs can recognize and process antigens in the periphery and then migrate to secondary lymphoid organ where they prime primary T cell responses (33). The capability of DCs to activate naive T cells in a primary response has been explained by their ability to express high levels of class II MHC and co-stimulatory molecules (34). The immature DCs must be matured or further activated in order to fully perform accessory cell functions (35). Maturation signals appear to be very diverse and include inflammatory cytokines such as TNF-α and IL-1β (36). It is noteworthy that 1 mg/ml PLA-NLs appeared to be toxic to the cells when they were added to very early stage of DC generation (day 4 from the initiation of the culture) (Fig. 1). However, PLA-NLs did not exert cytotoxic activity on immature BM-DCs that were harvested on day 8 from the initiation of the culture (Fig. 5). At 1 mg/ml concentration, PLA-NLs even increased the proliferation of day-8 immature DCs. The underlying mechanism for the differential effects is not clear at present.
These results show that PLA-NLs induce the generation and functional activities of BM-DCs. Both types of PLA-NLs, PLA-NL-1 and PLA-NL-2, exerted similar stimulatory activity on BM-DCs.
ACKNOWLEDGEMENTS
This research was financially supported by the Ministry of Knowledge Economy (MKE) and Korea Institute for Advancement of Technology (KIAT) through the Research and Development for Regional Industry.
References
1. Min SK, Kim SH, Kim JH. Preparation and swelling behavior of biodegradable poly(aspartic acid)-based hydrogel. J Ind Eng Chem. 2000; 6:276–279.
2. Goddard JM, Hotchkiss JH. Polymer surface modification for the attachment of bioactive compounds. Progress in Polymer Science. 2007; 32:698–725.


3. Champion JA, Walker A, Mitragotri S. Role of particle size in phagocytosis of polymeric microspheres. Pharm Res. 2008; 25:1815–1821. PMID: 18373181.


4. Elamanchili P, Diwan M, Cao M, Samuel J. Characterization of poly(D,L-lactic-co-glycolic acid) based nanoparticulate system for enhanced delivery of antigens to dendritic cells. Vaccine. 2004; 22:2406–2412. PMID: 15193402.


5. Akagi T, Shima F, Akashi M. Intracellular degradation and distribution of protein-encapsulated amphiphilic poly(amino acid) nanoparticles. Biomaterials. 2011; 32:4959–4967. PMID: 21482432.


6. Foged C, Sundblad A, Hovgaard L. Targeting vaccines to dendritic cells. Pharm Res. 2002; 19:229–238. PMID: 11934227.
7. Panyam J, Labhasetwar V. Biodegradable nanoparticles for drug and gene delivery to cells and tissue. Adv Drug Deliv Rev. 2003; 55:329–347. PMID: 12628320.


8. Matsusaki M, Hiwatari K, Higashi M, Kaneko T, Akashi M. Stably-dispersed and surface-functional bionanoparticles prepared by self-assembling amphipaethic polymers of hydrophilic poly(g-glutamic acid) bearing hydrophobic amino acids. Chemistry Letters. 2004; 33:398–399.
9. Akagi T, Wang X, Uto T, Baba M, Akashi M. Protein direct delivery to dendritic cells using nanoparticles based on amphiphilic poly(amino acid) derivatives. Biomaterials. 2007; 28:3427–3436. PMID: 17482261.


10. Giammona G, Pitarresi G, Tomarchio V, Dispenza C, Spadaro G. Synthesis and characterization of water-swellable α,β-polyasparthydrazide derivatives. II. Hydrogels at low crosslinking degree as potential systems for anticancer drug release. Colloid and Polymer Science. 1995; 273:559–564.
11. Nakato T, Yoshitake M, Matsubara K, Tomida M, Kakuchi T. Relationships between structure and properties of poly(aspartic acid)s. Macromolecules. 1998; 31:2107–2113.


12. Nakato T, Kusuno A, Kakuchi T. Synthesis of poly(succinimide) by bulk polycondensation of L-aspartic acid with an acid catalyst. Journal of Polymer Science Part A: Polymer Chemistry. 2000; 38:117–122.
13. Matsubara K, Nakato T, Tomida M. 1H and 13C NMR characterization of poly(succinimide) prepared by thermal polycondensation of l-aspartic acid. Macromolecules. 1997; 30:2305–2312.
14. Horgan A, Saunders B, Vincent B, Heenan RK. Poly(butyl methacrylate-g-methoxypoly(ethylene glycol)) and poly (methyl methacrylate-g-methoxypoly(ethylene glycol)) graft copolymers: preparation and aqueous solution properties. J Colloid Interface Sci. 2003; 262:548–559. PMID: 16256637.
15. Zhu G. Micellization of polystyrene-graft-poly(ethylene oxide) and its mixtures with polystyrene homopolymer in ethanol. European Polymer Journal. 2005; 41:2671–2677.


