Abstract
Background
The ligand for CD137 (CD137L; also called 4-1BBL) is mainly expressed on activated APCs such as dendritic cells, B cells and macrophages. Even though CD137L functions as a trigger of the CD137 signaling pathway for T cell activation and expansion, engagement of CD137L can deliver a signal leading to the production of proinflammatory cytokines in macrophages.
Methods
We generated cell-permeable TAT-CD137L cytoplasmic domain fusion protein (TAT-CD137Lct) and examined its ability to initiate the CD137L reverse signaling pathway.
Results
Treatment of TAT-CD137Lct induced the production of high levels of IL-6 and TNF-α mRNAs and proteins in peritoneal macrophages. TAT-CD137Lct increased phosphorylation of Erk, p38 MAPK and Jnk, and activated transcription factors C/EBP and CREB. However, TAT-CD137Lct did not visibly affect the degradation of the inhibitor of NF-kB (IkBα). We further demonstrated that JNK activation was required for TAT-CD137Lct-induced production of TNF-α, while activation of Erk and p38 MAPK were involved in IL-6 and TNF-α production.
There is evidence for the existence of reverse signaling pathways of TNF superfamily members (1), including CD137L (2,3). CD137L reverse signals have been shown to mediate various cellular responses not only in immune cells such as monocytes/macrophages (4-8), dendritic cells (9,10), T cells (11,12), and B cells (13,14) but also in non-immune cells such as endothelial cells (15) and carcinoma cells (16,17). Biological activities that are mediated by CD137L reverse signals have been characterized most in details in monocytes/macrophages. For example, engagement of CD137L in monocytes/macrophages increases their migration and proinflammatory cytokine production (4-8,15,18,19). In addition, evidence is accumulating that CD137L signals are important for myelopoiesis. It has been clearly demonstrated that CD137L signals negatively regulate myelopoiesis (20). However, in vitro experiments using CD137-Fc fusion protein have shown that stimulation of the CD137L signaling promotes proliferation of hemaopoietic progenitor cells and their differentiation into monocytes (21,22).
To trigger the CD137L reverse signaling without blocking the CD137 receptor signaling pathway, we generated fusion protein consisting of the CD137L cytoplasmic domain and the protein transduction domain (PTD) of HIV TAT (48-57 amino acid residues: GRKKRRQRRR) (TAT-CD137Lct). A delivery tool using TAT is widely used to modulate various cellular activities (23). We showed that the TAT-CD137Lct evoked active CD137L reverse signaling in peritoneal macrophages. Our results suggest that recombinant TAT-CD137Lct protein should be used as an effective activator for the CD137L reverse signaling pathway without interfering with interactions between CD137 and CD137L.
C57BL/6 mice, 6-8 wk of age, were purchased from Hyo Chang Bioscience (Korea). Peritoneal macrophages were isolated by peritoneal larvage with 1x PBS (WelGENE, Korea). Mixture containing macrophages and RBCs was incubated in RBC lysis buffer for 5 min on ice and washed with 1x PBS three times. After that, cells were counted using hemocytometer and cultured in DMEM (WelGENE) containing 1% FBS (WelGENE). The following Abs were used for immunoblotting: Abs to p-p38, p38, p-Erk, Erk (all from Cell signaling Technology, Danvers, MA), IkBα (Santa Cruz Biotechnology), GAPDH (Millipore), and His (Santa Cruz Biotechnology, Santa Cruz, CA). Erk inhibitor (PD98059), p38 inhibitor (SB203580), and Jnk inhibitor (SP600125) were purchased from Merk (Germany).
Total RNA isolated using TRIzol reagent (Invitrogen, Carlsbad, CA) from mouse spleens was subjected to cDNA synthesis using Olig dT and AMV reverse transcriptase (Promega, Madison, WI). The cytoplasmic domain of mouse CD137L gene was amplified by PCR using sense (5'-GAATTCATGG ACCAGCACACA-3') and antisense primers (5'-CTCGAGTCAT GGGTGGCGGGA-3'). CD137Lct DNA fragments were inserted in pET21a-HisTAT vector (TAT-CD137Lct). To construct pET21a-HisTAT vector, double-stranded oligonucleotide including his-tag and TAT sequences was prepared by annealing (heating at 95℃ for 5 min and chilling at room temperature for 60 min) of single-strand oligonucleotides (Forward, 5'-CAT ATG CAC CAC CAC CAC CAC CAC TAT GGC AGG AAG-3'; Reverse, 5'-GGA TCC TCG TCG TCG CTG TCT CCG CTT CTT CCT GCC-3') and then inserted in pET21a vector. EGFP sequence was obtained from pEGFPN1 vector (Clonetech Laboratories, Mountain View, CA) by PCR amplification using the following primers: sense, 5'-GAA TTC ATG AGT AAA GGA GAA-3'; antisense, 5'-CTC GAG TTT GTA TAG TTC ATC-3'.
