Abstract
Background
The underlying rationale of platelet rich plasma (PRP) therapy is that an injection of concentrated PRP at the site of injury may promote tissue repair via cytokine release from platelets. The molecular mechanisms of PRP therapy in the skin wound healing process are not well understood at present, and would benefit from clarification.
Methods
PRP was stimulated with angonists for 5 min, and cytokine profile analysis was performed. To investigate the wound healing activity of PRP, cell proliferation and migration analyses were performed in skin cells. The effects of PRP were analyzed on the expression and activity of matrix metalloproteinase (MMP)-1, -2, -9, and the activation of transcription factors.
Results
Thrombin was found to be a strong stimulator of PRP activation to release growth factors and chemokines. PRP induced cell proliferation and migration in HUVECs, HaCaT cells, and HDFs, as well as MMP-1and MMP-9 expression in HaCaT cells, but PRP did not have a significant effect on the expression or activity of MMPs in HDFs. The transcription factors, including signal transducer and activator of transcription-3 (STAT-3) were found to be phosphorylated following PRP treatment in HaCaT cells.
Conclusion
In this study, we have identified the cytokine profile of activated PRP after agonist stimulation. We have shown that PRP plays an active role in promoting the proliferation and migration of skin cells via the regulation of MMPs, and this may be applicable to the future development of PRP therapeutics to enhance skin wound healing.
The accumulation of platelets at sites of injury plays a critical role in hemostasis and atherothrombosis [1]. The adhesion of platelets to collagen exposed following the injury of endothelial cells initiates platelet activation and aggregation, and finally leads to the formation of a stable fibrin clot [2-4]. However, the function of platelet rich plasma (PRP) in the wound healing process has not yet been clearly elucidated.
Anuclear platelets contain several types of granules involved in coagulation, inflammation, atherosclerosis, antimicrobial host defense, and angiogenesis [5, 6]. Platelet-derived growth factor (PDGF), transforming growth factor-β (TGF-β), vascular endothelial growth factor (VEGF), basic fibroblast growth factor (bFGF), epidermal growth factor (EGF), and insulin-like growth factor (IGF) released from α-granules play a pivotal role in cell proliferation, migration, and differentiation. Adenosine diphosphate (ADP), serotonin, and calcium released from dense granules are key elements in the coagulation cascade and the recruitment of new platelets [7, 8]. Antiplatelet agents such as aspirin are targeted to block the formation of thromboxane A2 (TXA2), whilst the P2Y12 receptor antagonist clopidogrel, used in patients with coronary artery disease, inhibits ADP stimulation. ADP has an autocrine effect on promoting stable platelet aggregation by interacting with ADP-specific receptors (P2Y1 and P2Y12) on the platelet surface. ADP also has a paracrine effect by binding to ADP receptors on the surface of neighboring platelets, thereby amplifying platelet activation induced by other agonists. Platelets undoubtedly play a major role in pathogenic developments in the cardiovascular system. However, several lines of evidence suggest that platelets may also promote the wound healing processes.
PRP therapy is receiving growing attention in the fields of orthopedics, sports-related injuries, cancer biology, and dermatology. The underlying rationale of PRP therapy is that an injection of concentrated PRP at sites of injury may spur tissue repair via the release of growth factors from platelets. For successful wound healing, the turnover and remodeling of the extracellular matrix (ECM) must be exquisitely controlled, and the importance of biochemical mediators in tissue repair and homeostasis has been well demonstrated [9, 10]. In vivo and in vitro studies show that MMPs are frequently found in tissues undergoing repair or remodeling, and also in human atherosclerotic lesions [11-13]. Studies by Wang et al. and de Mos et al. have shown that PRP treatment increases cell proliferation, collagen production, and MMP expression in human tenocytes, which suggest that PRP might be a candidate as a future therapy for tendon regeneration [14, 15]. In human gingival fibroblasts, PRP stimulates membrane-type matrix metalloproteinase-1 (MT-MMP-1) and tissue inhibitor of metalloproteinase-2 (TIMP-2) production, cell migration/invasion, and myofibroblastic differentiation, and has positive effects in gingival repair [16]. In contrast, a recent study reported that PRP injections in chronic Achilles tendinopathy did not produce any significant improvement of symptoms [17]. Therefore, although some favorable results have been shown for PRP therapy in wound healing, the efficacy and underlying mechanisms of PRP are yet to be fully clarified.
In this study, we aimed to characterize PRP activation and test the feasibility of PRP therapy in skin wound healing.
Dulbecco's modified Eagle's medium (DMEM) and fetal bovine serum (FBS) were obtained from Hyclone (Logan, UT, USA). Endothelial cell growth medium-2 (EGM-2) Bulletkit was purchased from Lonza (Walkersville, MD, USA). MMP-1 antibody was bought from Calbiochem (San Diego, CA, USA). Anti-phospho-STAT3 (Tyr705), anti-phospho-c-Jun N-terminal kinase (JNK), and anti-phospho-nuclear factor kappa-light-chain-enhancer of activated B cells (NF-κB) antibodies were purchased from Cell Signaling Technology (Beverly, MA, USA), and anti-β actin antibody was obtained from Santa Cruz Biotechnology (Santa Cruz, CA, USA).
Ten healthy volunteers each donated 10 mL of whole blood. The donors gave informed consent for participation in the study. For the preparation of PRP, 10 mL of blood was collected in an acid citrate dextrose (ACD-A) tube coated with anti-coagulating agent (Sigma-Aldrich, 10% (v/v)) and centrifuged at 250×g for 15 min at 37℃. The supernatant fraction was harvested and incubated for 10 min at 37℃, then centrifuged at 1,900×g for 8 min. Pellets were resuspended in Tyrode's buffer (137 mM NaCl, 2.7 mM KCl, 16 mM NaHCO3, 5 mM MgCl2, 3.5 mM HEPES, 2.5 mM CaCl2, 5.5 mM glucose, 2 mg/mL BSA, pH 7.4) containing 10 U/mL heparin and 0.5 µM PGI2, and incubated for 10 min at 37℃. After centrifugation at 1,900×g for 8 min, Tyrode's buffer containing 0.02 U/mL of heparin was added to resuspend pellets and obtain PRP. Fluorescence-activated cell sorting (FACS) analysis was performed to stain P-selectin on platelets after stimulation with thrombin, and it was confirmed that 90% of the cell population expressed P-selectin. PRP containing 1.5×109 platelets/mL was prepared and used for further study. PRP stimulation was performed for 5 min with 5 µM ADP, 1% Ca2+, 20 µg/mL collagen, or 20 U/mL thrombin.
