Abstract
Background
Hypersensitivity pneumonitis (HP) is an interstitial lung disease that develops following repeated exposure to inhaled particulate antigens. The family of Fcγ receptors (FcγRs) has emerged as central regulators for modulating both pro-and anti-inflammatory responses. However, the role of FcγRs in the development of HP has not been investigated yet.
Methods
To explore the functional roles of FcγRs in HP, FcγR-/- and B6 mice were challenged with Saccharopolyspora rectivirgula (SR) antigen intranasally, and compared these mice in terms of the histological change, infiltrated immune cells in BALF and in vitro immune responses.
Results
FcγR-/- mice exhibited attenuation of HP in terms of histological alterations, and reduced numbers of neutrophils and macrophages in and the increased CD4:CD8 ratio of bronchoalveolar lavage fluid. The lungs of FcγR-/- mice showed high production of Th2 cytokine such as IL-4 and slightly low production of Th1 cytokine, INF-γ compared to those of B6 mice. However, SR-specific adaptive immune responses of FcγR-/- mice were similar to those of B6 mice.
Hypersensitivity pneumonitis (HP) is a group of lung diseases that result from repeated pulmonary exposure to a variety of environmental organic dusts, fungi, and molds with uncertain immunological mechanisms (1). This disorder affects the parenchyma and the small airways of the lungs and presents an acute, subacute or chronic form. Consequently, HP may lead to irreversible pulmonary damages, which cause fibrosis or emphysema, and depend on several factors such as duration of exposure and nature of the inhaled antigens (2).
Although the pathogenesis of HP is incompletely understood, it has been generally accepted that a combination of humoral and cell-mediated immune responses contributes to the development of HP (3). Several studies have suggested that the murine models of experimental HP are mediated by Th1 immune responses. In mice, adoptive transfer of Th1 clones increased cell infiltration and caused reversible HP (4). Moreover, when the Th1/Th2 balance shifts toward Th1 immune responses, the HP is more severe whereas it is attenuated by Th2-biased responses (5,6). Beside T cells, various immune cell types including neutrophils, lymphocytes, monocytes, and macrophages have been known to be involved in the development of HP (7). Upon antigen challenge, a large number of alveolar macrophages infiltrate the lungs and secrete cytokines and chemokines such as TNF-α, IL-1, IL-8, and MIP-1α, which recruit neutrophils and lymphocytes (8,9). Chemokine-induced infiltration of neutrophils is found in the alveoli and followed by an influx of activated T cells with a predominance of CD8+ T cells (8). In Saccharopolyspora rectivirgula (SR)-induced HP of murine models, neutrophils are most prominent in bronchoalveolar lavage fluid (BALF) immediately after antigen challenge, and they produce a large amount of IFN-γ (10). Moreover, we previously demonstrated that NKT cells suppress IFN-γ-producing Gr-1+ granulocytes by producing IL-4, resulting in the attenuation of SR-induced HP (11). These observations suggest that macrophages, neutrophils and NKT cells may play an important role in development of HP.
Fcγ receptors (FcγRs) for IgG are surface glycoproteins and bridge humoral and cellular immunity by directing the interaction of antibodies with effector cells. These receptors are expressed on effector cells of the immune system and mediate phagocytosis, antibody-dependent cell-mediated cytotoxicity (ADCC), and activation of inflammatory cells (12,13). To date, four different classes of FcγRs (FcγRI, FcγRIIB, FcγRIII and FcγRIV) have been recognized in mice (14,15). FcγRIIB provides inhibitory signals, whereas FcγRI, FcγRIII, and FcγRIV act as activating receptors in immune cells (16). In mice, monocytes and macrophages express all activating and inhibitory FcγRs (FcγRI-FcγRIV), while neutrophils express the inhibitory FcγRIIB and the activating FcγRIII and FcγRIV. In addition, the expression of FcγRI, FcγRIIB, and FcγRIII dominates on dendritic cell (DC)s (17). In rodents, cell surface expression and signaling capacity of all activating FcγRs (FcγRI, FcγRIII, and FcγRIV) are dependent on the γ-chain. Therefore, genetic deletion of this subunit leads to loss of cell surface expression of FcγRs, resulting in functional deficiency of these activating FcγRs. Therefore, γ-chain of Fc receptor-knockout animals showed dramatically impaired antibody mediated effector cell responses (14,18-21).