16. Li P, Yin YL, Li D, Kim SW, Wu G. Amino acids and immune function. Br J Nutr. 2007; 98:237–252. PMID: 17403271.


17. Kidd MT, Kerr BJ, Anthony NB. Dietary interactions between lysine and threonine in broilers. Poult Sci. 1997; 76:608–614. PMID: 9106889.


18. Konashi S, Takahashi K, Akiba Y. Effects of dietary essential amino acid deficiencies on immunological variables in broiler chickens. Br J Nutr. 2000; 83:449–456. PMID: 10858703.
19. Yang SR, Lee HJ, Kim JD. Histidine-conjugated poly(amino acid) derivatives for the novel endosomolytic delivery carrier of doxorubicin. J Control Release. 2006; 114:60–68. PMID: 16828916.


20. Xu Q, An L, Yu M, Wang S. Design and synthesis of a new conjugated polyelectrolyte as a reversible ph sensor. Macromolecular Rapid Communications. 2008; 29:390–395.


21. Jeong JH, Park TG. Poly(L-lysine)-g-poly(D,L-lactic-co-glycolic acid) micelles for low cytotoxic biodegradable gene delivery carriers. J Control Release. 2002; 82:159–166. PMID: 12106986.


22. Stone WL, Mukherjee S, Smith M, Das SK. Therapeutic uses of antioxidant liposomes. Methods Mol Biol. 2002; 199:145–161. PMID: 12094566.


23. Stone WL, Smith M. Therapeutic uses of antioxidant liposomes. Mol Biotechnol. 2004; 27:217–230. PMID: 15247495.


24. Chen CH, Liu DZ, Fang HW, Liang HJ, Yang TS, Lin SY. Evaluation of multi-target and single-target liposomal drugs for the treatment of gastric cancer. Biosci Biotechnol Biochem. 2008; 72:1586–1594. PMID: 18540096.


25. Koike M, Ishino K, Kohno Y, Tachikawa T, Kartasova T, Kuroki T, Huh N. DMSO induces apoptosis in SV40-transformed human keratinocytes, but not in normal keratinocytes. Cancer Lett. 1996; 108:185–193. PMID: 8973593.


26. Paromov V, Kumari S, Brannon M, Kanaparthy NS, Yang H, Smith MG, Stone WL. Protective effect of liposome-encapsulated glutathione in a human epidermal model exposed to a mustard gas analog. J Toxicol. 2011; 2011:109516. PMID: 21776256.


27. Kim SI, Min SK, Kim JH. Synthesis and characterization of novel amino acid-conjugated poly(aspartic acid) derivatives. Bull Korean Chem Soc. 2008; 29:1887–1892.
28. Lee JK, Lee MK, Yun YP, Kim Y, Kim JS, Kim YS, Kim K, Han SS, Lee CK. Acemannan purified from Aloe vera induces phenotypic and functional maturation of immature dendritic cells. Int Immunopharmacol. 2001; 1:1275–1284. PMID: 11460308.


29. Lee YR, Lee YH, Im SA, Kim K, Lee CK. Formulation and characterization of antigen-loaded plga nanoparticles for efficient cross-priming of the antigen. Immune Netw. 2011; 11:163–168. PMID: 21860609.


30. Im SA, Lee YR, Lee YH, Oh ST, Gerelchuluun T, Kim BH, Kim Y, Yun YP, Song S, Lee CK. Synergistic activation of monocytes by polysaccharides isolated from Salicornia herbacea and interferon-gamma. J Ethnopharmacol. 2007; 111:365–370. PMID: 17204386.
31. Lee YH, Lee YR, Kim KH, Im SA, Song S, Lee MK, Kim Y, Hong JT, Kim K, Lee CK. Baccatin III, a synthetic precursor of taxol, enhances MHC-restricted antigen presentation in dendritic cells. Int Immunopharmacol. 2011; 11:985–991. PMID: 21354357.


32. Talmor M, Mirza A, Turley S, Mellman I, Hoffman LA, Steinman RM. Generation or large numbers of immature and mature dendritic cells from rat bone marrow cultures. Eur J Immunol. 1998; 28:811–817. PMID: 9541575.


33. Mora JR. Homing imprinting and immunomodulation in the gut: role of dendritic cells and retinoids. Inflamm Bowel Dis. 2008; 14:275–289. PMID: 17924560.


34. Cai Z, Brunmark AB, Luxembourg AT, Garcia KC, Degano M, Teyton L, Wilson I, Peterson PA, Sprent J, Jackson MR. Probing the activation requirements for naive CD8+ T cells with Drosophila cell transfectants as antigen presenting cells. Immunol Rev. 1998; 165:249–265. PMID: 9850865.


35. Drutman SB, Trombetta ES. Dendritic cells continue to capture and present antigens after maturation in vivo. J Immunol. 2010; 185:2140–2146. PMID: 20644175.