To analyze cytokines released from PRP, a multiplex MAP human cytokine/chemokine immunoassay (Millipore, Billerica, MA, USA) was used following the manufacturer's protocol (Luminex 100™ IS Total System, XMAP Technology with MasterPlex software, Millipore, Billerica, MA, USA). Forty growth factors, inflammatory cytokines, and chemokines, including PDGF-AA, AB/BB, TGF-α, VEGF, FGF, eotaxin, granulocyte-macrophage colony-stimulating factor (GM-CSF), interferon (IFN)-α2, IFN-γ, interleukin (IL)-10, IL-12, IL-1α, IL-1β, IL-1Rα, IL-2, IL-6, interferon gamma-induced protein 10 (IP-10), monocyte chemoattractant protein (MCP)-1, Regulated on activation normal T cell expressed and secreted (RANTES), and tumor necrosis factor-α (TNF-α), were quantitatively analyzed. For the analysis of TGF-β1, 2, and 3 isoforms, multiplex TGF-β1, 2, 3 immunoassay (Millipore, Billerica, MA, USA) was used following the manufacturer's protocol.
HUVECs were kindly gifted by Dr. Y.S. Choi (CHA University, Seoul, Korea) and cultured in endothelial cell growth medium (EGM) supplemented with growth factors, 10% FBS, and 1% penicillin/streptomycin solution (10,000 IU/mL). HaCaT cells (an immortalized keratinocyte cell line) were kindly gifted from Dr H.K. Lee (Yonsei University, Seoul, Korea), and HDFs were purchased from BioBud Co. (Seungnam, Korea). HaCaT cells and HDFs were cultured in DMEM (Sciencell, USA) containing 10% fetal bovine serum (FBS), 100 U/mL penicillin, and 100 µg/mL streptomycin (GIBCO) at 37℃, 5% CO2. HUVEC, and HDF cultures used for the study were passage 8 or less.
HUVECs were plated on a 1% gelatin-coated plate at a density of 40,000 cells/35-mm dish HaCaT cells were plated at a density of 50,000 cells/35-mm dish. The following day, cells were starved with serum-free medium for 24 hr. The culture medium was then replaced with fresh serum-free medium, and 200 µL of PRP was added (10% v/v). After incubation for 24 hr, cells were washed with PBS 3 times, trypsinized, and counted using a hemocytometer.
Cell migration was assessed by scratch wound assay [18]. Briefly, HaCaT cells were plated at a density of 80,000 cells/35-mm dish, and HDFs were plated at a density of 50,000 cells/35-mm dish. The following day, culture medium was replaced with fresh serum-free DMEM, and scratch wounds were created in a cell monolayer using a sterile pipette tip. After washing away suspended cells, the culture medium was replaced with fresh serum-free medium and 200 µL of PRP was added (10% v/v). After 24 hr, the wound width was estimated using a light microscope (Nikon, Japan) equipped with a digital camera, and 4 measurements of wound width were made at randomly chosen points. The extent of wound closure was presented as the percentage by which the original scratch width had decreased at each measured time point.
Two mL of conditioned media was concentrated to 500 µL by Vivaspin 500 (Satorius Stedim Biotech, Goettingen, Germany), and then 10 µL was loaded onto a 10% SDS-polyacrylamide gel containing 1 mg/mL gelatin. The gel was run in Tris/glycine buffer for 4 hr, and then incubated in 2.5% Triton X-100 solution for 15 min twice to remove SDS. To detect gelatinase activity, the gel was incubated in reaction buffer containing 50 mM Tris-HCl (pH 7.4), 10 mM CaCl2, and 0.05% Brij 35 overnight at 37℃. The gelatinolytic activity was shown by staining with 0.1% (w/v) Coomassie Brilliant Blue R-250, 10% (v/v) glacial acetic acid, and 30% (v/v) methanol, followed by destaining with 10% (v/v) acetic acid and 30% (v/v) methanol.
Proteins were extracted with urea lysis buffer (4 M urea, 0.5% SDS, 0.5% Nonidet P-40, 100 mM Tris (pH 7.4) and 5 mM EDTA). Cell lysates were centrifuged, and supernatants containing soluble proteins were collected and subjected to sodium dodecyl sulfate-polyacrylamide gel electrophoresis (SDS-PAGE). Separated proteins were then transferred onto 0.45-µm PVDF membranes. Blots were blocked with TBST (10 mM Tris-base, 100 mM NaCl, and 0.01% Tween 20) containing 5% bovine serum albumin for 1 hr, and then probed with corresponding antibodies. Membranes were incubated with HRP-coupled appropriate secondary antibodies for 1 hr, and immunoreactive signals were detected by using the ECL detection kit (GE healthcare).
For cell proliferation and cell migration assays, each experiment was repeated at least three times. The experimental data were analyzed by a one-way analysis of variance (ANOVA) or student t test. Statistical analysis was performed using Microsoft Exel Program. Data were presented as mean±SD, and P<0.05 was considered statistically significant.
PRP is considered to be a rich source of cytokines (growth factors/chemokines), thus, we characterized growth factor profiles after stimulation with agonists. One milliliter of resting PRP containing 1.5×109 platelets was stimulated with 5 µM ADP, 20 µg/mL collagen, 1% Ca2+, or 20 U/mL thrombin for 5 min. After centrifugation at 12,000×g for 30 min, supernatants were collected, and growth factor analysis was performed using a multiplex human cytokine/chemokine immunoassay system (Millipore Co, Billerica, MA, USA). Our study showed that thrombin acted as a powerful stimulator for the release of PDGF-AB/BB (>10,000 pg/mL), PDGF-AA (>10,000 pg/mL), and bFGF (370 pg/mL). However, the release of VEGF was negligible, and neither EGF nor IGF was detected following thrombin stimulation. When PRP was stimulated with ADP, collagen, or Ca2+, the levels of released PDGF-AA, AB/BB, and bFGF were relatively lower than in thrombin-stimulated PRP supernatants (Fig. 1A). This study indicates that the activation of 2 thrombin receptors, protease activated receptor type 1 (PAR-1) and PAR-4 on the platelet surface [19] occurred within 5 min. However, PRP activation was slower in response to ADP, Ca2+, and collagen, indicating differences in signaling dynamics between the platelet membrane receptors for these agonists. In an analysis of chemokine profiles, thrombin again acted as a strong stimulator for chemokine release by PRP. RANTES, GRO (cotton rat growth-regulated protein), and soluble CD40 ligand (sCD40L) were the 3 most abundantly expressed chemokines, with levels ranging between 2,000 pg/mL and 5,000 pg/mL. As expected, the highest level of RANTES release was observed by thrombin stimulation (3,228 pg/mL). This