During HP, immune complexes formed by soluble antigens and IgG antibodies trigger the complement cascade, resulting in activation of alveolar macrophage, in sequence, recruitment of neutrophils and lymphocytes (2). Furthermore, prominent humoral responses have been reported in pigeon fancier's disease, one of the most common HP in human (22), as revealed by the elevated levels of IgGs in BALF and the infiltration of B lymphocytes into interstitial and alveolar spaces (23,24). Based on these observations, we postulate that immune complexes of exposed antigens and IgGs activate neutrophils and macrophages via FcγRs, which may contribute to initiation of pulmonary inflammation upon SR antigen challenge. Therefore, in this study, we investigated the functional roles of FcγRs in the development of murine SR-induced HP using Fcγ-chain knockout mice.
C57BL/6 (B6) mice were purchased from Orient Company (Seoul, Korea) and FcγR-/- mice were purchased from Taconic (Germantown, NY). The mice were maintained in a specific pathogen-free environment at the AAALAC accredited the Clinical Research Institute of Seoul National University Hospital. All procedures involving animals were approved by the Institutional Animal Care and Use Committee of the Clinical Research Institute of Seoul National University Hospital.
SR antigen was prepared from a strain of SR obtained from the American Type Culture Collection (ATCC, catalog no. 29034, Manassas, VA), which was grown in a lysogeny broth (LB) in a 55℃ shaking incubator for 4 days, centrifuged, and rinsed with PBS three times. Next, SR antigen was homogenized and lyophilized. The SR antigen was re-suspended in pyrogen-free saline. SR antigen contained less than 20 ng/mg endotoxin, estimated using a limulus amebocyte lysate assay (Sigma Chemical, St. Louis, MO).
HP was induced by intranasal administration of 150µg SR antigen in saline into mice under light anesthesia using isoflurane. The procedure was performed on three consecutive days in each week for three weeks. To evaluate histological alteration of the lungs, paraffin sections of the lungs fixed in formalin were cut and stained with hematoxylin and eosin. Pathologic scores were defined as follows: 0, no lung abnormality; 1~5, the presence of inflammation and granulomas involving 10, 10~30, 30~50, 50~80, and >80% of the lungs, respectively.
To preparation BALF from mice, tracheas were cannulated and lungs were lavaged five times with 1ml of cold PBS. Each sample was then centrifuged at 2,000 rpm for 10 min at 4℃. The total number of cells was counted by a hemacytometer, and the samples were incubated with mAbs for 5 min on ice to block FcγRII/III. Without washing, these cells were stained in a 200µl total volume with a 1µg combination of the following mAbs: anti-CD8, CD4, TCR, B220, F4/80, NK1.1, CD11c, CD11b, and Gr-1 mAbs. These mAbs were purchased from BD bioscience (SanDiego, CA) or Ebioscience (San diego, CA). Data were acquired by FACS Calibar (Becton Dickinson, San Jose, CA, USA).
Diluted serum (1:10) was added to ELISA high-binding plates (Greiner, USA Scientific, Inc., Ocala, FL) coated with SR (500µg/ml) antigen in PBS (pH 7.4) and incubated overnight at 4℃. Next, alkaline phosphatase-conjugated goat anti-mouse IgG (Pierce, Rockford, IL) diluted in PBS/0.1% bovine serum albumin (BSA) (1:5,000) was added and the plates were incubated for 1 h at room temperature. The plates were then washed and 100µl of phosphatase substrate (ρ-nitrophenyl-phosphate in a carbonate buffer, pH 9.6) was added to each well. The absorbance of each sample was read at 405 nm using a BioTek ELx808 microplate reader and Gen5 software package (BioTek Instruments Inc., Winooski, VT).
The spleen cells were taken from B6 and FcγR-/- mice administered SR antigen for 3 weeks and cultured with filtered SR antigen. SR-specific immune cell proliferation was determined using MTS assay kits (Promega, Madison, WI).
For quantitative real-time PCR, whole lungs were homogenized and the total lung mRNA extracts was prepared using an RNeasy Mini Kit (Qiagen Korea Ltd., Seoul, Korea). Three micrograms of the RNA were then reverse transcribed using Moloney murine leukemia virus reverse transcriptase (Promega, Medison, WI, USA) was performed using the following primers and probes from Applied Biosystems (Foster City, CA): GAPDH (TaqMan Assay Reagent: 4352339E), IFN-γ (TaqMan Assay Reagent: Mm 00801778 m1), IL-4 (TaqMan Assay Reagent: Mm 00445259_m1), MIP-1alpha (TagMan Assay Reagent Mm 99999057_m1), MCP-1 (TagMan Assay Reagent; Mm 99999056_m1), IP-10 (TagMan Assay Reagent; Mm 99999072_m1) and RANTES (TagMan Assay Reagent; Mm 01302427_m1). The results for each cytokine were normalized with respect to GAPDH expression.
