Abstract
Methods
Rat bone marrow mast cells (BMMC) were prepared both by recombinant rat IL-3 (rrIL-3) and by recombinant mouse stem cell factor (rmSCF), and investigated for both proliferation and differentiation in time course. Rat BMMC was induced by culture of rat bone marrow cells (BMCs) in the presence of both rrIL-3 (5 ng/ml) and rmSCF (5 ng/ml). Culture media were changed 2 times per week with the cell number condition of 5×104/ml in 6 well plate. Proliferation was analyzed by cell number and cell counting kit-8 (CCK-8) and differentiation was by rat mast cell protease (RMCP) II and histamine.
Results
Cell proliferation rates reached a maximum at 8 or 11 days of culture and decreased thereafter. However, both RMCP II production and histamine synthesis peaked after 11 days of culture. By real time RT-PCR, the level of histidine decarboxylase mRNA was more than 500 times higher on culture day 11 than on culture day 5. By transmission electron microscopy, the cells were heterogeneous in size and contained cytoplasmic granules. Using gated flow cytometry, we showed that cultured BMCs expressed high levels of FcεRI and the mast cell antigen, ganglioside, on culture day 11.
Mast cells derived from bone marrow are important immune cells that control innate and adaptive immunity (1-3). These cells are heterogeneous, depending on the tissue location, and have diverse functions, such as secretion of cytokine amines and prostaglandins as well as expression of cell membrane proteins that activate adjacent cells (4-6). In addition, these cells produce heparin, which is not produced by any other cells, and have a role in vasculogenesis (7,8).
The investigation of mast cell using mast cell line such as RBL-2H3, or HMC-1 has contributed the comprehension of cell activation and immune function of mast cells. However, for the application of cell therapy, the study of mast cell prepared by primary cell culture was necessary. Generating mast cells from direct differentiation of proliferating bone marrow cells (BMC) provides an ample supply of mast cells. Rat bone marrow-derived mast cells (BMMC) were first generated by Haig et al in 1982 using conditioned medium (CM) prepared from the cell culture supernatant of mesenteric lymph node cells. The lymph node cells were harvested from rats infected with Nipponstrongylus brasiliensis (9). In 1999, McCloskey et al described rat BMMC induction by both recombinant rat IL-3 (rrIL-3) and recombinant human stem cell factor (rhSCF) and contributed the comprehension of minimal required cytokines for rat BMMC culture (10). Since then, no study has characterized rat BMMCs generated with recombinant cytokines, nor have there been any reports on the immune function of BMMCs, as assessed by a pubmed search.
Mouse BMMCs induced by recombinant mouse IL-3 are widely used to study mast cell function (11,12). However, they are not appropriate for certain parasite infection models. The investigations using rat system produce the results contributing to comprehension of human infection in Trichinella spiralis, Clonorchis sinensis and Anisakis simplex (13-15). In these instances, the rat is a better model. The preparation of rat BMMC with recombinant cytokines was reported by the research group of McCloskey et al, using cell culture supernatant of COS cells transfected with necessary plasmid (10,16). However, their rat BMMC preparation was not known to be repeated by any other research laboratories. As the investigation of rat BMMC did not continue for 10 years, rat BMMC induction by recombinant cytokines was necessary for replication by any other investigator.
Mast cells have the characteristics to produce histamine. Although histamine activate diverse immune cells, that causes itch sensation or anaphylaxis in certain situation (17,18). In rat BMMC preparation, although mast cell maturity was analyzed by rat mast cell protease (RMCP) II, histamine synthesis is a good indicator to comprehend mast cell differentiation. Therefore, we have characterized rat BMMCs generated from recombinant cytokine induction of BMCs in relation with RMCP II level and histamine production. The described rat BMMCs are expected to enhance studies of mast cell immune function.
Seven-ten wk old female Sprague-Dawley outbred rats (150~200 g) were purchased from Samtako (Osan, South Korea). They were maintained in an experimental animal room and handled according to the guidelines of the Korean University Institutional Animal Care and Use Committee. All studies were performed with approval from the committee.
Both rrIL-3 and rmSCF were purchased from Biosource International-Invitrogen (Camarillo CA, USA) or from R&D Systems (Minneapolis MN, USA) (19,20). R rat IL-4 (rrIL-4) and r rat IL-6 (rrIL-6) were purchased from Biosource. LPS and Con A were purchased from Sigma (St. Louis MO, USA). BC4, mAb targeting rat high affinity IgE receptor (FcεRI), and AA4, mAb targeting rat mast cell antigen of ganglioside, were purchased from BD Biosciences (Jan Jose, CA, USA) (21,22). PE-labeled goat anti-mouse Ig-multiple adsorbed (PEGAMM) and PE-conjugated rat anti-mouse IgG1 mAb (PERAMG1) were also purchased from BD BioSciences. The RMCP II ELISA kit was purchased from Moredun Scientific limited (Midlothian, UK), and EIA HISTAMINE was purchased from Immunotech (Marseille, France) (23,24). The DC protein assay kit was purchased from Bio-Rad (Hercules, CA, USA). The prototype of rat IgE, IR162 was purchased from Zymed (San Francisco, CA, USA).
Rats were euthanized by anesthetization with CO2 and cervical dislocation. Bone marrow cells were harvested from the femur by syringe pumping and were separated from the red blood cells using a 50% or 70% Percoll gradient cushion (25,26). Cells were then washed with incomplete IMDM, supplemented with penicillin/streptomycin and gentamycin. Cells were plated at 5×104 cells/ml per well of a 6-well plate in complete IMDM supplemented with 20% inactivated horse serum, sodium pyruvate, 2-ME, non essential amino acids, L-glutamine, HEPES, and antibiotics. Each well was filled with 3 ml culture media that was changed 2 times per week. When necessary, cells were cultured for 4 days without changing the medium. Cell numbers were calculated using a hemocytometer.
