Abstract
Background
The MK1 strain, a novel bacterial isolate from soft-rotten tissue of the Neungee mushroom, produces copious amounts of exopolysaccharide (EPS) in a dextrose minimal medium. This study examined the molecular characteristics and immunomodulatory activity of MK1 EPS.
Methods
The EPS in the culture supernatant was purified by cold ethanol precipitation, and characterized by SDS-PAGE/silver staining and Bio-HPLC. The immunomodulatory activities of the EPS were examined using the mouse monocytic cell line, RAW 264.7 cells.
Results
The molecular weights of the purified EPS were rather heterogeneous, ranging from 10.6 to 55 kDa. The EPS was composed of glucose, rhamnose, mannose, galactose, and glucosamine at an approximate molar ratio of 1.00:0.8:0.71:0.29:0.21. EPS activated the RAW cells to produce cytokines, such as TNF-α and IL-1β, and nitric oxide (NO). EPS also induced the expression of co-stimulatory molecules, such as B7-1, B7-2 and ICAM-1, and increased the phagocytic activity. The macrophage-activating activity of EPS was not due to endotoxin contamination because the treatment of EPS with polymyin B did not reduce the macrophage-activating activity.
Microbial exopolysaccharides (EPS) are a polycarbohydrate produced and excreted by microorganisms. There are a various types of EPS, either homopolysacharides (e.g. cellulose, dextran, mutan, alternant, pullulan, levan and curdlan) and heteropolysaccharides (e.g. gellan and xanthan) depending on the types of repeating subunit (1). Microbial EPSs are either capsular polysaccharide bound covalently to the cell surface or slime polysaccharides bound loosely to the cell surface (2). Microbial EPSs play a number of indispensable roles in self-protection against harsh environments, such as osmotic stress, desiccation, UV radiation, antimicrobial substances, toxic compounds, and bacteriophage attack (3). In addition, microbial EPSs allow the cells to adhere other bacteria, animal and plant tissues or inert surfaces, thereby forming biofilms (4). Owing to the properties of EPSs, such as water retention, film-forming, texture-enhancing, thickening, water binding, gelling, and suspending, various microbial EPSs are expected to have immense value in detergents, adhesives, heavy metal removal, microbial enhanced oil recovery, wastewater treatment, cosmetics, pharmaceuticals, brewing, food etc. (5). For example, Enterobacter cloaceae EPS showing good viscosity, even at high temperatures, makes it a good candidate for microbial enhanced oil recovery (6). Gelrite obtained from Pseudomonas spp. is a new gelling polysaccharide with good thermal stability and clarity (7). Xanthan gum produced by Xanthomonas campestris has a wide range of applications as a thickening and stabilizing agent, particularly in the food industry, cosmetics, paper milling, textiles, and pharmaceutical sector as well as in enhanced oil recovery (8). In addition, cell-bound EPS produced by marine bacterium, Zooglea sp., was reported to adsorb metal ions, such as chromium, lead, and iron in solution (9). In addition, the great value of microbial EPSs would be associated with their various physiological activities, such as anti-tumor, anti-viral, anti-inflammation, inducer of interferon production, platelet aggregation inhibition, and colony stimulating factor. For example, the EPSs produced by either marine Vibrio or Pseudomonas sp. have anti-tumor, antiviral, and immunostimulating activities (10,11). EPS produced by Bifidobacterium longum promotes its colonization on the inner surface of the intestine (12,13) and has a strong anti-mutagenesis effect (14). EPS produced by Bacillus licheniformis has antiviral and immunoregulatory activities (15). EPS produced by Lactococcus lactis sp. cremoris KVS20 exhibits bioactivity, such as lymphocyte mitogenicity (16-19), macrophage cytostaticity (20), and cytokine (IFN-γ and IL-1β) production in macrophages (21). The MK1 strain, a bacterial isolate originated from the soft-rotten tissue of the Neungee gill, appears to be a novel bacterium (22,23). The most peculiar feature of the heterotrophic MK1 strain produces copious amounts of EPS in a dextrose medium (24).
In this study, EPS was purified and characterized by SDS-PAGE/silver staining and Bio-HPLC. The purified EPS exhibited immunomodulatory activity on the mouse monocytic cell line.
Carbon sources consumed in minimal salt medium and chemicals, such as acetic acid, formaldehyde, sodium thiosulfate, silver nitrate, and sodium carbonate, used in the silver staining of EPSs were purchased from Sigma-Aldrich Inc. (St. Louis, MO, USA). The size marker kit used to determine the total EPS molecular weight was obtained from Fermentas Inc. (Burlington, Canada). Ethanol for EPS purification and sulfuric acid needed to determine the glucose equivalent EPS were supplied by Merck Ltd. (Bangkok, Thailand). Alcian blue 8GX for the quantitation of the acidic sugar in EPS was acquired from Aldrich Chemical Co. (Milwaukee, WI, USA).
Ten ml of the MK1 cells grown overnight in a Luria-Bertani medium (LB, 1.0% tryptone, 0.5% yeast extract, and 0.5% sodium chloride) were inoculated into a 500 ml of basal minimal glucose medium and incubated for 3 days at 30℃ with continuous shaking at 150 rpm. The basal minimal glucose medium (g/L) was composed of glucose 20.0, Na2HPO4 4.8, KH2PO4 4.4, (NH4)2SO4 1.0, MgSO4·7H2O 0.5, CaCl2 0.05, and FeCl3 0.005. The initial pH of the minimal salt medium was adjusted to pH 7.0 by adding Na2HPO4 and KH2PO4. Bacterial growth was monitored by determining the optical density at 600 nm (DU-65 Spectrophotometer, Beckman, Fullerton, CA, USA).