To investigate whether FcγRs play a role in the development of HP, we administrated SR antigen intranasally to B6 and FcγR-/- mice on three consecutive days. Seven days after the first SR antigen exposure, the immune cells in the lung tissues of B6 and FcγR-/- mice were examined. As shown in Fig. 1B, there was no significant difference in total cell numbers of BALF between B6 and FcγR-/- mice in SR-induced HP. However, the cellular composition of inflammatory cells in BALF of FcγR-/- mice differed from that of B6 mice (Fig. 1A and C). The numbers of macrophages and neutrophils were markedly reduced, while T, B, and dendritic cells were more recruited into the lungs of FcγR-/- mice than those in B6 mice. HP is characterized by a lymphocytic alveolitis showing an increase in both the percentage and absolute number of CD8+ T cells in the BALF (25). Therefore, the percentages of CD4+ and CD8+ T cells in the BALF from B6 and FcγR-/- mice were examined during SR-induced HP. The numbers of CD4+ and CD8+ T cells were increased in BALF of FcγR-/- mice compared with B6 mice (Fig. 1C). However, the percentage of CD8+ T cells among gated lymphocytes in BALF of FcγR-/- mice was similar to that of B6 mice in SR-induced HP, whereas the percentage of CD4+ T cells was much higher than that of B6 mice (Fig. 1D). Therefore, CD8:CD4 T cell ratio was reduced in BALF of FcγR-/- mice compared to B6 mice during SR-induced HP. Combined, these findings suggested that FcγR-deficeint innate immune cells such as neutrophils and macrophages less migrated into the lungs than those cells of B6 mice, whereas more T and B cells were recruited into the lungs of FcγR-/- mice compared to B6 mice during SR-induced HP.
To compare the phenotypes of the B6 and FcγR-/- mice with HP, we investigated the histological alterations in the lungs 3 weeks after the first SR antigen exposure. SR antigen induced multifocal pulmonary lesions characterized with peribronchial lymphocytic infiltration, alveolitis, interstitial fibrosis in B6 mice (Fig. 2A). In particular, the infiltration of neutrophils and macrophages in alveolar spaces and interstitium of B6 mice was prominent compared with FcγR-/- mice. In contrast, peribronchial hyperplasia of lymphoid follicles was more frequently found in FcγR-/- mice than in B6 mice. Consistent with histological alteration in these mice, the histological scorings of the pulmonary lesions revealed significant attenuation of HP in FcγR-/- mice compared with B6 mice (Fig. 2B).
Many studies have indicated that various cytokines and chemokines are important in the pathogenesis of inflammatory lung diseases (8,10,26,27). Cytokines and chemokines induced by administration of SR antigen mediate the recruitment of immune cells and induce differentiation of Th1 cells, resulting in granuloma formation. To evaluate cytokines and chemokines expression in FcγR-/- and B6 mice after SR antigen administration, we measured mRNAs of IFN-γ, IL-4, IP-10, Mip1α, RANTES, and MCP-1 in the lungs. The expression of IP-10, Mip1α, RANTES, and MCP-1 was reduced in FcγR-/- mice compared to B6 mice (Fig. 3A). Among these chemokines, the production of IP-10 was significantly different between B6 and FcγR-/- mice. Upon inoculation of SR-antigen, FcγR-/- mice exhibited the low levels of IFN-γ, whereas the transcript level of IL-4 was significantly increased (Fig. 3B). Therefore, FcγR-/- mice show decreased chemokines expression and Th2-dominant immune responses during SR-induced HP.
The immunologic responses to SR antigen in SR-induced HP can be assessed by measuring SR-specific immune cell proliferation, and IgG levels in sera and BALF of mice given SR antigen (2,28,29). To evaluate the immune responses against SR antigen, BALF and sera from B6 and FcγR-/- mice administered SR antigen three weeks after the first antigen exposure were obtained. FcγR-/- mice given SR antigen showed slightly higher serum SR-specific IgG levels (Fig. 4A), whereas B6 mice challenged with SR antigen showed slightly higher IgG production in BALF (Fig. 4B). However, we could not find statistically significant difference in the IgG levels both of sera and of BALF between B6 and FcγR-/- mice. To evaluate SR-specific immune cell proliferation, splenocytes from B6 and FcγR-/- mice were stimulated with SR antigen in vitro 24 h. The proliferation of immune cells against SR antigen showed no difference between SR-challenged B6 and FcγR-/- mice (Fig. 4C). These results indicated that activating FcγRs do not play a critical role in modulation of SR-induced adaptive immune responses.
During the development of HP, the inhaled antigen enters the alveolar spaces, crosses the alveolar endothelium, and binds to circulating antibody leading to immune complexes deposition within the lung (30). Moreover, these immune complexes activate various immune cells via engagement of IgG Fc receptors containing an activation motif (ITAM), resulting in initiation and propagation of an inflammatory response (31). Therefore, it has been suggested that FcγR play a critical role in the development of HP. Consistent with this suggestion, our experiments demonstrated that FcγR-/- mice exhibited an attenuated phenotype of HP in terms of histologic alterations compared with B6 mice, indicating that activating FcγRs contribute to the development of SR-induced HP. The activating FcγRs (FcγRI, III, and IV) are usually expressed on innate cells such as monocytes, macrophages, dendritic cells, whereas B cells express inhibitory FcγRIIB and none of FcγRs is detected on T cells (17). Thus, this expression pattern of FcγR on immune cells enables us to hypothesize that innate immune cells might be involved in FcγR-mediated immune responses of SR-induced HP rather than adaptive immune cells. Consistent with this hypothesis, the cellular compositions of BALF were strikingly different between FcγR-/- and B6 mice in SR-induced HP. Macrophages and neutrophils were significantly reduced in BALF of FcγR-/- mice compared to B6 mice, whereas T and B lymphocytes were increased. These findings indicate that FcγRs contribute to activation and recruitment of macrophages or neutrophils via immune complexes, resulting in attenuation of histopathology of lung parenchyma in FcγR-/- mice during SR-induced HP. HP has been considered to involve two pathologic mechanisms: immune complex-mediated immune response and T cell-mediated immune response (32). These mechanisms occur either simultaneously and/or independently at different stages of the disease. The former mechanism usually initiates and mediates the acute HP, while the latter mediates the subacute and chronic HP. Therefore, it is more likely that FcγR-mediated activation and recruitment of innate immune cells might be an early event of HP rather than occur at chronic phase of disease.
IFN-γ produced by T cells and neutrophils in the lungs contributes to the development of murine HP, categorized as a Th1 disease (5,33). Moreover, IFN-γ-producing neutrophils contribute to granuloma formation in the absence of IFN-γ-producing T cells during SR-induced HP (10). These findings indicate that innate immune cells more contribute to IFN-γ production and development of HP than adaptive immune cells do. In our experiments, the subset analysis of BALF revealed that neutrophils were remarkably reduced in BALF of FcγR-/- mice during SR-induced HP, which led us to hypothesize that small numbers of neutrohpils in the lungs of FcγR-/- mice produce small amount of IFN-γ, resulting in the marked reduction of IFN-γ production in the lungs. However, we did not detect statistically significant reduction of IFN-γ production in the lungs of FcγR-/- mice compared to B6 mice. Here, there are several lines of evidences to explain this discrepancy in IFN-γ production of FcγR-/- mice during SR-induced HP. First, in contrast to neutrophils, CD4+ T cells, known to be another main source of IFN-γ in the lungs during SR-induced HP, significantly increased in BALF of FcγR-/- mice upon SR antigen inoculation. These findings suggest that IFN-γ produced by CD4+ T cells might compensate reduced amount of IFN-γ produced by neutrophils during SR-induced HP. Second, we recently reported that IL-4-producing NKT cells play a protective role in SR-induced HP by suppressing IFN-γ-producing neutrophils (11). Moreover, NKT cells express an activating receptor FcγRIII that provides strong activating signals for activation of these cells (34-36). Our experiments in antibody-induced joint inflammation demonstrated that the engagement of FcγRIII via immune complexes activates NKT cells, resulting in modulation of joint inflammation (37). Thus, there is a possibility that signals via FcγRIII also contribute to activation of NKT cells during SR-induced HP, which suppresses IFN-γ-producing neutrophils in the lungs. Based on this possibility, we postulate that loss of activating signals via FcγRIII on NKT cells in FcγR-/- mice causes the reduction of suppressive effects on IFN-γ-producing neutrophils, resulting in enhancement of IFN-γ production by neutrophils during SR-induced HP. Combined, it is suggested that increased number of IFN-γ-producing CD4+ and CD8+ T cells in the lungs and loss of NKT cell-mediated suppression of IFN-γ-producing neutrophils in FcγR-/- mice compensate reduced IFN-γ production by FcγR-deficient neurtophils.
Considering clinical aspects of HP, the presence of serum precipitating antibodies or cell-mediated hypersensitivity against presumptive antigens may be helpful for the diagnosis of patients with HP (2,28,29). Moreover, in several studies using the SR-induced murine HP model, SR-spedific serum IgG or IL-2 production of BAL cells was measured to evaluate the adaptive immune responses in SR-induced HP (38,39). Therefore, to examine adaptive immune responses, we measured the SR-specific immune cell proliferation and the IgG levels both in sera and BALF. In contrast to histological alteration and cellular composition of BALF, there was no difference in adaptive immune responses between B6 and FcγR-/- mice upon SR-antigen exposure. However, our experiments demonstrated that the number of CD4+ and CD8+ T, and B cells in BALF of FcγR-/- mice increased compared to that of B6 mice. These findings suggest that adaptive immune responses induced by T and B cells against SR antigen are not affected in FcγR-/- mice although T and B cells more infiltrated into the lungs of FcγR-/- mice compared to B6 mice. Very recently, we reported that CD4+CD25+ regulatory T (Treg) cells attenuate SR-induced HP by suppressing IFN-γ-producing CD4+ and CD8+ T cells in the lungs via both direct cell-to-cell contact and IL-10 dependent manners (40). Even though the exact number and function of Treg cells needs to be determined in FcγR-/- and B6 mice, it is hypothesized that increased numbers or function of Treg cells upon SR angtigen challenge contributes to more suppression of CD4+ and CD8+ T cells in FcγR-/- mice upon SR antigen exposure, resulting in similar adaptive immune responses between FcγR-/- and B6 mice.