Phenotypes were analyzed by flow cytometry using FACSCalibur (BD Biosciences, Jan Jose, CA, USA). Cell size was gated by FSC and SSC. Suspending cells were harvested from culture plate and washed with PBS by centrifugation. Cell suspensions were incubated with BC4, or AA4 mAb for 30 minutes on ice. Cells were washed twice with ice cold PBS and were incubated with PEGAMM or PERAMG1 for 1 hour. Cells were washed 3 times and then analyzed by flow cytometry.
RMCP II production or histamine synthesis was used as an indicator for rat mast cell differentiation. RMCP II and histamine levels were analyzed using the RMCP II ELISA kit (Moredun Scientific limited Midlothian, UK) (23) or the EIA HISTAMINE (Immunotech, Marseille, France) (24), respectively, according to the manufacturer's instruction. For the cells cultured in 6 well plate, cell lysate prepared with Triton X-100 were applied. For the cells cultured in 96 well plate, cell and cell supernatant were applied.
Cell proliferation was analyzed using the Cell counting kit-8 (CCK-8, Dojindo Laboratories, Kumamoto, Japan). Harvested cells were plated at 105 cells/well of a 96-well cell culture plate and were cultured at 37℃ in a humidified incubator with 5% CO2. Ten microliter CCK-8 diluted by ten times with IMDM was added to each well. After 4 hrs, the OD was read at 450 nm using a Spectramax ELISA microplate reader (Molecular Devices, Menlo Park, CA, USA). Cell numbers were calculated using a hemocytometer (purchased from Sigma) when the media was changed.
Cells were harvested at culture day 8 and incubated (cell No. 106/ml, 3 ml/well) in complete IMDM with IR162 (1µg/ml) (27) in the presence of 5 ng/ml rrIL-3 and 5 ng/ml rmSCF for 16 hrs at 37℃ in a CO2 incubator. Cells were washed once with Tyrode's solution. The cell supernatant was aspirated without disturbing the cell pellet. Cells were resuspended in Tyrode's solution to 6.7×105 cells/ml and treated with 5µg/ml of the rat IgE-specific mAb, B5 (28). Each 4 ml polypropylene tube contained 200µl of cell suspension. β-hexosaminidase released from the mast cells was analyzed using p-nitropnenyl-N-acetyl-β-D-glucosaminide as a substrate (29,30).
RNA was extracted from harvested cells using Trizol (Gibco Division of Invitrogen, Carlsbad, CA, USA). cDNA was synthesized by incubating RNA at 25℃ for 10 min, at 48℃ for 30 min, and then at 95℃ for 5 min with the SUPERSCRIPT reverse transcriptase (Invitrogen, Carlsbad, CA, USA) in a PTC-100 thermal cycler (MJ Research, Inc. Watertown MA, USA). HDC real time PCR primers included the following: sense, 5'-GCA GCA AGG AAG AAC AAA ATC C-3'; antisense, 5'-CAA CAA GAC GAG CGT TCA GAG A-3'; probe, FAM-AAA GCG CAT GAG CCC AAT GCT GAT-TAMRA (31,32). These were purchased from Bioneer (Daedockgu, Daejeon South Korea). The primers and probe for rodent GAPDH (Rat-GAPDH) were purchased from Applied Biosystems (Carlsbad, CA, USA) (33). The following PCR parameters were used: an initial incubation at 50℃ 2 min and a denaturation for 10 min at 95℃, followed by 40 cycles of 15 seconds at 95℃ and 1 min at 60℃. Real time PCR was assayed using the ABI Prism 7000 (Applied Biosystems). HDC mRNA expression levels were normalized against rat GAPDH using a relative quantitation of gene expression 2-ΔΔCT (34).
Harvested cells were fixed in a mixture of 2% glutaraldehyde and 2%paragormaldehyde. Cells were treated with 1% OsO4 and processed for TEM by the Division of Particular instruments and materials of the Medical Science Research Center (MSRC) at the Korean University College of Medicine (KUMC) (35). The prepared samples were analyzed using a Hitachi H-7500 transmission electron microscope.
Rat bone marrow cells cultured in rrIL-3 and rmSCF were visualized by light microscopy. Day 5 cells (Fig. 1A) were diverse in size and had blast like structures in cell clusters. When stained by Toluidine blue, 4-day cells (Fig. 1B), 11-day cells (Fig. 1C) and 18-day cells (Fig. 1D) had pinkish cytoplasmic staining.
To understand the detailed structure of cultured cells, cells were assayed by TEM. Rat bone marrow cells that were harvested over a 70% Percoll cushion on day 0 were very diverse in size and intracellular structure. The outer cell membrane was relatively irregular (Fig. 2A). At day 4, round cells with large nuclei were relatively predominant. Cells with dense cytoplasm, as seen by round black spots, were seen intermittently. At day 11, the size of the nuclei was relatively smaller than that of day 4 cells. The amount of cytoplasm was greater at day 11 than that at day 4. At day 15, the cells with dense cytoplasm were rare, and open or empty cytoplasmic structures were observed.
Fig. 3 presents the flow cytometric analysis of cultured rat bone marrow cells. Cells were harvested at day 11. Population analysis by light scatter demonstrated two groups, which were gated as R1 and R2 (Fig. 3A). The constituents of R1 were negative for BC4 or AA4 staining (Fig. 3B, D). However, the most constituents of R2 were BC4 and AA4 positive as evidenced (Fig. 3C, E).
Rat bone marrow cells were cultured in a humidified CO2 incubator at 37℃ in the presence of several concentrations of rrIL-3 and rmSCF. Five nanograms per milliliter both of rrIL-3 and of rmSCF were selected as the concentration required to induce evident cell proliferation (data not shown). The effects of culture media, supplemental serum, and cell density were analyzed by comparing RPMI-1640 vs. IMDM, FBS vs horse serum (HS), and 50% Percoll vs. 70% Percoll, respectively. When cultured in IMDM supplemented with FBS, cell proliferation peaked on culture day 8, regardless of cell differentiation density (Fig. 4A, closed circle and close triangle). When cultured in IMDM supplemented with HS, cell proliferation peaked on culture day 11 (Fig. 4A, open circle and open triangle). When cells were harvested over a 50% Percoll cushion and supplemented with HS supplement, proliferation peaked on day 8, regardless of the culture media used (Fig. 4B, closed circle and closed triangle). In other conditions tested, proliferation peaked on day 11 (Fig. 4B, open circle and open triangle, Fig. 4C).