The supernatant containing EPS was obtained from the MK1 cell culture by centrifugation (SUPRA 22K, Hanil, Korea) at 6,520×g for 20 min at 4℃. The EPS in the supernatant was precipitated with three volumes of absolute cold ethanol followed by incubation at 4℃ for 24 hr. The ethanol precipitated EPS was collected by centrifugation for 15 min at 4,520×g, at 4℃, and the EPS precipitate was suspended in 2 ml of D-H2O. The EPS was washed three times with cold ethanol and freeze-dried (Ilsin® Lab., Korea) at -50℃ for 2 days (25). This freeze-dried EPS was used for the morphological observations by optical microscopy and scanning electron microscopy (SEM, S-2500C, Hitachi, Japan). The dried EPS was adjusted with D-H2O to a final volume of 5 ml and dialyzed (M.W. cut off 1,000 Da) against 36 L of D-H2O for 3 days at 4℃ with four changes per day. The EPS in the dialyzed preparation was recovered by cold ethanol precipitation and freeze-drying, as described above. The dried EPS was used for the EPS quantitation, sugar composition analysis, etc.
To express the quantity of EPS, both amounts of glucose (glucose-equivalent EPS) and acidic sugar (acidic sugar equivalent EPS) were assayed using the phenol-sulfuric acid method (26,27) and alcian blue binding (25,28), respectively. The glucose-equivalent EPS was determined using the standard curve constructed with different glucose concentrations (100, 200, 300, 400, 500 µg/ml). Alcian blue (a cationic copper phthalocyanine dye) binds to the anionic carboxyl and half-ester sulfate groups of acidic EPS to form an insoluble precipitate. The intensity of the blue color of the supernatant is inversely proportional to the amount of acidic EPS in the reaction mixture. Xanthan gum, the reference material needed to assay the acidic sugar quantitation in EPS, was dissolved in D-H2O to 0.5 mg/ml by stirring with mild heating for 30 min and then stored at 4℃. Using a standard curve constructed with different xanthan gum concentrations (50, 100, 150, 200 µg/ml), the Y-axis on the standard curve was calculated by subtracting the OD600 for each xanthan concentration from a blank. The quantity of acidic sugar in EPS was estimated from the standard curve. All analyses were performed in triplicate.
The freeze-dried EPS was resolved by SDS-polyacrylamide gel electrophoresis (29). EPS was dissolved in a buffer containing 2% SDS, 25% glycerol, 60 mM Tris-HCl (pH 6.8), 14.4 mM 2-mercaptoethanol, and 0.1% bromophenol blue to a concentration of 1% (w/v), and heated to 100℃ for 15 min. SDS-PAGE were carried out on 0.1% SDS-15% polyacrylamide gel (100×80×0.15 mm) in the electrophoresis buffer (pH 8.8) containing 25 mM Tris, 192 mM glycine, and 0.1% SDS. The details to visualize EPS on the gel by silver staining can be found in reference (30). The molecular weights were estimated using the Rf values of the EPS bands from a standard curve constructed with the Rf values (the relative mobility) of the protein size makers, which were plotted on a semi-logarithmic graph as a function of the protein molecular weights (31).
The sugar composition was analyzed by Bio-liquid chromatography at the Carbohydrate Bioproduct Research Center of Sejong University (Seoul, Korea). Twenty µg of the freeze-dried EPS was hydrolyzed at 100℃ for 4 hr with 2 M trifluoroacetic acid in sealed test tubes. The EPS-acid hydrolysates were analyzed on a CarboPacTM PA1 column with a HPAEC-PAD system (Dionex, Sunnyvale, CA, USA). The column was eluted at a flow rate of 1 ml/min with 18 mM NaOH (for neutral and amino sugar detection) and 100 mM NaOH/150 mM NaOAC (for acidic sugar detection), respectively.
The RAW 264.7 cells were cultured in Dulbecco's modified Eagle's medium (Hyclone Laboratories Inc., Logan, Utah, USA) supplemented with 10% heat-inactivated fetal bovine serum (Hyclone) 100 U/ml penicillin and 100 µg/ml streptomycin (Hyclone), and 50 µM 2-mercaptoethanol (SIGMA, St. Louis, MO, USA) at 37℃ in an atmosphere containing 5% CO2.
The RAW cells were cultured in the presence of different EPS concentrations (48.32 µg glucose-equivalent EPS/mg EPS, 9.0 µg acidic sugar equivalent EPS/mg EPS) in a 96-well microtiter plate (2×104 cells/well). DNA synthesis was measured by the level of [3H]-thymidine (2 Ci/mmol, PerkinElmer, Shelton, CT, USA) incorporation at a concentration of 0.5 µCi/well for the final 6 hr of the 2 day culture period. The cells were harvested onto glass fiber filter paper using an automated cell harvester (Inotech, Dottikon, Switzerland). The filters were washed, dried and then counted in a microbeta liquid scintillation counter (Wallac, Turku, Finland).
The RAW cells were cultured in the presence of different EPS concentrations in a 24-well microtiter plate (5×105 cells/well) in a total volume of 1 ml. After 48 hr incubation, the culture supernatants were collected, and the quantities of IL-1β and TNF-α were measured using commercial immunoassay kits (BD Biosciences, San Diego, CA, USA) according to the manufacturer's instruction.
