Abstract
Objective
This study examined the potential of the in vitro osteogenesis of microtopographically modified surfaces, RBM (resorbable blasting media) surfaces, which generate hydroxyapatite grit-blasting.
Methods
RBM surfaces were modified hydroxyapatite grit-blasting to produce microtopographically modified surfaces and the surface morphology, roughness or elements were examined. To investigate the potential of the in vitro osteogenesis, the osteoblastic cell adhesion, proliferation, and differentiation were examined using the human osteoblast-like cell line, MG-63 cells. Osteoblastic cell proliferation was examined as a function of time. In addition, osteoblastic cell differentiation was verified using four different methods of an ALP activity assay, a mineralization assay using alizarin red-s staining, and gene expression of osteoblastic differentiation marker using RT-PCR or ELISA.
Results
Osteoblastic cell adhesion, proliferation and ALP activity was elevated on the RBM surfaces compared to the machined group. The cells exhibited a high level of gene expression of the osteoblastic differentiation makers (osteonectin, type I collagen, Runx-2, osterix). imilar data was represented in the ELISA produced similar results in that the RBM surface increased the level of osteocalcin, osteopontin, TGF-beta1 and PGE2 secretion, which was known to stimulate the osteogenesis. Moreover, alizarin red-s staining revealed significantly more mineralized nodules on the RBM surfaces than the machined discs.
References
1. Davies JE. Mechanisms of endosseous integration. Int J Prosthodont. 1998; 11:391–401.
2. Berglundh T, Abrahamsson I, Lang NP, Lindhe J. De novo alveolar bone formation adjacent to endosseous implants. Clin Oral Implants Res. 2003; 14:251–62.


3. Albrektsson T, Wennerberg A. Oral implant surfaces: Part 2. Review focusing on clinical knowledge of different surfaces. Int J Prosthodont. 2004; 17:544–64.
4. Esposito M, Coulthard P, Thomsen P, Worthington HV. The role of implant surface modifications, shape and material on the success of osseointegrated dental implants: a Cochrane systematic review. Eur J Prosthodont Restor Dent. 2005; 13:15–31.
6. Zhao G, Schwartz Z, Wieland M, Rupp F, Geis-Gerstorfer J, Cochran DL, et al. High surface energy enhances cell response to titanium substrate microstructure. J Biomed Mater Res A. 2005; 74:49–58.


7. Cooper LF, Masuda T, Yliheikkila ¨ PK, Felton DA. Generalizations regarding the process and phenomenon of osseointegration: Part II. In vitro studies. Int J Oral Maxillofac Implants. 1998; 13:163–74.
9. Kieswetter K, Schwarts Z, Dean DD, Boyan BD. The role of implant surface characteristic in the healing of bone. Crit Rev Oral Biol Med. 1996; 7:329–45.
10. Thomas KA, Cook SD. An evaluation of variables influencing implant fixation by direct bone apposition. J Biomed Mater Res. 1985; 19:875–901.


11. Predecki P, Stephan JE, Auslaender BA, Mooney VL, Kirkland K. Kinetics of bone growth into cylindrical channels in aluminum oxide and titanium. J Biomed Mater Res. 1972; 6:375–400.


12. Carlsson L, Ro ¨stlund T, Albrektsson B, Albrektsson T. Removal torques for polished and rough titanium implants. Int J Oral Maxillofac Implants. 1988; 3:21–4.
13. Lauer G, Wiedmann-Al-Ahmad M, Otten JE, Hu ¨bner U, Schmelzeisen R, Schilli W. The titanium surface texture effects adherence and growth of human gingival keratinocytes and human maxillar osteoblast-like cells in vitro. Biomaterials. 2001; 22:2799–809.


14. Mustafa K, Wennerberg A, Wroblewski J, Hultenby K, Lopez BS, Arvidson K. Determining optimal surface roughness of TiO2 blasted titanium implant material for attachment, proliferation, and differentiation of cells derived from human mandibular alveolar bone. Clin Oral Implants Res. 2001; 12:515–25.
15. Ellingsen JE, Johansson CB, Wennerberg A, Holme ′ n A. Improved retention and bone-to implant contact with fluoride-modified titanium implants. Int J Oral Maxillofac Implants. 2004; 19:659–66.
16. Anselme K, Bigerelle M, Noel B, Dufresne E, Judas D, Iost A, et al. Qualitative and quantitative study of human osteoblast adhesion on materials with various surface roughnesses. J Biomed Mater Res. 2000; 49:155–66.


18. Wennerberg A, Albrektsson T, Andersson B. Bone tissue response to commercially pure titanium implants blasted with fine and coarse particles of aluminum oxide. Int J Oral Maxillofac Implants. 1996; 11:38–45.
19. Sader MS, Balduino A, Soares Gde A, Borojevic R. Effect of three distinct treatments of titanium surface on osteoblast attachment, proliferation, and differentiation. Clin Oral Implants Res. 2005; 16:667–75.


20. Le Guehennec L, Lopez-Heredia MA, Enkel B, Weiss P, Amouriq Y, Layrolle P. Osteoblastic cell behaviour on different titanium implant surfaces. Acta Biomater. 2008; 4:535–43.