Exocytosis of prepared rat BMMC was induced by the rat IgE, IR162, and the anti rat IgE, B5. The level of exocytosis was approximately 20% of the total cell lysate (Fig. 5). The total cell lysate was prepared by lysing cells in 1% TritonX-100/Tyrode. The exocytosis of rat BMMC induced by rat IgE and anti rat IgE showed a dose relationship when multiple number of rat BMMC was applied (data not shown).
On the culture day 8, cells were cultured with a diverse combination of several cell activators, including rmIL-3, rrIL-4, rrIL-6, LPS, and ConA (Fig. 6, bottom table). As measured with the cell counting kit, CCK-8, cells cultured with rrIL-3 and rmSCF grew better than cells cultured with either rrIL-3 or rmSCF alone, regardless of the Percoll cushion used (Fig. 6A and C). The cells cultured with both rrIL-3 and rmSCF produced 2 times more RMCP II than the cells cultured in either rrIL-3 or rmSCF alone (Fig. 6B and D).
Histamine production was used as a measure of BMMC differentiation. HDC expression was analyzed by real time RT-PCR using 0.006, 0.06 and 0.6µg RNA. An evident dose relationship was found between 0.06 and 0.6µg RNA. On culture day 11 HDC mRNA levels were 500~800 times higher than on culture day 5 (Fig. 7). Fig. 7 showed representative data.
The proliferation of cultured bone marrow cells peaked at day 8 or day 11 (Fig. 4A~C). The proliferation kinetics was similar to those described by Haig et al who found proliferation peaked at culture day 6 or 8 (9,38,39). Although rat BMCs cultured in CM are reported to proliferate in response to COS-1 cell supernatant carrying recombinant rat IL-3 (19,40), rat BMCs do not proliferate in response to recombinant rat IL-3 alone (unpublished data). In contrast, the addition of rmSCF and rrIL-3 induced evident BM cell proliferation. Since anti rrSCF antibody inhibits proliferation of bone marrow initiated by CM (41,42), SCF is an essential factor for rat BM cell growth.
RMCP II levels were analyzed as a marker for rat mast cells (Fig. 4D~F). Differentiated rat BMMC had high RMCP II levels and thus share characteristics of mucosal mast cells (41). RMCP II levels were compared to RMCP I, which is usually found in connective tissue mast cells (43). RMCP II levels were elevated on culture day 11 in most lysates, indicating a transition to a mucosal type mast cell.
Histamine is produced mainly by mast cells and basophils. This amine imparts its biological activities by binding histamine receptors on the cell membrane of non-classical histamine binding sites, in addition to its itching effect observed in allergic reactions (17,32). As an indicator of mast cell maturation, histamine levels were analyzed (Fig. 4G~I). Histamine levels were specifically elevated in most cases at culture day 11. In addition, HDC mRNA levels were more than 500 times greater on day 11 than on day 5 or 8 (Fig. 7). Although cells were actively proliferating at day 8, neither histamine nor mRNA was observed at that point. Since histamine synthesis and HDC transcription was much higher on day 11 than on day 8, mast cell maturation was complete by culture day 11. Additionally, histamine production in cultured BMCs was likely associated with new HDC transcription and not with preformed HDC, as histamine levels increased concurrently with HDC transcription.
By light microscopy, the cells showed signs of blast formation, which is indicating of active proliferation (Fig. 1A). Upon Toluidine blue staining, the cells harvested days 4, 11 and 18 had a pink cytoplasm, which is typical of mast cells. Cells were observed by electron microscopy, which enhanced our comprehension of the differentiation process. Fig. 2A shows BM cells with diverse size and nuclear structure. However, at culture day 4, most cells were relatively homogenous with large nuclei and small black cytoplasmic granules (Fig. 2B). On day 11, the nuclei were smaller than on day 4 (Fig. 2C), while the cytoplasmic granules were bigger. On day 15, nuclear structure was not dominant (Fig. 2D). These trends are similar to those expected for mast cell differentiation observed in vivo (22).
Based on AA4 staining, mast cells make up only 2.4% of non-cultured rat BMCs. Additionally, the AA4-positive cells expressed FcεRI as recognized by the BC4 antibody (22). In this study, most of the cultured rat bone marrow cells stained positive for both AA4 and BC4 at culture day 11 (Fig. 3B~E). By flow cytometric forward and side scatter analysis, 2 groups were evident when plotted in the log scale (Fig. 3A). R1 was 87.7% and R2 was 6.1% of dots. However, the R1 was shown as a small pint in the linear scale (unpublished data). In addition, the R1 was much smaller on day 2 than on day 11 (data not shown). Given that mature mast cells contain cellular microgranules, the R1 gate may constitute material secreted by the mast cells, such as exosomes or small organelles.
Mast cells from human cord blood or mouse BM cells are affected by certain cytokine or by LPS (44-47). The effect of recombinant cytokines, LPS, or Con-A on proliferating rat bone marrow cells was observed in a CCK-8 test. Bone marrow cell proliferation was induced by rrIL-3 and rmSCF and needed a continuous supply of both cytokines for efficient cell growth. No other tested cytokine enhanced the proliferation rate (Fig. 6A, C). Mouse IL-3 did not enhance proliferation, even in the presence of mouse SCF. This result is consistent to findings by Cohen et al who reported that rat IL-3 is differs markedly from mouse IL-3 in amino acid structure (19). Additionally, neither rrIL-4 nor rrIL-6 induced differentiation of the rat mast cells, as assessed by RMCP II expression. Continuous endeavor was believed to enhance rat mast cell differentiation processing by cytokines.