The RAW cells were cultured in the presence of different concentrations of EPS in a 24-well microtiter plate (5×105 cells/well) in a total volume of 1 ml. After 48 hr stimulation, 50 µl of the cell-free supernatants were collected, incubated with an equal volume of Griess reagent (1% sulfanilamide, 0.1% naphthylenediamine dihydrochloride, 5% H3PO4) at room temperature for 5 min, and the absorbance at 550 nm was determined using a VERSAmax (Molecular Devices Inc., CA, USA) microplate reader. The NO2- concentration was determined from the least squares linear regression analysis of a sodium nitrite standard curve.
The cells were stained with the monoclonal antibodies recognizing murine cell surface markers, as previously described (32). Phenotypic analysis of the macrophages was performed on a FACSCanto II (Becton-Dickinson, San Jose, CA, USA), and the data was analyzed using FlowJo software. The monoclonal antibodies, anti-CD40 (clone 3/23), anti-ICAM-1 (clone 3E2), anti-I-Ab (clone AF6-120.1), anti-B7-1 (clone 16-10A1), anti-B7-2 (clone GL1), and isotype-matched control antibodies were purchased from Pharmingen (San Diego, CA, USA). The dead cells were gated out by their low forward angle light scatter intensity. In most analyses, 10,000 cells were scored.
The RAW cells were cultured in 6-well plates (2×106 cells/well) in the presence or absence of EPS for 2 days, and then added with biodegradable microspheres (average diameter, 300 nm) containing both ovalbumin (OVA) and fluorescein isothiocyanate (FITC). The cells were incubated for 2 hr and washed with pre-warmed PBS to remove the unphagocytozed microspheres. The cells were then harvested by pipetting after cooling on ice for 20 min, fixed in 1% paraformaldehyde in PBS, and analyzed by flow cytometry on a FACSCanto II. Microspheres containing both OVA and FITC were prepared using a solvent-evaporation method (33). Briefly, OVA was dissolved in 3% polyvinyl alcohol (final, 4 mg/ml), and poly (l-lactic acid) (PLG, final, 5%), and FITC (final, 5 mg/ml) were dissolved in a mixture of acetone and ethanol (9:1). These two solutions, 150 ml of OVA solution and 30 ml of the PLG solution, were mixed slowly and emulsified by continuous stirring overnight at room temperature. The hardened microspheres were collected by centrifugation at 300×g, and washed twice with PBS.
The possible contaminants of endotoxin contained in the EPS were removed using Affi-Prep Polymyxin Matrix (BIO-RAD, Hercules, CA, USA). Briefly, 1 ml of Affi-Prep Polymyxin Matrix was packed in a Bio-spin column (BIO-RAD), centrifuged for 2 min at 200×g, and then 0.5 ml of the EPS (200 µg/ml) was added. After incubating overnight at 4℃, the effluent was recovered from the column by centrifugation under the same conditions.
The EPS obtained from the MK1 strain grown in a minimal glucose medium was observed by optical microscopy and SEM (Fig. 1). The freeze-dried EPS was a white powder and was readily soluble in water. The molecular weights of the purified EPS were rather heterogeneous, ranging from 10.6 to 55 kDa, when it was resolved by SDS-PAGE/silver staining (Fig. 2). Complete hydrolysis of the EPS with 2 M trifluoroacetic acid followed by monosaccharide composition analysis showed that this EPS was a heteropolysaccharide composed of glucose, rhamnose, mannose, galactose, glucosamine in an approximate molar ratio of 1.00:0.8:0.71:0.29:0.21 with a trace amount of fucose, fructose, and galacturonic acid, as shown in Fig. 3.
To examine the immnuomodulatory activity of EPS, EPS was added to the cultures of RAW cells, and the growth inhibitory activity was observed 2 days later. The growth inhibitory activity of the EPS on RAW cells was documented further by the [3H]-thymidine uptake for the final 6 hr of the 2 day culture period. As shown in Fig. 4, EPS inhibited the growth of RAW cells in a dose dependent manner. The growth inhibitory activity of EPS reached 90% at 100 µg/ml. EPS was treated with polymyxin B, which is a specific inhibitor of polysaccharide, to ensure that the effects of the EPS were not due to endotoxin contamination. Passage of the EPS solution (200 µg/ml) through the polymyxin B-affinity column did not reduce the growth inhibitory activity. The growth inhibitory activity of EPS was not due to direct cytotoxicity.
The increased expression of co-stimulatory molecules can be a marker of macrophage activation. Therefore, the effects of EPS on the expression of co-stimulatory molecules on RAW cells, which were involved in T cell activation, were also examined. As shown in Fig. 5, 100 µg/ml EPS increased slightly the expression of B7-1, B7-2 and ICAM-1. This demonstrates that EPS induces the further differentiation of macrophages.
Cytokine and nitric oxide production is a parameter of the functional activation of macrophages. To determine if the EPS-activated RAW cells produced cytokines and nitric oxide, the culture supernatants were collected at 48 hr and the quantity of TNF-α, IL-1β and nitric oxide were measured. The stimulatory activity of EPS on macrophages was demonstrated further by the dose-dependent increase in TNF-α production (Fig. 6A), IL-1β production (Fig. 6B) and nitric oxide release (Fig. 6C) by RAW cells stimulated with EPS. As shown in a representative result in Fig. 6, the incubation of EPS with a polymyxin B-affinity column did not reduce the macrophage-activating activity.