effect was not specific to thrombin, since unstimulated PRP, as well as PRP stimulated with ADP, Ca2+, or collagen, also released RANTES to concentrations ≥2,500 pg/mL. RANTES in α-granules of platelets is known to be released during the acute stages of inflammation and is a potent chemoattractant for T cells, monocytes, natural killer cells, basophils, and eosinophils [20]. It is notable that unstimulated PRP releases a high level of RANTES, as this indicates that the RANTES release from PRP is less dependent on agonist stimulation than other cytokines/chemokines. In contrast, the release of sCD40L (3,418 pg/mL) and GRO (3,895 pg/mL) from PRP was specific to thrombin stimulation (Fig. 1B). Platelets are a major source of sCD40L, and binding of sCD40L to CD40 receptor initiates inflammatory processes; thus, the pharmaceutical inhibition of sCD40L is being vigorously pursued as a potential avenue for the prevention of cardiovascular disease [21]. GRO, another chemokine stored in platelet α-granules, is a member of the CXC chemokine family, which also includes IL-4, platelet factor 4 (PF4), and macrophage inflammatory protein 2 (MIP-2). GRO is expressed in macrophages, endothelial cells, and fibroblasts, but the functions of GRO are not well understood at present, although increased serum levels of GRO were observed in women with preeclampsia [22]. In our analysis of TGF-β isoforms, thrombin was found to stimulate the release of TGF-β1 (2,435 pg/mL) and TGF-β2 (103.5 pg/mL). However, neither TGF-β3 nor TGF-α was released from PRP stimulated with thrombin, ADP, Ca2+, or collagen. PRP was mildly stimulated to release TGF-β1 (100 pg/mL) by ADP, Ca2+, and collagen (Fig. 1C).
The proliferation of skin cells is an active process in wound healing, in which coordinated upregulations are seen for PDGF, TGF-β, VEGF, bFGF, keratinocyte growth factor (KGF), and hepatocyte growth factor (HGF) [23]. On the basis of the results of our previous experiments, which showed a large release of growth factors and chemokines from both unstimulated and agonist-stimulated PRP, we further investigated the effects of PRP on cell proliferation. When HUVECs were treated with PRP for 24 hr, cell proliferation was increased 7-fold compared to serum-free controls (P<0.05, N=3). Surprisingly, there were no significant differences in cell proliferation rates between unstimulated PRP, and PRP stimulated with thrombin, ADP, or collagen. However, treatment of HUVECs with Ca2+ stimulated PRP significantly induced HUVEC proliferation. Ca2+ treatment without PRP treatment did not have any effect on HUVEC proliferation (data not shown). For HaCaT cells, we only tested the effect of unstimulated PRP on cell proliferation. The HaCaT cell proliferation rate was increased 5-fold by PRP compared to serum-free controls (P<0.05, N=4) (Fig. 2). This result is encouraging, because PRP itself may be safe to apply for therapeutics, while PRP agonists could cause adverse non-target effects. For example, treatment with bovine thrombin as a potent platelet activator can cause the development of antibodies against thrombin, which lead to severe postoperative bleeding following bypass graft surgery to treat thrombosis in animal studies [24].
As cell motility and migration play pivotal roles in skin wound healing, we investigated the effect of PRP on cell migration by scratch wound assay. When HaCaT cells and HDFs were treated with PRP for 24 hr, cell migration was increased by 60% in HaCaT cells (P<0.05, N=4) and 35% in HDFs (P<0.05, N=3) (Fig. 3). As PDGF-AA is a key chemoattractant in the process of tissue repair, we consider that PDGF-AA released from PRP may have played a role in inducing the migration of HDFs in this experiment [25]. However, an effect of PDGF-AA on the migration of HaCaT cells can be ruled out, since HaCaT cells do not express PDGF receptors on the cell surface [26]. Instead, TGF-β1 released from PRP is more likely to have been responsible for the migration of HaCaT cells via epithelial-to-mesenchymal transformation (EMT) [27].
Analysis of MMP expression has shown that MMP-1, -2, -3, -9, -10, and -14 are prevalently expressed during the skin wound healing process [28]. HaCaT cells were plated at a density of 300,000 cells/60-mm dish, and HDFs were plated at a density of 200,000 cells/60-mm dish in DMEM containing 10% FBS and 1% P/S. The following day, cells were starved with serum-free medium for 24 hr. Culture media were then replaced with fresh serum-free medium, and cells were incubated with 200 µL PRP (10% v/v) for 48 hr. Culture media were concentrated, MMP-2 and -9 were analyzed by gelatin zymography, and MMP-1 was analyzed by Western blotting. Our study showed that unstimulated PRP contained detectable levels of active MMP-9 and MMP-2. In HaCaT cells, treatment with PRP induced MMP-9 activity but did not have any effect on MMP-2 activity. In addition, MMP-1 expression was observed in unstimulated PRP, and treatment with PRP induced MMP-1 expression in HaCaT cells. However, PRP treatment did not have any significant effect on MMP-1, MMP-2, or MMP-9 activity in HDFs. Our study suggests that the effects of PRP on the regulation of MMP expression differ between HaCaT cells and HDFs (Fig. 4).
To investigate the regulation by PRP of transcription factors capable of transactivation at MMP promoters, we analyzed the activation of STAT-3, activator protein-1 (AP-1) and NF-κB by Western blotting using phospho-specific antibodies [29]. HaCaT cells were plated at a density of 300,000 cells/35-mm dish in DMEM containing 10% FBS and 1% P/S. The following day, cells were starved with serum-free medium for 48 hr. The culture medium was then replaced with fresh serum-free medium, and 200 µL PRP (10%, v/v) was added for 0, 5, 15, 30, 60, or 180 min. Following PRP incubations, cell lysates were prepared, and 40 µg protein was analyzed by Western blotting with anti-phospho-STAT-3, phospho-JNK and phospho-NF-κB antibodies. We found that PRP treatment induced the activation of STAT-3 by phosphorylation of tyr-705 from 5-30 min. A STAT-3 binding site is located in the promoter region of MMP-1 [29]. We found that PRP treatment induced the phosphorylation of JNK at 30 min, which may be responsible for MMP-1 and MMP-9 induction, as AP-1 binding sites are found in the promoter regions of MMP-1, -2, and -9. The activation of NF-κB by PRP was initiated at 5 min and sustained till 60 min. As NF-κB binding sites are also located in the promoter regions of MMP-1 and MMP-9, we assume that the activation of NF-κB may contribute to the induction of MMP-1 and MMP-9 expression (Fig. 5). Studies using specific inhibitors for these transcription factors may be able to further elucidate the mechanisms of MMP regulation by PRP treatment.