BL12 star E. coli (Merk, Germany) were transformed with each DNA construct, cultured overnight, and added with 1 mM isopropyl-β-D-thiogalactopyranoside (IPTG) for protein induction at 37℃ for 6 h. Harvested cells were sonicated in lyisis buffer (50 mM NaH2PO4, 300 mM NaCl, 10 mM imidazole). Lysates were clarified by centrifugation at 13,000 rpm for 20 min at 4℃. Clarified lysates were bound with Ni-NTA agarose bead (QIAGEN, Valencia, CA) for 1 h. Bound proteins were washed 5 times with washing buffer (50 mM NaH2PO4, 300 mM NaCl, 50 mM imidazole) and eluted with elution buffer (50 mM NaH2PO4, 300 mM NaCl, 250 mM imidazole). Purified proteins were dialysed using 1x PSB (pH 8.0) and LPS was removed using ToxinEsaer TM Endotoxin Removal kit (GeneScript, Piscataway, NY). Protein concentration was measured using bicinchoninic acid (BCA) kit (Thermo Scientific, Rockford, IL).
Peritoneal macrophages (1×106) were incubated with 10 µM TAT-EGFP, CD137Lct, TAT-CD137Lct and TAT-CD137Lct-EGFP for 3 min. Cells were lysed in lysis buffer (50 mM Tris-HCl [pH7.4], 150 mM NaCl, 1 mM EDTA, 1% NP-40, 1 mM PMSF, protease inhibitors, 1 mM NaF, and 0.1 mM Na3VO4) on ice for 30 min. Proteins were resolved in 17% SDS-PAGE and transferred onto nitrocellulose membranes. Immunoblot procedure was described in the next section. Transduced proteins were detected by anti-his Abs. Intracellular localization of TAT-EGFP and TAT-CD137Lct-EGFP protein was visualized by confocal microscopy. Cells were cytospinned at 1,000 rpm for 15 min on slide glass. Cytospinned cells were mounted with Fluromount-G solution (Southern Biotech).
Protein lysates were prepared from peritoneal macrophages and their protein concentrations were determined using the Bio-Rad assay kit (Hercules, CA). Lysates (5 µg of protein per sample) were mixed with gel loading buffer (60 mM Tris/HCl, pH 6.8, 25% glycerol, 2% SDS, 14.4 mM β-mercaptoethanol, 0.1% bromphenol blue) and boiled for 3 min. Proteins were resolved in 15% or 17% SDS-PAGE and transferred to nitrocellulose membranes (Millipore, Bedford, MA). Membranes were blocked for 1 h in blocking buffer (1X TBST [50 mM Tris-HCl, pH 7.5, 150 mM NaCl] including 5% dried milk), followed by overnight incubation at 4℃ with first Abs. Membranes were washed 3 times with 1X TBST and incubated with secondary Abs conjugated with alkaline phosphatase (Santa Cruz Biotechnology) for 1 h at room temperature and developed by ECL-plus substrate kit (Amersham Biosciences, Piscataway, NJ).
Peritoneal macrophages (1×105) were treated with 20 ng TAT-EGFP, CD137Lct, TAT-CD137Lct, and TAT-CD137Lct-EGFP for 4 h. Culture supernatants were collected and the amount of IL-6 and TNF-α was quantified using a CBA kit (BD Biosciences, San Diego, CA) with a FACS-Caliber cytometer equipped with Cell-QUEST-PRO and CBA software according to the manufacturer's instructions.
Total RNA isolated using TRIzol reagent (Invitrogen) was subjected to cDNA synthesis using Olig dT and AMV reverse transcriptase (Promega). Dilutions (5- or 10-fold) of cDNA reaction mixtures were subjected to PCR amplification using the following primers: IL-6 sense, 5'-GATGCTACCAAACTGGATA TAATC-3'; IL-6 antisense, 5'-GGTCCTTAGCCACTCCTTCTGTG-3'; TNF-α sense, 5'- AGGGGCCACCACGCTCTT CT-3'; TNF-α antisense, 5'- CGGGGCAGCCTTGTCCCTTG-3'; GAPDH sense, 5'-TGAAGGTCGGTGTGAACGGATTTG-3'; GAPDH antisense, 5'-CATGTAGGCCATGAGG TCCACCAC-3'.
Nuclear extracts were prepared from 1×106 of peritoneal macrophages stimulated with 50 ng TAT-EGFP, CD137Lct and TAT-CD137Lct proteins. Cells were washed with ice-cold PBS, resuspended in buffer A (20 mM HEPES, pH 7.9, 1.5 mM MgCl2, 10 mM KCl, 0.1 mM EDTA, 0.5 mM dithiothreitol, and 0.5 mM phenylmethylsulfonyl fluoride), and left on ice for 10 min. Nuclei were pelleted by centrifugation at 5,000 rpm for 10 min at 4℃ and resuspended in buffer B (20 mM HEPES, pH 7.9, 1.5 mM MgCl2, 420 mM NaCl, 0.2 mM EDTA, 20% glycerol, and 1 mM dithiothreitol). After incubation for 30 min at 4℃, the mixture was centrifuged at 13,000 rpm for 15 min at 4℃. The supernatant as nuclear extract was collected and stored at -70℃ until use. The C/EBP (sc-2525) and CREB (sc-2504) probe was purchased from Santa Cruz. Each probe was end-labeled with [γ-32P]dATP (Amersham Biosciences) by T4 polynucleotide kinase (Promega). The nuclear extracts and probe were incubated for 30 min at room temperature in reaction buffer containing 10 mM HEPES, pH 7.9, 50 mM NaCl, 1 mM EDTA, 1 mM DTT, and 5% glycerol. Each reaction contained 0.02 unit of poly[dI-dC] (Sigma, St. Louis, MO). Reactions were electrophoresed on a 4% non-denaturing polyacrylamide gel in 0.5X Tris borate-EDTA buffer at 150 V for 2 h, and then analyzed by autoradiography.