The phagocytic activity of the EPS-activated RAW cells was examined using the microspheres containing fluorescein isothiocyanate (FITC). One of the most distinguishing features of macrophage activation would be the increase in phagocytic activity. RAW cells were cultured in the presence of EPS (100 µg/ml) for 2 days, and added with the microspheres containing FITC. The cells were incubated for 2 hr and washed with pre-warmed PBS to remove the unphagocytozed microspheres. The cells were harvested, fixed and analyzed by flow cytometry. As shown in Fig. 7, the thin line histograms represent the phagocytic activity of RAW cells stimulated with EPS, and the shaded histograms represent the phagocytic activity of the untreated RAW cells. EPS activated the phagocytic activity. This demonstrates that EPS is an activator of macrophages. Passage of the EPS solution (200 µg/ml) with the polymyxin B-affinity column did not reduce the phagocytic activity of RAW cells.
These results show that the EPS produced by the MK1 strain isolated from Neungee mushroom is an immunomodulatory polysaccharide with no cytotoxic effects. EPS was shown to inhibit the proliferation of RAW cells, inducing morphological changes from slightly adherent monocytic cells to strongly adherent macrophages. Because macrophages are end stage cells that do not proliferate further, the growth inhibitory activity of EPS may be due to the induction of further differentiation of monocytic RAW cells. EPS was also shown to stimulate RAW cells to produce nitric oxide, which is the principal effecter molecule produced by macrophages for cytotoxic activity and can be used as a quantitative index of macrophage activation (34). EPS stimulated the RAW cells to produce TNF-α and IL-1β, which have often been implicated as key mediators produced from macrophages in response to bacterial LPS, infection and inflammatory stimuli (35,36).
Immature macrophages do not induce primary immune responses because they do not express the requisite class II MHC molecules and co-stimulatory molecules, nor do they express antigenic peptides as stable complexes with MHC molecules. EPS was shown to enhance the expression of co-stimulatory molecules, such as B7-1 and B7-2, and class II MHC molecules. One of the most important parameters of macrophage activation is the increased phagocytic activity. Phagocytosis is an innate immune response mechanism for the removal of foreign pathogens. EPS was shown to activate the phagocytic activity on macrophages. These results show that EPS is a good activator of macrophages.
Indeed, plant-derived polysaccharides have been implicated for immunostimulatory polysaccharides. For example, immunostimulatory polysaccharides have been isolated from Aloe vera (32,37), Angelica acutiloba (38), Coriolus versiclor (39), Schizophyllum commune (40), and Lentinus edodes (41). The immunostimulatory polysaccharides isolated from these plants or mushrooms appear to trigger the immune responses primarily by activating macrophages, even though the direct activation of B cells and other immune cells has been implicated. Composition analysis of the polysaccharide suggests that it is quite different from well known immunomodulatory polysaccharides, such as acemannan, lentinan and PSK (37,39,41). Possible contamination of endotoxin is always a concern for high molecular weight components because endotoxin is a strong activator of macrophages and is contaminated in many plant materials. In this study, the macrophage-activating activity of EPS was not due to endotoxin contamination, as shown by polymyxin B-treatment experiments. A particular component of microbial EPS seems to be engaged in specific bioactivity. For example, phosphate-containing EPS produced by Lactobacillus delbrueckii sp. bulgaricus induced macrophage activation (42). Mannose-rich EPS from Tremella mesenterica (43) or rhamnose-rich (6-deoxy-L-mannose) EPS from Lactobacillus rhamnosus (44) stimulated the immune system through the mannose receptors situated on macrophage. Fucosamine-containing EPS produced by a marine Pseudomonas exhibited strong antiviral activity (11). L-fucose-enriched EPS would be used as a skin moisturizing agent in the cosmetic industry because L-fucose exhibited anticancer and anti-inflammatory activity (45). Sulfate-containing EPS showed a broad range of important bioactivities, such as antioxidant, anticoagulant, and antithrombotic activities (46). Therefore, it is possible that macrophage activation by EPS would be associated with the rhamnose or mannose moieties in its structure.
Macrophages are the most important defense cells in the immune system in that they not only initiate immune responses, but can also serve as effecter cells. Furthermore, macrophages are unique components of the innate immunity and have a range of functions related to the activation process. Activated macrophages become more efficient antigen presenting cells because they express increased levels of class II MHC molecules and co-stimulatory molecules (47), altered phagocytic activity as well as increased cytokine and nitric oxide production (48). Because EPS exerts strong macrophage-activating activity and the MK1 strain produces copious amounts of EPS, these results may lead to the discovery of a new source of immunomodulatory polysaccharides that can be obtained in large quantities.
ACKNOWLEDGEMENTS
This work was supported by the grant of the Korean Ministry of Education, Science and Technology (The Regional Core Research Program/Chungbuk BIT Research-Oriented University Consortium).
References
1. Welman AD, Maddox IS. Exopolysaccharides from lactic acid bacteria: perspectives and challenges. Trends Biotechnol. 2003; 21:269–274. PMID: 12788547.