Although described in the early 80's, generation of rat BMMCs was not studied or reported after 1999 (10). The rat BMMC prepared with resuspended cytokines should simplify the investigation of mast cell function. Mouse BMMCs are generated worldwide from the addition of resuspended cytokine (5,48,49). Haig et al showed that rat stem cell factor alone could induce rat BMC proliferation and differentiation into rat BMMC by 1994 (41), although this finding was not repeated thereafter. In addition, they recorded that the CM used at 1994 (CM-11994) did not induce any rat BMC proliferation. The effect of CM-1994 was contrasted with the results reported before 1994 by Haig et al (9,38-40). In contrast to the description by Haig et al, this investigation did observe that neither rmSCF nor rrIL-3 alone were not sufficient to induce any rat BMC proliferation with the applied method (data not shown). However, in considering that rmSCF alone did not induce any mouse BMC proliferation, the results of this investigation was regarded to be high probable (50). In addition, rrSCF was shown not to induce mouse BMC proliferation independently (51).
In the preparation of bone marrow derived mast cell, suspending cells are mainly attended in mice, rats and humans (9,11,37,46). Rat BMC growing in adherence to culture plate bottom can be observed in the culture only with rrIL-3, in which, any rapid proliferation is not observed. The investigation by McCloseky et al described the adhering rat BMC as rat BMMC in the culture with both rhSCF and rrIL-3 (10). The adhering cells are not appropriate to be compared with the suspending cells that are rapidly proliferating.
Rats are very similar to humans in that both IL-3 and SCF are basically required for mast cell differentiation from BM cells (52,53). Considering the similarity between rats and humans in the differentiation of mast cells, further studies of rat BMMC are regarded to accelerate human diseases related to mast cells.
The current study described the generation of rat BMMCs by the simple addition of resuspended rrIL-3 and rmSCF. Neither the processing of parasite infection and maintenance, nor rat IL-3 cDNA transfection is not inevitable processing for rat BMMC investigation. In the kinetics of maturation of cultured mast cell, histamine synthesis was elevated abruptly on culture day 11, in contrast that RMCP II was progressively increased (Fig. 4). When cell phenotype was analyzed, cells on culture day 8 expressed both FcεRI and ganglioside antigen for AA4 (unpublished data). In considering that HDC mRNA was also abruptly elevated on culture day 11 (Fig. 7), rat BMMCs on culture day 8 were regarded to have the characteristics of mucosal mast cells that did not carry any histamine gene of mRNA or preformed histamine.
This investigation showed that rat bone marrow cells proliferated and differentiated into bone marrow derived mast cell in the presence of rrIL-3 and rmSCF. The proliferative BM cells demonstrate characteristics of mast cells with high levels of histamine and RMCP II. In addition, these cells express FcεRI and are positive for AA4, which recognizes ganglioside.
Figures and Tables
Figure 1
Light microscopic view (A) and Toluidine blue staining (B~D) of rat bone marrow cells. Cultured rat bone marrow cells were harvested at (B) 4 days, (C) 11 days, or (D) 18 days and were stained by Toluidine blue.
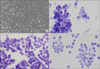
Figure 2
TEM of rat bone marrow cells. Rat bone marrow cells cultured in the presence of rrIL-3 and rmSCF were analyzed by TEM at day 0 (A), day 4 (B), day 11 (C), and day 15 (D).
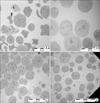
Figure 3
Flowcytometry analysis of cell populations and phenotype of cultured rat bone marrow cells. Cell were analyzed by flow cytometry for cell size by both FSC and SSC (A), and for surface expression of the BC4 (B, C) and AA4 (D, E) antigens. Control with dotted line in (B~E) were only treated with the secondary antibody, PE-goat anti mouse IgG. The solid lines in (B and C) were for the membrane protein for the primary antibody, BC4, and the 2nd antibody. The solid lines in (D and E) were for the membrane protein for the primary antibody, AA4, and the 2nd antibody. (B and D) for gated as the R1, (C and E) gated as the R2.
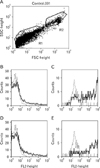
Figure 4
Cell proliferation, RMCP II production, and histamine synthesis in rat bone marrow cells treated with rrIL-3 and rmSCF. Rat BM cells were incubated under specified culture condition. Cells numbers were counted using a hemocytometer (A~C). RMCP II expression was analyzed with 200 ng/ml cell lysate of cultured rat bome marrow cells (D~F). Histamine levels were analyzed using a Histamine EIA kit (G~I). Culture conditions were controlled in in terms of serum (FBS vs. HS), media (IMDM vs. RPMI) or percoll concentration (50% vs. 70%). Open/white symbol or bar, FBS; and closed/gray symbol or bar, HS. In the line graph: circle, cells separated by 50% percoll; and triangle, cells by 70% percoll. Solid line, IMDM; and dash-dot line, RPMI-1640.
: 50 IM FBS,
: 50 IM HS,
: 50 RP HS,
: 50 RP HS,
: 70 IM FBS,
: 70 IM HS,
: 70 RP FBS,
: 70 RP HS. In the bar graph, bar with horizontal line, 50% percoll and IMDM; bar with oblique line, 50% percoll and RPMI-1640; bar with vertical line, 70% percoll and IMDM; bar with net cross, 70% percoll and RPMI-1640.
: 50 IM FBS,
: 50 IM HS,
: 50 RP FBS,
: 50 RP HS,
: 70 IM FBS,
: 70 IM HS,
: 70 RP FBS,
: 70 RP HS. IM for IMDM, RP for RPMI-1640, NA for not applied, ND for not determined. Y axis for both H and I in log scale.
