In this study, we hypothesized that the content of cytokines in PRP may have a favorable effect on skin wound healing, including cell proliferation, cell migration, and regulation of MMPs. Our result showed that thrombin was a powerful agonist for the induction of the release of growth factors and chemokines by PRP compared to ADP, Ca2+, or collagen. Although platelet α-granules are known to contain various growth factors, we found that the growth factors released from PRP stimulated with thrombin for 5 min were mostly PDGF-AB/PDGF-BB (>10,000 pg/mL), PDGF-AA (>10,000 pg/mL), TGF-β1 (2,435 pg/mL), and bFGF (370 pg/mL). However, only a negligible amount of VEGF was released. Surprisingly, stimulation with ADP, Ca2+, or collagen had only a very mild effect, or no effect on the release of growth factors from PRP (Fig. 1). We assume that 5 min duration for PRP stimulation with agonists may not be long enough to trigger α-degranulation, except for thrombin, which acts through PAR-1 and PAR-4 receptors on the platelet surface [19]. Accordingly, prior studies have reported that an almost complete release of growth factors occurs from PRP stimulated with thrombin within the first few hours, and thrombin leads to FGF degradation and decreased levels of FGF in chronic wounds [30-32]. In addition, Harrison et al. showed that the use of thrombin as an activator results in an immediate release of PDGF and TGF-β1, and the use of collagen as an activator results in a cumulative release of TGF-β1 from PRP, which suggests that intricate kinetics of growth factor release differ between agonists [33]. Thus, allowing sufficient time for agonists to access platelet surface receptors would be critical for platelet degranulation, and the kinetics of growth factor release should be considered in order to achieve maximum efficacy for PRP therapeutics.
In our chemokine analysis, we found that the levels of RANTES, sCD40L, and GRO released from PRP were highest after thrombin stimulation. Interestingly, the levels of RANTES were not significantly different between unstimulated PRP and agonist-stimulated PRP, and unstimulated PRP released high levels of RANTES (2,500 pg/mL). It has been suggested that the release of RANTES, CD40L, and GRO may be correlated. For example, in women with preeclampsia, levels of RANTES, sCD40L, and GRO were elevated together [22]. However, our study implies that the mechanisms of RANTES release from PRP are different from those of CD40L or GRO release. Furthermore, it is also suggested that circulating platelet microparticles (PMPs) serve as the transcellular delivery system for RANTES [34]. PMPs are membrane vesicles shed from platelets, which range from 0.1 to 1.0 µm in diameter. PMP shedding from platelets is known to be stimulated by thrombin or collagen [35]. Choi et al. reported that sCD40L release from platelets is mediated by the interaction of MMP-2 with glycoprotein IIIa (GPIIIa) [36]. These data are supported by the observation that sCD40L levels are effectively reduced by inhibitors of GPIIb/IIIa both in vitro and In vivo, while the GRO is also localized to GPIIIa [37, 38]. Taken together, it is highly possible that GRO and sCD40L may associate with GPIIIa and interact with each other during platelet activation.
PDGF and TGF-β1 actively participate in wound healing processes involving cell proliferation and migration. To investigate the effects of PRP on the skin wound healing process, we first tested its effect on HUVECs and HaCaT cells. When PRP stimulated with ADP, Ca2+, collagen, or thrombin was incubated with HUVECs, unstimulated PRP induced HUVEC proliferation 7-fold compared to serum-free controls. Surprisingly, thrombin-stimulated PRP did not show any significant effect on HUVEC proliferation; neither did ADP nor collagen-stimulated PRP (Fig. 2). The reasons for this may be as follows: based on our results, PRP stimulated with thrombin releases a large amount of cytokines within 5 min, and as platelets are anuclear, it is very likely that the released cytokines were already stored in platelet granules, as the de novo biosynthesis of cytokines could not occur without nuclei. This means that unstimulated PRP, or PRP stimulated with ADP or collagen, contained the same amount of stored cytokines as the PRP stimulated by thrombin, but the release of these stored cytokines from platelets was more highly stimulated by thrombin. Thus, during the 24 hr incubation period with PRP for cell proliferation experiments, physical interactions between cells and platelets may have triggered cytokine release from platelets, leading to a similar stimulation of cell proliferation. This is encouraging, because application of PRP at injury sites may cause the spontaneous activation of PRP, leading to the release of growth factors needed for cell proliferation in wound healing. This hypothesis is also supported by the increased cell migration induced by PRP in HaCaT cells (Fig. 3).
Kalvegren et al. showed that platelets induce the expression of MMP-1 and MMP-2, but not MMP-9 when analyzed by immunocytochemistry, and platelets release significant amounts of MMP-1 and MMP-2 when stimulated with collagen or thrombin [39]. According to Cecchetti et al., megakaryocytes contain transcripts of MMP-1, -2, and -9, but platelets do not contain MMP-2 transcripts [40]. Our study showed that unstimulated PRP contained active MMP-1, -2, and -9, and PRP treatment induced the expression of MMP-1 and MMP-9 by HaCaT cells. In contrast, treatment with PRP did not have any effect on MMP expression in HDFs (Fig. 4). Interestingly, Choi et al. showed that the inhibition of either platelet-derived MMP-2 activity or binding between MMP-2 and integrins, leads to significant reductions in platelet activation and the release of sCD40L by platelets [36]. These results imply that MMPs in PRP may have roles beyond extracellular matrix turnover, which may include interactions with growth factors or chemokines.
In summary, we have determined the cytokine profile of activated PRP after agonist stimulation. We have also shown that PRP has an active role in inducing proliferation and migration in skin cells, which suggests that PRP is a promising candidate as a therapeutic agent to promote and enhance skin wound healing (Fig. 6).
References
1. Libby P, Theroux P. Pathophysiology of coronary artery disease. Circulation. 2005; 111:3481–3488. PMID: 15983262.