3. Wang H, Jiang X, Mu H, Liang X, Guan H. Structure and protective effect of exopolysaccharide from P. Agglomerans strain KFS-9 against UV radiation. Microbiol Res. 2007; 162:124–129. PMID: 16580187.


4. Sutherland I. Biofilm exopolysaccharides: a strong and sticky framework. Microbiology. 2001; 147:3–9. PMID: 11160795.


5. Kumar CG, Joo HS, Choi JW, Koo YM, Chang CS. Purification and characterization of an extracellular polysaccharide from haloalkalophilic Bacillus sp. I-450. Enzyme Microb Technol. 2004; 34:673–681.


6. Iyer A, Mody K, Jha B. Rheological properties of an exopolysaccharide produced by a marine Enterobacter cloaceae. Natl Acad Sci Lett. 2005; 28:119–123.
7. Lin CC, Casida LE. GELRITE as a gelling agent in media for the growth of thermophilic microorganisms. Appl Environ Microbiol. 1984; 47:427–429. PMID: 16346480.


8. Kalogiannis S, Iakovidou G, Liakopoulou-Kyriakides M, Kyriakidis DA, Skaracis GN. Optimization of xanthan gum production by Xanthomonas campestris grown in molasses. Process Biochem. 2003; 39:249–256.


9. Kong J, Lee H, Hong J, Kang Y, Kim J, Chang M, Bae S. Utilization of a cell-bound polysaccharide produced by the marine bacterium Zooglea sp: new biomaterial for metal adsorption and enzyme immobilization. J Mar Biotechnol. 1998; 6:99–103.
10. Okutani K. Antitumor and immunostimulant activities of polysaccharides produced by a marine bacterium of the genus Vibrio. Bull Jap Soc Sci Fish. 1984; 50:1035–1037.
11. Okutani K. Antiviral activities of sulfated derivatives of a fucosamine-containing polysaccharide of marine bacterial origin. Nippon Suisan Gakkaishi. 1992; 58:927–930.


12. Abbad Andaloussi S, Talbaoui H, Marczak R, Bonaly R. Isolation and characterization of exocellular polysaccharides produced by Bifidobacterium longum. Appl Microbiol Biotechnol. 1995; 43:995–1000. PMID: 8590666.