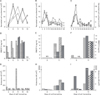
Figure 5
Exocytosis of cultured rat bone marrow cells by IR162 and B5. Cultled rat bone marrow cells were applied to the processing of exocytosis by IR162 and B5, a mouse anti rat IgE mAb.
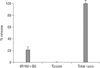
Figure 6
The effects of cytokine treatment on rat bone marrow cell proliferation and differentiation. The specified cytokines were added to activeted rat bone marrow cells at culture day 8, which were incubated for 3 (black bar) or 7 (white bar) days more. At the indicated time point, cell proliferation was analyzed CCK-8 (A and C) and cell differentiation was analyzed by RMCP II production (B and D). Cells harvested on a 70% Percoll cushion (A and B). Cells harvested on a 50% Percoll cushion. (C and D). rrIL-3, recombinant rat interleukin 3; rmSCF, recombinant rat stem cell factor; RMCP II, rat mast cell protease II; rrIL-4, recombinant rat interleukin-4; rrIL-6, recombinant rat interleukn-6; rmIL-3, recombinant mouse interleukin-3; LPS, lipopolysaccharide; Con A, concanavalin A. Cell number is represented as times of the given condition described in material and methods.
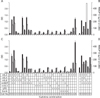
ACKNOWLEDGEMENTS
We appreciated Hyoung Kee Ahn (Department of Surgical Pathology Anam Hosptal, KUMC), Jin Chul Kim (Department of Parasitology, KUMC) and Chang Hyun Park (Medical Science Research Center, KUMC) for the assistance in slide preparation, bone marrow cell preparation and electron microscope operation. This work was supported both by the Korea Research Foundation Grant funded by the Korean Government (MOEHRD)(KRF-2005-015-E00099) and by the Brain Korea 21 Projects in 2008.
References
1. Galli SJ, Nakae S, Tsai M. Mast cells in the development of adaptive immune responses. Nat Immunol. 2005. 6:135–142.