3. Offermanns S. Activation of platelet function through G protein-coupled receptors. Circ Res. 2006; 99:1293–1304. PMID: 17158345.


4. Angiolillo DJ, Ueno M, Goto S. Basic principles of platelet biology and clinical implications. Circ J. 2010; 74:597–607. PMID: 20197627.


5. Blair P, Flaumenhaft R. Platelet alpha-granules: basic biology and clinical correlates. Blood Rev. 2009; 23:177–189. PMID: 19450911.
6. Badimon L, Vilahur G, Padró T. Lipoproteins, platelets and atherothrombosis. Rev Esp Cardiol. 2009; 62:1161–1178. PMID: 19793522.
7. Cattaneo M, Gachet C. ADP receptors and clinical bleeding disorders. Arterioscler Thromb Vasc Biol. 1999; 19:2281–2285. PMID: 10521355.


8. Jin J, Quinton TM, Zhang J, Rittenhouse SE, Kunapuli SP. Adenosine diphosphate (ADP)-induced thromboxane A(2) generation in human platelets requires coordinated signaling through integrin alpha(IIb)beta(3) and ADP receptors. Blood. 2002; 99:193–198. PMID: 11756171.
9. Toriseva M, Kähäri VM. Proteinases in cutaneous wound healing. Cell Mol Life Sci. 2009; 66:203–224. PMID: 18810321.