Avoidance of the causative antigens and steroid treatment are well-known treatment of patients with HP. However, some patients develop advanced pulmonary fibrosis despite of treatment (2). Therefore, alternative therapeutic modalities must be developed. Here, we propose a therapeutic approach using blockage of activating FcγRs based on our current research (11). However, it is likely that the blockage of FcγRs might attenuate the protective function of FcγRIII-expressing NKT cells on HP, which provides adverse effects on the treatment of HP. Therefore, a comprehensive approach should be considered to optimize therapeutic effects on HP.
In summary, our results demonstrate that activating signals through Fcγ receptors play an important role in the development of HP by provoking innate immune cells to mobilize and to produce cytokines in particular Th1 inducing cytokines. These results provide new insights into the complex immunological mechanisms underlying HP, and lead to the development of more effective therapeutic approaches for HP.
Figures and Tables
Figure 1
B6 and FcγR-/- mice show different cellular composition of BALF during SR-induced HP. BALF cells were obtained from mice 7 days after the first inoculation with SR antigen and counted. The cellular subset was evaluated using flow cytometric analysis. (A) FSC and SSC scatter diagrams of total BALF cells in B6 and FcγR-/- mice (B, C) The numbers of immune cells in BALF were counted and (D) the percentages of CD4+ and CD8+ T cells were determined among the gated lymphocytes in flow cytometric analysis. The results shown are representative of three independent experiments and statistical analysis was performed using the program Prism 4.0 (n=3, N.S.: not significant, *p<0.05, **p<0.01, B6 mice versus FcγR-/- mice).
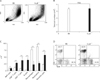
Figure 2
Hypersensitivity pneumonitis is attenuated in FcγR-/- mice compared to B6 mice in terms of histological alteration. In B6 and FcγR-/- mice, hypersensitivity pneumonitis was induced by inoculating SR antigen intranasally. These mice were sacrificed 3 weeks after HP induction. (A) The lungs were removed from the B6 and FcγR-/- mice, and paraffin sections were stained with H&E. Original magnification, ×100 or ×400. The photographs are representative of the three mice in each group. (B) Inflammatory responses in the lungs of B6 and FcγR-/- mice were graded. 0 (n=3, *p<0.05, B6 mice versus FcγR-/- mice).
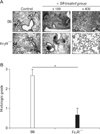
Figure 3
FcγR-/- mice alter chemokines and cytokines expression in the lungs during SR-induced HP. The transcription of chemokines such as IP-10, Mip-1α, RANTES and MCP-1 (A) and cytokines, IFN-γ, and IL-4 (B) were measured using real-time PCR in the lungs from B6 and FcγR-/- mice 7 days after the first administration of SR antigen. All expression levels were normalized to GAPDH. The results shown are representative of three independent experiments and statistical analysis was performed using the program Prism 4.0 (n=3, *p<0.05, B6 mice versus FcγR-/- mice).
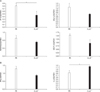
Figure 4
FcγR-/- mice show similar SR-specific proliferation of immune cells and production of IgG during SR-induced HP. HP was induced by intranasal inoculation of SR antigen. (A, B) The lungs were removed from the B6 and FcγR-/- mice 3 weeks after induction of HP, and the amounts of SR-specific IgG in BALF and serum were measured 3 weeks after HP induction using ELISA. (C) Immune cell proliferation to SR antigen was evaluated by a MTS assay using spleen cells 21 days after the first nasal inoculation with SR antigen. The results shown are representative of three independent experiments and statistical analysis was performed using the program Prism 4.0 (n=3, N.S.: not significant,, B6 mice versus FcγR-/- mice).
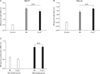
ACKNOWLEDGEMENTS
This study is supported by Seoul National University Hospital (SNUH) grant (04-2008-0370). We thank the Department of Experimental Animals at the Clinical Research Institute of Seoul National University Hospital for animal management.
References
1. Schatz M, Patterson R, Fink J. Immunopatholgenesis of hypersensitivity pneumonitis. J Allergy Clin Immunol. 1977. 60:27–37.
2. Patel AM, Ryu JH, Reed CE. Hypersensitivity pneumonitis: current concepts and future questions. J Allergy Clin Immunol. 2001. 108:661–670.