3. Bonnefoy JY, Gauchat JF, Life P, Graber P, Mazzei G, Aubry JP. Pairs of surface molecules involved in human IgE regulation: CD23-CD21 and CD40-CD40L. Eur Respir J Suppl. 1996. 22:63s–66s.
4. Galli SJ, Grimbaldeston M, Tsai M. Immunomodulatory mast cells: negative, as well as positive, regulators of immunity. Nat Rev Immunol. 2008. 8:478–486.


5. McLachlan JB, Shelburne CP, Hart JP, Pizzo SV, Goyal R, Brooking-Dixon R, Staats HF, Abraham SN. Mast cell activators: a new class of highly effective vaccine adjuvants. Nat Med. 2008. 14:536–541.


6. Metz M, Maurer M. Mast cells -- key effector cells in immune responses. Trends Immunol. 2007. 28:234–241.


7. Humphries DE, Wong GW, Friend DS, Gurish MF, Qiu WT, Huang C, Sharpe AH, Stevens RL. Heparin is essential for the storage of specific granule proteases in mast cells. Nature. 1999. 400:769–772.


8. Soucek L, Lawlor ER, Soto D, Shchors K, Swigart LB, Evan GI. Mast cells are required for angiogenesis and macroscopic expansion of Myc-induced pancreatic islet tumors. Nat Med. 2007. 13:1211–1218.


9. Haig DM, McKee TA, Jarrett EE, Woodbury R, Miller HR. Generation of mucosal mast cells is stimulated in vitro by factors derived from T cells of helminth-infected rats. Nature. 1982. 300:188–190.


10. McCloskey MA, Fan Y, Luther S. Chemotaxis of rat mast cells toward adenine nucleotides. J Immunol. 1999. 163:970–977.
11. Razin E. Culture of bone marrow-derived mast cells: a model for studying oxidative metabolism of arachidonic acid and synthesis of other molecules derived from membrane phospholipids. Methods Enzymol. 1990. 187:514–520.
12. Nakano N, Nishiyama C, Kanada S, Niwa Y, Shimokawa N, Ushio H, Nishiyama M, Okumura K, Ogawa H. Involvement of mast cells in IL-12/23 p40 production is essential for survival from polymicrobial infections. Blood. 2007. 109:4846–4855.


13. Quan FS, Matsumoto T, Lee JB, Timothy O, Kim TS, Joo KH, Lee JS. Immunization with Trichinella spiralis Korean isolate larval excretory-secretory antigen induces protection and lymphocyte subset changes in rats. Immunol Invest. 2004. 33:15–26.