10. Ross R. The pathogenesis of atherosclerosis: a perspective for the 1990s. Nature. 1993; 362:801–809. PMID: 8479518.


11. Libby P, Sukhova G, Lee RT, Galis ZS. Cytokines regulate vascular functions related to stability of the atherosclerotic plaque. J Cardiovasc Pharmacol. 1995; 25(Suppl 2):S9–S12. PMID: 8699871.


12. Ganz P, Creager MA, Fang JC, et al. Pathogenic mechanisms of atherosclerosis: effect of lipid lowering on the biology of atherosclerosis. Am J Med. 1996; 101:4A10S–4A16S.
13. Lee E, Vaughan DE, Parikh SH, et al. Regulation of matrix metalloproteinases and plasminogen activator inhibitor-1 synthesis by plasminogen in cultured human vascular smooth muscle cells. Circ Res. 1996; 78:44–49. PMID: 8603504.


14. Wang X, Qiu Y, Triffitt J, Carr A, Xia Z, Sabokbar A. Proliferation and differentiation of human tenocytes in response to platelet rich plasma: An in vitro and In vivo study. J Orthop Res. 2011; [Epub ahead of print].


15. de Mos M, van der Windt AE, Jahr H, et al. Can platelet-rich plasma enhance tendon repair? A cell culture study. Am J Sports Med. 2008; 36:1171–1178. PMID: 18326832.
16. Caceres M, Martinez C, Martinez J, Smith PC. Effects of platelet-rich and -poor plasma on the reparative response of gingival fibroblasts. Clin Oral Implants Res. 2011; [Epub ahead of print].
17. de Vos RJ, Weir A, van Schie HT, et al. Platelet-rich plasma injection for chronic Achilles tendinopathy: a randomized controlled trial. JAMA. 2010; 303:144–149. PMID: 20068208.
18. Ranzato E, Patrone M, Pedrazzi M, Burlando B. Hmgb1 promotes wound healing of 3T3 mouse fibroblasts via RAGE-dependent ERK1/2 activation. Cell Biochem Biophys. 2010; 57:9–17. PMID: 20361273.


19. Lova P, Campus F, Lombardi R, et al. Contribution of protease-activated receptors 1 and 4 and glycoprotein Ib-IX-V in the G(i)-independent activation of platelet Rap1B by thrombin. J Biol Chem. 2004; 279:25299–25306. PMID: 15078882.


20. Gear AR, Camerini D. Platelet chemokines and chemokine receptors: linking hemostasis, inflammation, and host defense. Microcirculation. 2003; 10:335–350. PMID: 12851650.


21. Henn V, Steinbach S, Büchner K, Presek P, Kroczek RA. The inflammatory action of CD40 ligand (CD154) expressed on activated human platelets is temporally limited by coexpressed CD40. Blood. 2001; 98:1047–1054. PMID: 11493450.