13. Roberts CM, Felt WF, Osman SF, Wijey C, O'Connor JV, Hoover DG. Exopolysaccharide production by Bifidobacterium longum BB-79. J Appl Bacteriol. 1995; 78:463–468.
14. Sreekumar O, Hosono A. The antimutagenic properties of a polysaccharide produced by Bifidobacterium longum and its cultured milk against some heterocyclic amines. Can J Microbiol. 1998; 44:1029–1036. PMID: 10029998.


15. Arena A, Maugeri TL, Pavone B, Iannello D, Gugliandolo C, Bisignano G. Antiviral and immunoregulatory effect of a novel exopolysaccharide from a marine thermotolerant Bacillus licheniformis. Int Immunopharmacol. 2006; 6:8–13. PMID: 16332508.


16. Kitazawa H, Yamaguchi T, Itoh T. B-cell mitogenic activity of slime products produced from slime-forming, encapsulated Lactococcus lactis ssp. cremoris. J Dairy Sci. 1992; 75:2946–2951. PMID: 1460126.


17. Kitazawa H, Yamaguchi T, Miura M, Saito T, Itoh T. B-cell mitogen produced by slime-forming, encapsulated Lactococcus lactis ssp. cremoris isolated from ropy sour milk, viili. J Dairy Sci. 1993; 76:1514–1519. PMID: 8326024.


18. Kitazawa H, Yamaguchi T, Fujimoto Y, Itoh T. Comparative activity of B-cell mitogen, a phosphopolysaccharide, produced by L. lactis ssp. cremoris on various lymphocytes. Anim Sci Technol. 1993; 64:605–607.
19. Kitazawa H, Yamaguchi T, Fujimoto Y, Itoh T. An analysis of mitogenic response of phosphopolysaccharide, a B-cell mitogen produced by Lactococcus lactis ssp. cremoris to spleen cells. Anim Sci Technol. 1993; 64:807–812.
20. Kitazawa H, Itoh T, Yamaguchi T. Induction of macrophage cytotoxicity by slime products produced by encapsulated Lactococcus lactis ssp. cremoris. Anim Sci Technol. 1991; 62:861–866.
21. Kitazawa H, Itoh T, Tomioka Y, Mizugaki M, Yamaguchi T. Induction of IFNγ and IL-1α production in macrophages stimulated with phosphopolysaccharide produced by Lactococcus lactis ssp. cremoris. Int J Food Microbiol. 1996; 31:99–106. PMID: 8880300.
22. Lee YN, Koo CD. Identification of bacteria isolated from diseased Neungee mushroom, Sarcodon aspratus. J Basic Microbiol. 2007; 47:31–39. PMID: 17304616.
23. Lee YN, Kim EJ. Characterization of a novel bacterium isolated from diseased Nuengee mushroom, Sarcodon aspratus. 2010. In : 11th Int Sym on the Genetics of Industrial Microorganisms; Melbourne, Australia. –Abs. p29.
24. Ryu JE, Lee YN. Optimizing culture condition of MK1 strain isolated from soft-rotten tissue of Neungee mushroom and its exopolysaccharide. Korean J Microbiol. 2009; 45:324–331.
25. McKellar RC, Geest JV, Cui W. Influence of culture and environmental conditions on the composition of exopolysaccharide produced by Agrobacterium radiobacter. Food Hydrocolloides. 2003; 17:429–437.


26. Dubois M, Gilles K, Hamilton JK, Rebers PA, Smith F. A colormetric method for the determination of sugars. Nature. 1951; 168:167. PMID: 14875032.
27. Masuko T, Minami A, Iwasaki N, Majima T, Nishimura SI, Lee YC. Carbohydrate analysis by a phenol-sulfuric and method in microplate format. Anal Biochem. 2005; 339:69–72. PMID: 15766712.
28. Ramus J. Alcian blue: A quantitative aqueous assay for algal and sulfated polysaccharides. J Phycol. 1977; 13:345–348.
29. Laemmli EK. Cleavage of structural proteins during the assembly of the head of bacteriophage T4. Nature. 1970; 227:680–685. PMID: 5432063.


30. Kim JB, Ahn JH. The modification of the silver stain method in sodium dodecyl sulfate-polyamide gels for detecting lipopolysaccharides. J Korean Soc Microbiol. 1993; 28:193–198.
31. Hedrick JL, Smith AJ. Size and charge isomer separation and estimation of molecular weights of proteins by disc gel electrophoresis. Arch Biochem Biophys. 1968; 126:155–164. PMID: 5671059.


32. Lee JK, Lee MK, Yun Y-P, Kim Y, Kim JS, Kim YS, Kim K, Han SS, Lee CK. Acemannan purified from Aloe vera induces phenotypic and functional maturation of immature dendritic cells. Int Immunopharmacol. 2001; 1:1275–1284. PMID: 11460308.