15. Cho SW, Lee HN. Immune reactions and allergy in experimental anisakiasis. Korean J Parasitol. 2006. 44:271–283.


16. McCloskey MA, Qian YX. Selective expression of potassium channels during mast cell differentiation. J Biol Chem. 1994. 269:14813–14819.


17. Dy M, Schneider E. Histamine-cytokine connection in immunity and hematopoiesis. Cytokine Growth Factor Rev. 2004. 15:393–410.


18. Jiang W, Kreis ME, Eastwood C, Kirkup AJ, Humphrey PP, Grundy D. 5-HT(3) and histamine H(1) receptors mediate afferent nerve sensitivity to intestinal anaphylaxis in rats. Gastroenterology. 2000. 119:1267–1275.


19. Cohen DR, Hapel AJ, Young IG. Cloning and expression of the rat interleukin-3 gene. Nucleic Acids Res. 1986. 14:3641–3658.


20. Zsebo KM, Wypych J, McNiece IK, Lu HS, Smith KA, Karkare SB, Sachdev RK, Yuschenkoff VN, Birkett NC, Williams LR, et al. Identification, purification, and biological characterization of hematopoietic stem cell factor from buffalo rat liver--conditioned medium. Cell. 1990. 63:195–201.


21. Basciano LK, Berenstein EH, Kmak L, Siraganian RP. Monoclonal antibodies that inhibit IgE binding. J Biol Chem. 1986. 261:11823–11831.


22. Jamur MC, Grodzki AC, Moreno AN, de Fatima CdM, Pastor MV, Berenstein EH, Siraganian RP, Oliver C. Identification and isolation of rat bone marrow-derived mast cells using the mast cell-specific monoclonal antibody AA4. J Histochem Cytochem. 2001. 49:219–228.


23. Ishih A, Uchikawa R. Immunoglobulin E and mast cell responses are related to worm biomass but not expulsion of Hymenolepis diminuta during low dose infection in rats. Parasite Immunol. 2000. 22:561–566.


24. Taurog JD, Mendoza GR, Hook WA, Siraganian RP, Metzger H. Noncytotoxic IgE-mediated release of histamine and serotonin from murine mastocytoma cells. J Immunol. 1977. 119:1757–1761.
25. Cho SW, Kilmon MA, Studer EJ, van der Putten H, Conrad DH. B cell activation and Ig, especially IgE, production is inhibited by high CD23 levels in vivo and in vitro. Cell Immunol. 1997. 180:36–46.


26. Defranco AL, Raveche ES, Asofsk R, Paul WE. Frequency of B lymphocytes responsive to anti-immunoglobulin. J Exp Med. 1982. 155:1523–1536.


27. Bazin H, Querinjean P, Beckers A, Heremans JF, Dessy F. Transplantable immunoglobulin-secreting tumours in rats. IV. Sixty-three IgE-secreting immunocytoma tumours. Immunology. 1974. 26:713–723.
28. Conrad DH, Studer E, Gervasoni J, Mohanakumar T. Properties of two monoclonal antibodies directed against the Fc and Fab' regions of rat IgE. Int Arch Allergy Immunol. 1983. 70:352–360.


29. Cho TH, Park HY, Cho S, Sohn J, Yoon YW, Cho JE, Cho SW. The time course of biological and immunochemical allergy states induced by Anisakis simplex larvae in rats. Clin Exp Immunol. 2006. 143:203–208.


30. Ortega Soto E, Pecht I. A monoclonal antibody that inhibits secretion from rat basophilic leukemia cells and binds to a novel membrane component. J Immunol. 1988. 141:4324–4332.
31. Watanabe T, Ohtsu H. L-histidine decarboxylase as a probe in studies on histamine. Chem Rec. 2002. 2:369–376.


32. Tippens AS, Davis SV, Hayes JR, Bryda EC, Green TL, Gruetter CA. Detection of histidine decarboxylase in rat aorta and cultured rat aortic smooth muscle cells. Inflamm Res. 2004. 53:390–395.


33. Kitamura Y, Das AK, Murata Y, Maeyama K, Dev S, Wakayama Y, Kalubi B, Takeda N, Fukui H. Dexamethasone suppresses histamine synthesis by repressing both transcription and activity of HDC in allergic rats. Allergol Int. 2006. 55:279–286.


34. Livak KJ, Schmittgen TD. Analysis of relative gene expression data using real-time quantitative PCR and the 2(-Delta Delta C(T)) Method. Methods. 2001. 25:402–408.


35. Kim SH, Cho YA, Park CH, Uhm CS. The ultrastructural changes of tendon axonal profiles of medial rectus muscles according to duration in patients with intermittent exotropia. Eye (Lond). 2008. 22:1076–1081.