22. Mellembakken JR, Solum NO, Ueland T, Videm V, Aukrust P. Increased concentrations of soluble CD40 ligand, RANTES and GRO-alpha in preeclampsia-possible role of platelet activation. Thromb Haemost. 2001; 86:1272–1276. PMID: 11816717.
23. Santoro MM, Gaudino G. Cellular and molecular facets of keratinocyte reepithelization during wound healing. Exp Cell Res. 2005; 304:274–286. PMID: 15707592.


24. Lawson JH. The clinical use and immunologic impact of thrombin in surgery. Semin Thromb Hemost. 2006; 32(Suppl 1):98–110. PMID: 16673271.


25. Schneider L, Cammer M, Lehman J, et al. Directional cell migration and chemotaxis in wound healing response to PDGF-AA are coordinated by the primary cilium in fibroblasts. Cell Physiol Biochem. 2010; 25:279–292. PMID: 20110689.


26. Lederle W, Stark HJ, Skobe M, Fusenig NE, Mueller MM. Platelet-derived growth factor-BB controls epithelial tumor phenotype by differential growth factor regulation in stromal cells. Am J Pathol. 2006; 169:1767–1783. PMID: 17071599.


27. Rahimi RA, Leof EB. TGF-beta signaling: a tale of two responses. J Cell Biochem. 2007; 102:593–608. PMID: 17729308.
28. Joo CK, Seomun Y. Matrix metalloproteinase (MMP) and TGF beta 1-stimulated cell migration in skin and cornea wound healing. Cell Adh Migr. 2008; 2:252–253. PMID: 19262153.
29. Overall CM, López-Otín C. Strategies for MMP inhibition in cancer: innovations for the post-trial era. Nat Rev Cancer. 2002; 2:657–672. PMID: 12209155.


30. Roussy Y, Bertrand Duchesne MP, Gagnon G. Activation of human platelet-rich plasmas: effect on growth factors release, cell division and in vivo bone formation. Clin Oral Implants Res. 2007; 18:639–648. PMID: 17590158.


31. Su CY, Kuo YP, Nieh HL, Tseng YH, Burnouf T. Quantitative assessment of the kinetics of growth factors release from platelet gel. Transfusion. 2008; 48:2414–2420. PMID: 18694465.


32. Sanchez M, Anitua E, Orive G, Mujika I, Andia I. Platelet-rich therapies in the treatment of orthopaedic sport injuries. Sports Med. 2009; 39:345–354. PMID: 19402740.
33. Harrison S, Vavken P, Kevy S, Jacobson M, Zurakowski D, Murray MM. Platelet activation by collagen provides sustained release of anabolic cytokines. Am J Sports Med. 2011; 39:729–734. PMID: 21398575.


34. Mause SF, von Hundelshausen P, Zernecke A, Koenen RR, Weber C. Platelet microparticles: a transcellular delivery system for RANTES promoting monocyte recruitment on endothelium. Arterioscler Thromb Vasc Biol. 2005; 25:1512–1518. PMID: 15890969.
35. VanWijk MJ, VanBavel E, Sturk A, Nieuwland R. Microparticles in cardiovascular diseases. Cardiovasc Res. 2003; 59:277–287. PMID: 12909311.


36. Choi WS, Jeon OH, Kim DS. CD40 ligand shedding is regulated by interaction between matrix metalloproteinase-2 and platelet integrin alpha(IIb)beta(3). J Thromb Haemost. 2010; 8:1364–1371. PMID: 20230421.
37. Slupsky JR, Kalbas M, Willuweit A, Henn V, Kroczek RA, Müller-Berghaus G. Activated platelets induce tissue factor expression on human umbilical vein endothelial cells by ligation of CD40. Thromb Haemost. 1998; 80:1008–1014. PMID: 9869175.


38. Simsek S, Folman C, van der Schoot CE, von dem Borne AE. The Arg633His substitution responsible for the private platelet antigen Gro(a) unravelled by SSCP analysis and direct sequencing. Br J Haematol. 1997; 97:330–335. PMID: 9163597.
39. Kalvegren H, Jönsson S, Jonasson L. Release of matrix metalloproteinases-1 and -2, but not -9, from activated platelets measured by enzyme-linked immunosorbent assay. Platelets. 2011; 22:572–578. PMID: 21756063.


40. Cecchetti L, Tolley ND, Michetti N, Bury L, Weyrich AS, Gresele P. Megakaryocytes differentially sort mRNAs for matrix metalloproteinases and their inhibitors into platelets: a mechanism for regulating synthetic events. Blood. 2011; 118:1903–1911. PMID: 21628401.