3. Girard M, Israël-Assayag E, Cormier Y. Pathogenesis of hypersensitivity pneumonitis. Curr Opin Allergy Clin Immunol. 2004. 4:93–98.


4. Irifune K, Yokoyama A, Kohno N, Sakai K, Hiwada K. T-helper 1 cells induce alveolitis but do not lead to pulmonary fibrosis in mice. Eur Respir J. 2003. 21:11–18.


5. Gudmundsson G, Hunninghake GW. Interferon-gamma is necessary for the expression of hypersensitivity pneumonitis. J Clin Invest. 1997. 99:2386–2390.


6. Ghadirian E, Denis M. Murine hypersensitivity pneumonitis: interleukin-4 administration partially abrogates the disease process. Microb Pathog. 1992. 12:377–382.


8. Schuyler M, Gott K, Cherne A. Mediators of hypersensitivity pneumonitis. J Lab Clin Med. 2000. 136:29–38.


9. Denis M. Proinflammatory cytokines in hypersensitivity pneumonitis. Am J Respir Crit Care Med. 1995. 151:164–169.


10. Nance S, Cross R, Yi AK, Fitzpatrick EA. IFN-gamma production by innate immune cells is sufficient for development of hypersensitivity pneumonitis. Eur J Immunol. 2005. 35:1928–1938.


11. Hwang SJ, Kim S, Park WS, Chung DH. IL-4-secreting NKT cells prevent hypersensitivity pneumonitis by suppressing IFN-gamma-producing neutrophils. J Immunol. 2006. 177:5258–5268.


12. Nimmerjahn F, Ravetch JV. Fcgamma receptors as regulators of immune responses. Nat Rev Immunol. 2008. 8:34–47.
13. Beaven MA, Metzger H. Signal transduction by Fc receptors: the Fc epsilon RI case. Immunol Today. 1993. 14:222–226.
14. Nimmerjahn F, Ravetch JV. Divergent immunoglobulin g subclass activity through selective Fc receptor binding. Science. 2005. 310:1510–1512.