36. Kulka M, Fukuishi N, Rottem M, Mekori YA, Metcalfe DD. Mast cells, which interact with Escherichia coli, up-regulate genes associated with innate immunity and become less responsive to Fc(epsilon)RI-mediated activation. J Leukoc Biol. 2006. 79:339–350.


37. Rottem M, Barbieri S, Kinet JP, Metcalfe DD. Kinetics of the appearance of FcεRI-bearing cells in interleukin-3-dependent mouse bone marrow cultures: correlation with histamine content and mast cell maturation. Blood. 1992. 79:972–980.


38. Haig DM, Jarrett EE, Tas J. In vitro studies on mast cell proliferation in N. brasiliensis infection. Immunology. 1984. 51:643–651.
39. Haig DM, McMenamin C, Gunneberg C, Woodbury R, Jarrett EE. Stimulation of mucosal mast cell growth in normal and nude rat bone marrow cultures. Proc Natl Acad Sci U S A. 1983. 80:4499–4503.


40. Haig DM, McMenamin C, Redmond J, Brown D, Young IG, Cohen SD, Hapel AJ. Rat IL-3 stimulates the growth of rat mucosal mast cells in culture. Immunology. 1988. 65:205–211.
41. Haig DM, Huntley JF, MacKellar A, Newlands GF, Inglis L, Sangha R, Cohen D, Hapel A, Galli SJ, Miller HR. Effects of stem cell factor (kit-ligand) and interleukin-3 on the growth and serine proteinase expression of rat bone-marrow-derived or serosal mast cells. Blood. 1994. 83:72–83.


42. MacDonald AJ, Thornton EM, Newlands GF, Galli SJ, Moqbel R, Miller HR. Rat bone marrow-derived mast cells co-cultured with 3T3 fibroblasts in the absence of T-cell derived cytokines require stem cell factor for their survival and maintain their mucosal mast cell-like phenotype. Immunology. 1996. 88:375–383.


43. Carlos D, Sá-Nunes A, de Paula L, Matias-Peres C, Jamur MC, Oliver C, Serra MF, Martins MA, Faccioli LH. Histamine modulates mast cell degranulation through an indirect mechanism in a model IgE-mediated reaction. Eur J Immunol. 2006. 36:1494–1503.


44. Yanagida M, Fukamachi H, Ohgami K, Kuwaki T, Ishii H, Uzumaki H, Amano K, Tokiwa T, Mitsui H, Saito H, Iikura Y, Ishizaka T, Nakahata T. Effects of T-helper 2-type cytokines, interleukin-3 (IL-3), IL-4, IL-5, and IL-6 on the survival of cultured human mast cells. Blood. 1995. 86:3705–3714.


45. Karimi K, Redegeld FA, Blom R, Nijkamp FP. Stem cell factor and interleukin-4 increase responsiveness of mast cells to substance P. Exp Hematol. 2000. 28:626–634.


46. Saito H, Ebisawa M, Tachimoto H, Shichijo M, Fukagawa K, Matsumoto K, Iikura Y, Awaji T, Tsujimoto G, Yanagida M, Uzumaki H, Takahashi G, Tsuji K, Nakahata T. Selective growth of human mast cells induced by Steel factor, IL-6, and prostaglandin E2 from cord blood mononuclear cells. J Immunol. 1996. 157:343–350.
47. Jayawardana ST, Ushio H, Niyonsaba F, Gondokaryono SP, Takenaka H, Ikeda S, Okumura K, Ogawa H. Monomeric IgE and lipopolysaccharide synergistically prevent mast-cell apoptosis. Biochem Biophys Res Commun. 2008. 365:137–142.


48. Razin E, Cordon-Cardo C, Good RA. Growth of a pure population of mouse mast cells in vitro with conditioned medium derived from concanavalin A-stimulated splenocytes. Proc Natl Acad Sci U S A. 1981. 78:2559–2561.


49. Vonakis BM, Gibbons SP Jr, Rotté MJ, Brothers EA, Kim SC, Chichester K, MacDonald SM. Regulation of rat basophilic leukemia-2H3 mast cell secretion by a constitutive Lyn kinase interaction with the high affinity IgE receptor (FcepsilonRI). J Immunol. 2005. 175:4543–4554.


50. Rottem M, Goff JP, Albert JP, Metcalfe DD. The effects of stem cell factor on the ultrastructure of Fc epsilon RI+ cells developing in IL-3-dependent murine bone marrow-derived cell cultures. J Immunol. 1993. 151:4950–4963.
51. Tsuji K, Zsebo KM, Ogawa M. Murine mast cell colony formation supported by IL-3, IL-4, and recombinant rat stem cell factor, ligand for c-kit. J Cell Physiol. 1991. 148:362–369.