We generated DNA constructs to produce polyhistidine-tagged TAT-CD137Lct, CD137Lct, TAT-CD137Lct-EGFP, and TAT-EGFP proteins in E. coli (Fig. 1A). Recombinant proteins purified using Ni-NTA agarose beads had expected molecular weights when separated in 13% SDS-PAGE gel (Fig. 1B). Western blot analysis showed that all TAT fusion proteins were able to be permeabilized into peritoneal macrophages (Fig. 1C). Transduced TAT-CD137Lct-EGFP was localized beneath the cell membrane of peritoneal macrophages and TAT-EGFP was found throughout the cytoplasm (Fig. 1D). Our results suggest that TAT fusion proteins could easily penetrate into the cell.
CD137L reverse signaling induces IL-6 and TNF-α production in monocytes (4). We investigated whether transduction of TAT-CD137Lct would result in the production of IL-6 and TNF-α in peritoneal macrophages. Peritoneal macrophages produced high levels of IL-6 and TNF-α in response to TAT-CD137Lct and TAT-CD137Lct-EGFP in a dose-dependent manner (Fig. 2A and B). A high concentration of CD137Lct slightly induced the production of IL-6 and TNF-α. This might be due to the ability of macrophages to uptake extracellular proteins, since TAT-EGFP had no effect on the cytokine production of macrophages. Heat-inactivated TAT-CD137Lct did not stimulate macrophages to produce IL-6 and TNF-α, suggesting that there was no LPS contamination. As expected, LPS and CD137-Fc (100 ng/ml) was potent in inducing the production of IL-6 and TNF-α in peritoneal macrophages. RT-PCR analysis showed that TAT-CD137Lct also significantly increased mRNA levels of IL-6 and TNF-α in peritoneal macrophages (Fig. 2C and D).
To further define the mechanism by which TAT-CD137Lct controls macrophage production of IL-6 and TNF-α, we investigated what intracellular signaling molecules are involved in this process. TAT-CD137Lct increased phosphorylation of the kinases Erk, p38 and JNK but had no visible effect on the degradation rate of the inhibitor of NF-κB (IκBα) (Fig. 3A). Consistent with this result, the production of IL-6 and TNF-α in peritoneal macrophages was inhibited specifically by the Erk (PD98059) and p38 (SB203580) inhibitors (Fig. 3B and C). Interestingly, the JNK inhibitor (SP600125) blocked the production of TNF-α but not IL-6 in TAT-CD137Lct- stimulated macrophages. We also found that TAT-CD137Lct strongly activated CREB and C/EBP in macrophages at 12 h after its treatment (Fig. 3D). Our data are consistent with previous studies showing that CD137L is required for activation of CREB and C/EBP but not NF-κB in macrophages (19).
It has been shown that interactions of cell surface CD137L with TLR4 increase LPS-induced cytokine production in macrophages (19). Since TAT-CD137Lct seemed to evoke the CD137L signaling pathway regardless of CD137L on the cell surface, we predicted that TAT-CD137Lct would have an additive effect with LPS on cytokine production in macrophages. Indeed, co-treatment of TAT-CD137Lct and LPS showed an additive effect on IL-6 and TNF production in peritoneal macrophages (Fig. 4).
In this study, we generated TAT-CD137Lct fusion protein that could penetrate into macrophages and trigger CD137L reverse signaling. This protein has experimental merits because it can initiate the intracellular CD137L signaling pathway without interferring with interactions between CD137 and CD137L. It would be difficult to discern the in vivo effect of CD137L engagement from that of blocking the CD137 signaling pathway if we used CD137-Fc fusion protein or anti-CD137L mAbs. Previous studies have shown that the cytoplasmic domain of CTLA-4 fused with PTD of human transcriptional factor Hph-1 is potent in inhibiting the activity of T cells (24,25). Cytoplasmic domains of cell surface molecules fused to PTD are a simple way to stimulate intracellular signaling pathways.