33. Gerelchuluun T, Lee YH, Lee YR, Im SA, Song S, Park JS, Han K, Kim K, Lee CK. Dendritic cells process antigens encapsulated in a biodegradable polymer, poly (D,L-lactide-co-glycolide), via an alternate class I MHC processing pathway. Arch Pharm Res. 2007; 30:1440–1446. PMID: 18087813.
34. Lorsbach RB, Murphy WJ, Lowenstein CJ, Snyder SH, Russell SW. Expression of the nitric oxide synthase gene in mouse macrophages activated for tumor cell killing. Molecular basis for the synergy between interferon-gamma and lipopolysaccharide. J Biol Chem. 1993; 268:1908–1913. PMID: 7678412.


35. Sherwin C, Fern R. Acute lipopolysaccharide-mediated injury in neonatal white matter glia: role of TNF-alpha, IL-1beta, and calcium. J Immunol. 2005; 175:155–161. PMID: 15972642.
36. Shamash S, Reichert F, Rotshenker S. The cytokine network of Wallerian degeneration: tumor necrosis factor-alpha, interleukin-1alpha, and interleukin-1beta. J Neurosci. 2002; 22:3052–3060. PMID: 11943808.
37. Im SA, Oh ST, Song S, Kim MR, Kim DS, Woo SS, Jo TH, Park YI, Lee CK. Identification of optimal molecular size of modified Aloe polysaccharides with maximum immunomodulatory activity. Int Immunopharmacol. 2005; 5:271–279. PMID: 15652758.


38. Yamada H, Kiyohara H, Cyong JC, Kojima Y, Kumazawa Y, Otsuka Y. Studies on polysaccharides from Angelica acutiloba. Part 1. Fractionation and biological properties of polysaccharides. Planta Med. 1984; 50:163–167.
39. Pang ZJ, Zhou M, Chen Y, Wan J. A protein-bound polysaccharide synergistic with lipopolysaccharide induces nitric oxide release and antioxidant enzyme activities in mouse peritoneal macrophages. Am J Chin Med. 1998; 26:133–141. PMID: 9799965.


40. Okamura K, Suzuki M, Yajima A, Chihara T, Fujiwara A, Fukuda T, Goto S, Ichinohe K, Jimi S, Kasamatsu T, Kawai N, Mizuguchi K, Mori S, Nakano H, Noda K, Sekiba K, Suzuki K, Suzuki T, Takahashi K, Takeuchi K, Takeuchi S, Ogawa N. Clinical evaluation of schizophyllan combined with irradiation in patients with cervical cancer. A randomized controlled study. Cancer. 1986; 58:865–872. PMID: 2941141.


41. Sasaki T, Takasuka N. Further study of the structure of lentinan, an anti-tumor polysaccharide from Lentinus edodes. Carbohydr Res. 1976; 47:99–104. PMID: 178444.


42. Kitazawa H, Harata T, Uemura J, Saito T, Kaneko T, Itoh T. Phosphate group requirement for mitogenic activation of lymphocytes by an extracellular phosphopolysaccharide from Lactobacillus delbrueckii subsp. bulgaricus. Int J Food Microbiol. 1998; 40:169–175. PMID: 9620124.
43. Chen NY, Hsu TH, Lin FY, Lai HH, Wu JY. Effects on cytokine-stimulating activities of EPS from Tremella mesenterica with various carbon sources. Food Chem. 2006; 99:92–97.


44. Chabot S, Yu HL, Leseleuc LD, Cloitier D, Van Calsteren MR, Lessard M, Roy D, Lacroix M, Oth D. Exopolysaccharides from Lactobacillus rhamnosus RW-9595M stimulate TNF, IL-6 and IL-12 in human and mouse cultured immunocompetent cells and IFN-γ in mouse splenocytes. Lait. 2001; 81:683–697.
45. Cescutti P, Kallioinen A, Impallomeni G, Toffanin R, Pollesello P, Leisola M, Eerikainen T. Structure of exopolysaccharide produced by Enterobacter amnigenus. Carbohydr Res. 2005; 340:439–447. PMID: 15680599.
46. Castro R, Piazzon MC, Zarra I, Leiro J, Noya M, Lamas J. Stimulation of turbot phagocytes by Ulva rigida C. Agardh polysaccharides. Aquaculture. 2006; 254:9–20.


47. Adams DO, Hamilton TA. The cell biology of macrophage activation. Annu Rev Immunol. 1984; 2:283–318. PMID: 6100475.


48. Fujiwara N, Kobayashi K. Macrophages in inflammation. Curr Drug Targets Inflamm Allergy. 2005; 4:281–286. PMID: 16101534.