Fig. 1
Cytokine profiles released by PRP after agonist stimulation. One mL of resting PRP containing 1.5×109 platelets was stimulated with 5 µM ADP, 20 µg/mL collagen, 1% Ca2+, or 20 U/mL thrombin for 5 min. The released growth factors (A), chemokines (B), and TGF-βs (C) were measured using a multiplex human cytokine/chemokine immunoassay system.
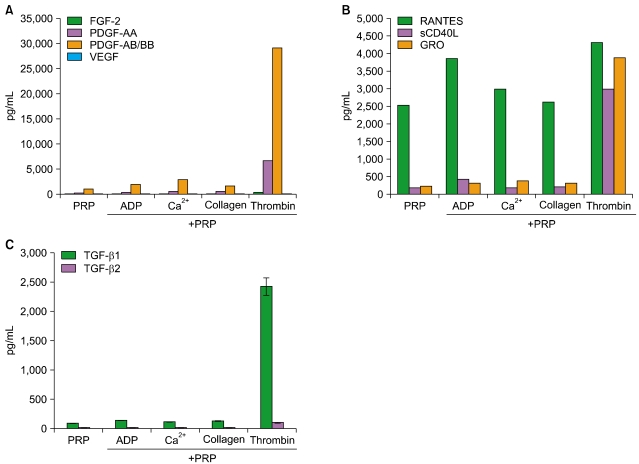
Fig. 2
Effects of PRP on cell proliferation. HUVECs (A) and HaCaT cells (B) were starved with serum-free medium for 24 hr, and then replaced with fresh serum-free medium and incubated for 24 hr with 200 µL of PRP (10% v/v), which had previously been stimulated with 5 µM ADP, 20 µg/mL collagen, 1% Ca2+, or 20 U/mL thrombin for 5 min. Cells were then harvested and counted by a hemocytometer. Each treatment was performed in triplicate, and data are presented as means±S.D.
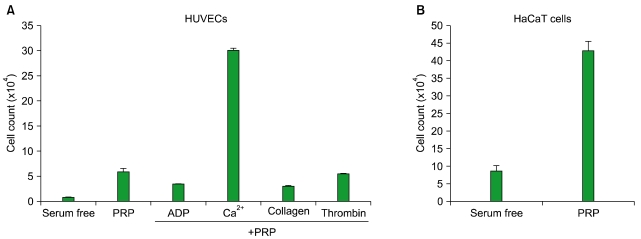
Fig. 3
Effects of PRP on the migration of skin cells. Scratch wounds were created in cell monolayers of HaCaT cells (A) and HDFs (B) using a sterile pipette tip. After washing away suspended cells, cells were replaced with fresh serum-free medium and 200 µL of PRP was added (10% v/v) for 24 hr. The extent of wound closure was presented as the percentage by which the original scratch width had decreased at each measured time point.
Experiments were performed in duplicate, and data are presented as means±S.D.
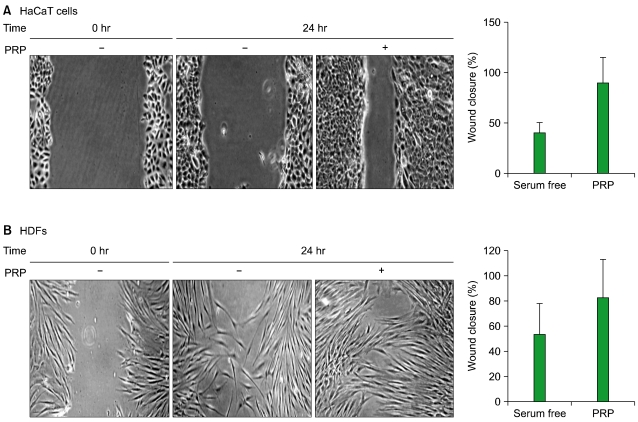
Fig. 4
Effects of PRP on the activity of MMP-1, 2, and 9 in skin cells. HaCaT cells (A) and HDFs (B) were starved with serum-free medium for 24 hr, then replaced with fresh serum-free medium and incubated with 200 µL of PRP (10:1, v/v) for 48 hr. The medium was concentrated, MMP-2 and MMP-9 activities were analyzed by gelatin zymography, and MMP-1 expression was analyzed by Western blotting.
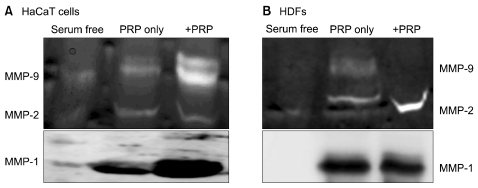
Fig. 5
Effects of PRP on the activation of STAT-3, JNK, and NF-κB in HaCaT cells. HaCaT cells were starved with serum-free medium for 48 hr, and fed with by medium replacement with fresh serum-free medium. Cells were then treated with 200 µL PRP (10% v/v) for 0, 5, 15, 30, 60, or 180 min. Cell lysates were analyzed by Western blotting with anti-phospho-STAT-3, phospho-JNK, and phospho-NF-κB antibodies.
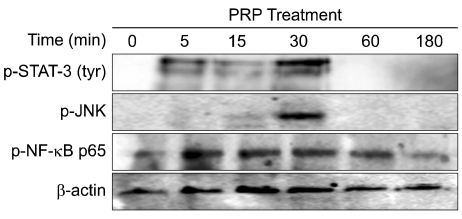