Recent studies suggest that CD137/CD137L interactions may participate in multiple stages of inflammation (26). The CD137L reverse signaling transduction pathway in APCs such as macrophages and dendritic cells is thought to be essential in the amplification loop for inflammation, since they can be activated by CD137L engagement to secrete cytokines and chemokines (4,10). CD137 and CD137L signals also play a role in inflammation of non-hematopoietic cells such as endothelial cells (15) and epithelial cells (manuscript in submission). CD137 and CD137L signals thus appear to have broad control over inflammation, a conclusion also supported by the CD137L-mediated control of myelopoiesis (20), which may reflect the need for sufficient numbers of inflammatory cells during acute inflammation.
In TAT-CD137Lct-stimulated macrophages, phosphorylation of Erk, p38, and Jnk was induced rapidly and inhibition of their phosphorylation reduced IL-6 and TNF-α production. Since TAT-CD137Lct did not induce NF-κB activation, early activation of the CD137L signaling may result in secretion of stored IL-6 and TNF-α. Since CD137Lct increased mRNA levels of IL-6 and TNF-α at 4 and 12 h after its treatment, we cannot exclude the possibility that CD137Lct activated NF-κB to elevate transcription for IL-6 and TNF-α. We found that CD137Lct also activated transcription factors CREB and C/EBP at 8 and 12 h after its treatment. It is presumable that activation of these transcription factors is required for late production of IL-6 and TNF-α in macrophages. Interestingly, cell surface CD137L promotes sustained production of TNF-α through activation of CREB and C/EBP in macrophages in response to TLR signals (19). Taken together, it seems that CD137L signals regulate proinflammatory cytokine production in macrophages in a tidal fashion.
Figures and Tables
Figure 1
Production and transduction of TAT fusion proteins. (A) Plasmid constructs of the TAT fusion proteins. (B) Purified proteins were separated on 13% SDS-PAGE and then stained with Commasie blue. (C, D) Transduction activity of purified proteins was analyzed using immunoblot (C) and confocal microscope (D). Peritoneal macrophages (1×106) were incubated with 10 µM of TAT-EGFP, CD137Lct, TAT-CD137Lct, and TAT-CD137Lct-EGFP for 30 min. Transduced proteins were detected using anti-his Abs (C). Intracellular localization of TAT-EGFP and TAT-CD137Lct-EGFP were observed using confocal microscopy (D). Scale bar is 10 µm.
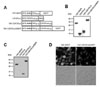
Figure 2
TAT-CD137Lct induces IL-6 and TNF production in peritoneal macrophages. (A, B) Peritoneal macrophages (1×105) were treated with indicated concentrations of TAT- EGFP, CD137Lct, TAT-CD137Lct, and TAT-CD137Lct-EGFP and cultured for 4 h. Culture medium was collected and levels of IL-6 (A) and TNF-α (B) were measured using a CBA kit. Heat-inactivated (HI) TAT-CD137Lct was used as a negative control and LPS (10 ng/ml) and CD137-Fc (100 ng/ml) as a positive control. Data are the mean±SD of triplicates. (C) Total RNA was extracted from peritoneal macrophages (1×106) that were treated with 20 ng of protein. Total RNA was subjected to cDNA synthesis. The TNF-α and IL-6 transcripts were amplified using specific primer sets and the PCR products were analyzed using 1.5% agarose gel electrophoresis. GAPDH mRNA expression was used as an internal control. (D) Density of amplified PCR product bands was analyzed using Image J program and normalized against the density of the GAPDH band.
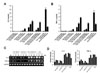
Figure 3
TAT-CD137Lct induced phosphorylation of Erk, p38, and Jnk and activaton of C/EBP and CREB. (A) Peritoneal macrophages (1×106) were treated with 50 ng of indicated proteins and harvested at 0, 15, 30, 60, and 120 min after their treatment. Lysates (10 µg) were used for immunoblot. LPS (50 ng/ml) is used as a positive control. p-, phosphorylated. (B, C) Peritoneal macrophages (1×105) were treated with TAT-CD137Lct (20 ng) in the presence of PD98059, SB203580, or SP600125 (10 µM each) and cultured for 4 h. Levels of IL-6 (B) and TNF (C) in culture medium were measured using a CBA kit. Data are the mean±SD of triplicates. (D) EMSA for nuclear extracts from peritoneal macrophages stimulated with 50 ng of indicated proteins was performed with radiolabeled C/EBP and CREB probes. LPS (50 ng/ml) is used as a positive control.
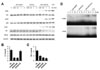
ACKNOWLEDGEMENTS
This work was supported by grants from National Research Foundation of Korea funded by the Ministry of Education, Science and Technology (2009-0073837).
References
1. Eissner G, Kolch W, Scheurich P. Ligands working as receptors: reverse signaling by members of the TNF superfamily enhance the plasticity of the immune system. Cytokine Growth Factor Rev. 2004. 15:353–366.