36. Karlsen M, Hovden AO, Vogelsang P, Tysnes BB, Appel S. Bromelain treatment leads to maturation of monocyte-derived dendritic cells but cannot replace PGE2 in a cocktail of IL-1β, IL-6, TNF-α and PGE2. Scand J Immunol. 2011; 74:135–143. PMID: 21449940.


Figure 1
PLA-NLs increase the generation of BM-DCs. Mouse BM-DCs were generated from C57BL/6 mouse BM cells. BM cells were cultured with the indicated concentration of PLA-NLs and GM-CSF (40 ng/ml). PLA-NLs were added to the culture on day 4 after the initiation of the BM cell culture, and the resultant BM-DCs were harvested on day 8 and counted using a hemocytometer. Results are mean±SD. *p<0.05 compared with untreated control.
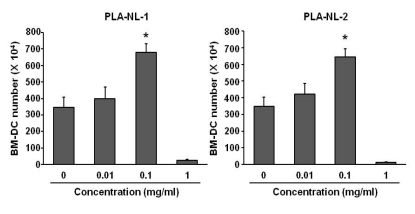
Figure 2
PLA-NLs induce phenotypic maturation of BM-DCs. PLA-NLs (0.1 mg/ml) were added to BM cell culture together with GM- CSF (40 ng/ml) on day 4 after the initiation of cultures, and the resultant BM-DCs were harvested on day 8, washed, and then used for immunophenotypic analysis. Levels of expression (thin line) in BM-DCs generated in the presence of GM-CSF and PLA-NLs are illustrated in comparison with those in BM-DCs generated in the presence of GM-CSF only (shaded line).
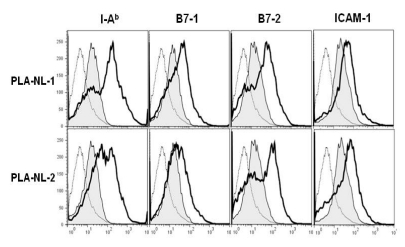
Figure 3
DCs generated in the presence of PLA-NLs exert increased capability to produce cytokines. PLA-NLs (0.1 mg/ml) were added to BM cell cultures together with GM-CSF (40 ng/ml) on day 4 after the initiation of cultures, and the resultant BM-DCs were harvested on day 8 and then cultured with OVA-containing nanoparticles (50 µg/ml as OVA). After 24 h, cytokines were measured by ELISA. Results are mean±SD. *p<0.05, **p<0.01 compared with untreated control.
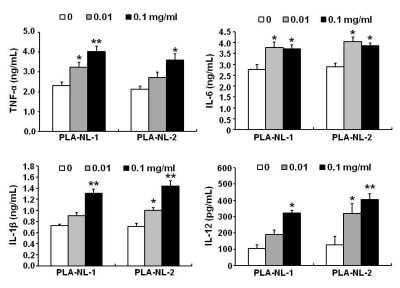
Figure 4
DCs generated in the presence of PLA-NLs exert increased allostimulatory capacity. PLA-NLs (0.1 mg/ml) were added to BM cell cultures together with GM-CSF (40 ng/ml) on day 4 after the initiation of cultures, and the resultant BM-DCs were harvested on day 8. All BM-DCs were treated with mitomycin C, washed, and then cultured with allogeneic mouse lymphocytes. After 3 days, cell growth was measured by [3H]-thymidine incorporation. Results are mean±SD. *p<0.01 compared with untreated control.
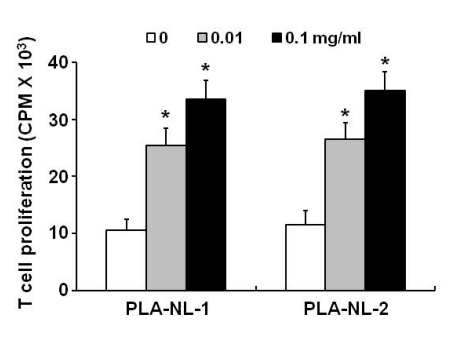
Figure 5
PLA-NLs induce the proliferation of GM-CSF-generated BM-DCs. The DCs generated in the presence of 40 ng/ml of GM-CSF only were harvested on day 8, and then cultured with the indicated concentration of PLA-NLs. After 2 days, the growth of BM-DCs was measured by [3H]-thymidine incorporation. Results are mean±SD. *p<0.05, **p<0.01 compared with untreated control.
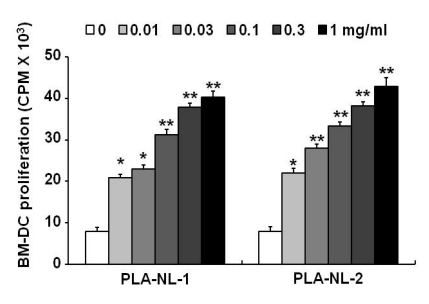