15. Nimmerjahn F. Activating and inhibitory FcgammaRs in autoimmune disorders. Springer Semin Immunopathol. 2006. 28:305–319.
16. Nimmerjahn F, Ravetch JV. Fcgamma receptors: old friends and new family members. Immunity. 2006. 24:19–28.
18. Uchida J, Hamaguchi Y, Oliver JA, Ravetch JV, Poe JC, Haas KM, Tedder TF. The innate mononuclear phagocyte network depletes B lymphocytes through Fc receptor-dependent mechanisms during anti-CD20 antibody immunotherapy. J Exp Med. 2004. 199:1659–1669.


19. Takai T, Li M, Sylvestre D, Clynes R, Ravetch JV. FcR gamma chain deletion results in pleiotrophic effector cell defects. Cell. 1994. 76:519–529.


20. Clynes R, Takechi Y, Moroi Y, Houghton A, Ravetch JV. Fc receptors are required in passive and active immunity to melanoma. Proc Natl Acad Sci U S A. 1998. 95:652–656.
21. Clynes R, Ravetch JV. Cytotoxic antibodies trigger inflammation through Fc receptors. Immunity. 1995. 3:21–26.


22. McSharry C, Anderson K, Boyd G. A review of antigen diversity causing lung disease among pigeon breeders. Clin Exp Allergy. 2000. 30:1221–1229.


23. Reynolds SP, Edwards JH, Jones KP, Davies BH. Immunoglobulin and antibody levels in bronchoalveolar lavage fluid from symptomatic and asymptomatic pigeon breeders. Clin Exp Immunol. 1991. 86:278–285.


24. Drent M, van Velzen-Blad H, Diamant M, Wagenaar SS, Hoogsteden HC, van den Bosch JM. Bronchoalveolar lavage in extrinsic allergic alveolitis: effect of time elapsed since antigen exposure. Eur Respir J. 1993. 6:1276–1281.
25. Semenzato G, Agostini C, Zambello R, Trentin L, Chilosi M, Pizzolo G, Marcer G, Cipriani A. Lung T cells in hypersensitivity pneumonitis: phenotypic and functional analyses. J Immunol. 1986. 137:1164–1172.
26. Oshima M, Maeda A, Ishioka S, Hiyama K, Yamakido M. Expression of C-C chemokines in bronchoalveolar lavage cells from patients with granulomatous lung diseases. Lung. 1999. 177:229–240.


27. Nance S, Cross R, Fitzpatrick E. Chemokine production during hypersensitivity pneumonitis. Eur J Immunol. 2004. 34:677–685.


29. Hisauchi-Kojima K, Sumi Y, Miyashita Y, Miyake S, Toyoda H, Kurup VP, Yoshizawa Y. Purification of the antigenic components of pigeon dropping extract, the responsible agent for cellular immunity in pigeon breeder's disease. J Allergy Clin Immunol. 1999. 103:1158–1165.


30. Woda BA. Hypersensitivity pneumonitis: an immunopathology review. Arch Pathol Lab Med. 2008. 132:204–205.


31. Ravetch JV, Clynes RA. Divergent roles for Fc receptors and complement in vivo. Annu Rev Immunol. 1998. 16:21–132.
32. Suga M, Yamasaki H, Nakagawa K, Kohrogi H, Ando M. Mechanisms accounting for granulomatous responses in hypersensitivity pneumonitis. Sarcoidosis Vasc Diffuse Lung Dis. 1997. 14:131–138.
33. Schuyler M, Gott K, Edwards B. Th1 cells that adoptively transfer experimental hypersensitivity pneumonitis are activated memory cells. Lung. 1999. 177:377–389.


35. Koyasu S. CD3+CD16+NK1.1+B220+ large granular lymphocytes arise from both alpha-beta TCR+CD4-CD- and gamma-delta TCR+CD4-CD8- cells. J Exp Med. 1994. 179:1957–1972.


36. Bendelac A. CD1: presenting unusual antigens to unusual T lymphocytes. Science. 1995. 269:185–186.


37. Kim HY, Kim S, Chung DH. FcgammaRIII engagement provides activating signals to NKT cells in antibody-induced joint inflammation. J Clin Invest. 2006. 116:2484–2492.
38. Israël-Assayag E, Fournier E, Cormier Y. Blockade of T cell costimulation by CTLA4-Ig inhibits lung inflammation in murine hypersensitivity pneumonitis. J Immunol. 1999. 163:6794–6799.
39. Gudmundsson G, Monick MM, Hunninghake GW. Viral infection modulates expression of hypersensitivity pneumonitis. J Immunol. 1999. 162:7397–7401.