2. Schwarz H. Biological activities of reverse signal transduction through CD137 ligand. J Leukoc Biol. 2005. 77:281–286.


3. Shao Z, Schwarz H. CD137 ligand, a member of the tumor necrosis factor family, regulates immune responses via reverse signal transduction. J Leukoc Biol. 2011. 89:21–29.


4. Langstein J, Michel J, Fritsche J, Kreutz M, Andreesen R, Schwarz H. CD137 (ILA/4-1BB), a member of the TNF receptor family, induces monocyte activation via bidirectional signaling. J Immunol. 1998. 160:2488–2494.
5. Langstein J, Michel J, Schwarz H. CD137 induces proliferation and endomitosis in monocytes. Blood. 1999. 94:3161–3168.


6. Langstein J, Schwarz H. Identification of CD137 as a potent monocyte survival factor. J Leukoc Biol. 1999. 65:829–833.


7. Langstein J, Becke FM, Söllner L, Krause G, Brockhoff G, Kreutz M, Andreesen R, Schwarz H. Comparative analysis of CD137 and LPS effects on monocyte activation, survival, and proliferation. Biochem Biophys Res Commun. 2000. 273:117–122.


8. Ju SW, Ju SG, Wang FM, Gu ZJ, Qiu YH, Yu GH, Ma HB, Zhang XG. A functional anti-human 4-1BB ligand monoclonal antibody that enhances proliferation of monocytes by reverse signaling of 4-1BBL. Hybrid Hybridomics. 2003. 22:333–338.


9. Laderach D, Wesa A, Galy A. 4-1BB-ligand is regulated on human dendritic cells and induces the production of IL-12. Cell Immunol. 2003. 226:37–44.