Figure 1
Photographs of MK1 exopolysaccharide. (A) Colony morphology of MK1 on potato dextrose agar, (B) Purified EPS (freeze-dried powder), (C) optical microscopy view of EPS (40×), (D) SEM view (6,000×).
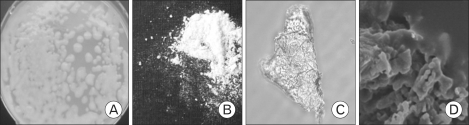
Figure 2
EPS resolved by SDS-PAGE. Resolution of the protein markers (5 µl) and EPS (150 µg) on 0.1% SDS-15% polyacrylamide gel.

Figure 3
Sugar profile of EPS by Bio-LC. Twenty µg of the freeze-dried EPS was hydrolyzed at 100℃ for 4 hr with 2 M trifluoroacetic acid in sealed test tubes. The EPS-acid hydrolysates were analyzed on a CarboPacTM PA1 column with a HPAEC-PAD system. (A) The profile of neutral and amino sugar. (B) Profile of acidic sugar.
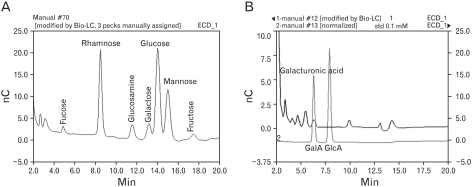
Figure 4
Growth inhibition of RAW cells stimulated with MK1. EPS was treated with a polymyxin B-affinity column to remove any possible endotoxin contamination. RAW cells were cultured in the presence of different MK1 EPS concentrations (0.8, 4, 20 and 100 µg/ml) for 2 days, and DNA synthesis was measured by [3H]-thymidine incorporation for the final 6 hr of the 2 day culture period. The CPM values of the untreated RAW cells served as control values in the calculation of % inhibition.

Figure 5
Phenotypic analysis of RAW cells stimulated with MK1 EPS. EPS was treated with a polymyxin B-affinity column to remove the possible contaminants of endotoxin. The RAW cells were cultured in the presence of EPS (100 µg/ml) for 2 days. The cells were collected, washed and used for immunophenotypic analysis. The levels of expression (thin line) are shown along with the isotype control (shaded line).
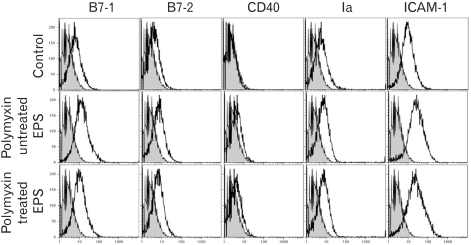
Figure 6
Cytokine and nitric oxide production of RAW cells stimulated with MK1 EPS. EPS was treated with a polymyxin B-affinity column to remove any possible endotoxin contamination. The RAW cells were cultured in the presence of different MK1 EPS concentrations (0.8, 4, 20 and 100 µg/ml) for 2 days, and the culture supernatants were assayed for TNF-α (A), IL-1β (B). The amounts of nitric oxide were measured using a Griess reagent (C).
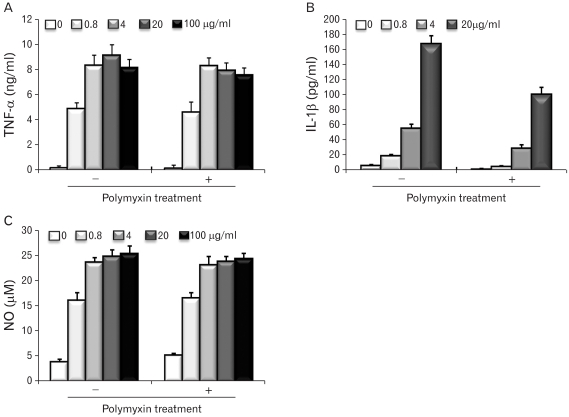
Figure 7
Phagocytic activity of RAW cells stimulated with MK1 EPS. EPS was treated with a polymyxin B-affinity column to remove any possible endotoxin contamination. The RAW cells were cultured in the presence of EPS (100 µg/ml) for 2 days, followed by the addition of microspheres containing fluorescein isothiocyanate (FITC). After 2 hr, unphagocytozed microspheres were removed by washing. The cells were harvested, fixed, and analyzed by flow cytometry. The thin line histograms represent the phagocytic activity of the RAW cells stimulated with MK1 EPS and the shaded histograms represent the phagocytic activity of the untreated RAW cells.
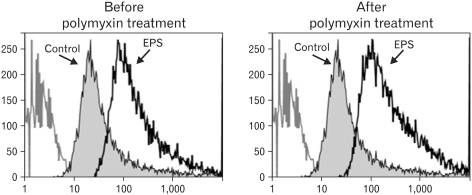