10. Lippert U, Zachmann K, Ferrari DM, Schwarz H, Brunner E, Mahbub-Ul Latif AH, Neumann C, Soruri A. CD137 ligand reverse signaling has multiple functions in human dendritic cells during an adaptive immune response. Eur J Immunol. 2008. 38:1024–1032.


11. Schwarz H, Blanco FJ, von Kempis J, Valbracht J, Lotz M. ILA, a member of the human nerve growth factor/tumor necrosis factor receptor family, regulates T-lymphocyte proliferation and survival. Blood. 1996. 87:2839–2845.


12. Kwajah M M S, Mustafa N, Holme AL, Pervaiz S, Schwarz H. Biphasic activity of CD137 ligand-stimulated monocytes on T cell apoptosis and proliferation. J Leukoc Biol. 2011. 89:707–720.


13. Pauly S, Broll K, Wittmann M, Giegerich G, Schwarz H. CD137 is expressed by follicular dendritic cells and costimulates B lymphocyte activation in germinal centers. J Leukoc Biol. 2002. 72:35–42.
14. Pollok KE, Kim YJ, Hurtado J, Zhou Z, Kim KK, Kwon BS. 4-1BB T-cell antigen binds to mature B cells and macrophages, and costimulates anti-mu-primed splenic B cells. Eur J Immunol. 1994. 24:367–374.


15. Jeon HJ, Choi JH, Jung IH, Park JG, Lee MR, Lee MN, Kim B, Yoo JY, Jeong SJ, Kim DY, Park JE, Park HY, Kwack K, Choi BK, Kwon BS, Oh GT. CD137 (4-1BB) deficiency reduces atherosclerosis in hyperlipidemic mice. Circulation. 2010. 121:1124–1133.


16. Salih HR, Kosowski SG, Haluska VF, Starling GC, Loo DT, Lee F, Aruffo AA, Trail PA, Kiener PA. Constitutive expression of functional 4-1BB (CD137) ligand on carcinoma cells. J Immunol. 2000. 165:2903–2910.


17. Gullo C, Koh LK, Pang WL, Ho KT, Tan SH, Schwarz H. Inhibition of proliferation and induction of apoptosis in multiple myeloma cell lines by CD137 ligand signaling. PLoS One. 2010. 5:e10845.


18. Drenkard D, Becke FM, Langstein J, Spruss T, Kunz-Schughart LA, Tan TE, Lim YC, Schwarz H. CD137 is expressed on blood vessel walls at sites of inflammation and enhances monocyte migratory activity. FASEB J. 2007. 21:456–463.


19. Kang YJ, Kim SO, Shimada S, Otsuka M, Seit-Nebi A, Kwon BS, Watts TH, Han J. Cell surface 4-1BBL mediates sequential signaling pathways 'downstream' of TLR and is required for sustained TNF production in macrophages. Nat Immunol. 2007. 8:601–609.


20. Lee SW, Park Y, So T, Kwon BS, Cheroutre H, Mittler RS, Croft M. Identification of regulatory functions for 4-1BB and 4-1BBL in myelopoiesis and the development of dendritic cells. Nat Immunol. 2008. 9:917–926.


21. Jiang D, Chen Y, Schwarz H. CD137 induces proliferation of murine hematopoietic progenitor cells and differentiation to macrophages. J Immunol. 2008. 181:3923–3932.


22. Jiang D, Yue PS, Drenkard D, Schwarz H. Induction of proliferation and monocytic differentiation of human CD34+ cells by CD137 ligand signaling. Stem Cells. 2008. 26:2372–2381.


23. Asoh S, Ohta S. PTD-mediated delivery of anti-cell death proteins/peptides and therapeutic enzymes. Adv Drug Deliv Rev. 2008. 60:499–516.


24. Choi JM, Ahn MH, Chae WJ, Jung YG, Park JC, Song HM, Kim YE, Shin JA, Park CS, Park JW, Park TK, Lee JH, Seo BF, Kim KD, Kim ES, Lee DH, Lee SK, Lee SK. Intranasal delivery of the cytoplasmic domain of CTLA-4 using a novel protein transduction domain prevents allergic inflammation. Nat Med. 2006. 12:574–579.