21. Citeau A, Guicheux J, Vinatier C, Layrolle P, Nguyen TP, Pilet P, et al. In vitro biological effects of titanium rough surface obtained by calcium phosphate grit blasting. Biomaterials. 2005; 26:157–65.
22. Sanz A, Oyarzu ′ n A, Farias D, Diaz I. Experimental study of bone response to a new surface treatment of endosseous titanium implants. Implant Dent. 2001; 10:126–31.
23. Novaes AB Jr, Souza SL, de Oliveira PT, Souza AM. Histomorphometric analysis of the bone-implant contact obtained with 4 different implant surface treatments placed side by side in the dog mandible. Int J Oral Maxillofac Implants. 2002; 17:377–83.
24. Ginsberg SD, Che S. Combined histochemical staining, RNA amplification, regional, and single cell cDNA analysis within the hippocampus. Lab Invest. 2004; 84:952–62.


25. Hutton LC, Castillo-Melendes M, Smythe GA, Walker DW. Microglial activation, macrophage infiltration, and evidence of cell death in the fetal brain after uteroplacental administration of lipopolysaccharide in sheep in late gestation. Am J Obstet Gynecol. 2008; 198:e1–11.


27. Jones JV, Mansour M, James H, Sadi D, Carr RI. A substrate amplification system for enzyme-linked immunoassays: II. Demonstration of its applicability for measuring anti-DNA antibodies. J Immunol Methods. 1989; 118:79–84.
28. Harada M, Hiraoka BY, Fukasawa K, Fukasawa KM. Purification and properties of bovine dental-pulp alkaline-phosphatase. Arch Oral Biol. 1982; 27:69–74.


29. Jung K, Pergande M. Influence of inorganic phosphate on the activity determination of isoenzymes of alkaline phosphatase in various buffer systems. Clin Chim Acta. 1980; 102:215–9.


30. Valarmathi MT, Yost MJ, Goodwin RL, Potts JD. The influence of proepicardial cells on the osteogenic potential of marrow stromal cells in a three-dimensional tubular scaffold. Biomaterials. 2008; 29:2203–16.


31. Rausch-fan X, Qu Z, Wieland M, Matejka M, Schedle A. Differentiation and cytokine synthesis of human alveolar osteoblast compared to osteoblast-like cells (MG63) in response to titanium surfaces. Dent Mater. 2008; 24:102–10.
32. Gregory CA, Gunn WG, Peister A, Prockop DJ. An Alizarin red-based assay of mineralization by adherent cells in culture: comparison with cetylpyridinium chloride extraction. Anal Biochem. 2004; 329:77–84.


33. Malladi P, Xu Y, Chiou M, Giaccia AJ, Longaker MT. Effect of reduced oxygen tension on chondrogenesis and osteogenesis in adipose-derived mesenchymal cells. Am J Physiol Cell Physiol. 2006; 290:C1139–46.


34. Sudo H, Kodama HA, Amagai Y, Yamamoto S, Kasai S. In vitro differentiation and calcification in a new clonal osteogenic cell line derived from newborn mouse calvaria. J Cell Biol. 1983; 96:191–8.


35. Ba ¨chle M, Kohal RJ. A systematic review of the influence of different titanium surfaces on proliferation, differentiation and protein synthesis of osteoblast-like MG63 cells. Clin Oral Implants Res. 2004; 15:683–92.


36. Kim MJ, Choi MU, Kim CW. Activation of phospholipase D1 by surface roughness of titanium in MG63 osteoblast-like cell. Biomaterials. 2006; 27:5502–11.


37. Schwartz Z, Lohmann CH, Oefinger J, Bonewald LF, Dean DD, Boyan BD. Implant surface characteristics modulate differentiation behavior of cells in the osteoblastic lineage. Adv Dent Res. 1999; 13:38–48.


38. Anselme K, Bigerelle M. Topography effects of pure titanium substrates on human osteoblast longterm adhesion. Acta Biomater. 2005; 1:211–22.


39. Kim MJ, Kim CW, Lim YJ, Heo SJ. Microrough titanium surface affects biologic response in MG63 osteoblast-like cells. J Biomed Mater Res A. 2006; 79:1023–32.


40. Wennerberg A, Albrektsson T, Andersson B. Bone tissue response to commercially pure titanium implants blasted with fine and coarse particles of aluminum oxide. Int J Oral Maxillofac Implants. 1996; 11:38–45.
41. Deligianni DD, Katsala ND, Koutsoukos PG, Missirlis YF. Effect of surface roughness of hydroxyapatite on human bone marrow cell adhesion, proliferation, differentiation and detachment strength. Biomaterials. 2001; 22:87–96.


42. Diniz MG, Pinheiro MA, Andrade Junior AC, Fischer RG. Characterization of titanium surfaces for dental implants with inorganic contaminant. Braz Oral Res. 2005; 19:106–11.


43. Piattelli A, Degidi M, Paolantonio M, Mangano C, Scarano A. Residual aluminum oxide on the surface of titanium implants has no effect on osseointegration. Biomaterials. 2003; 24:4081–9.


44. Canabarro A, Diniz MG, Paciornik S, Carvalho L, Sampaio EM, Beloti MM, et al. High concentration of residual aluminum oxide on titanium surface inhibits extracellular matrix mineralization. J Biomed Mater Res A. 2008; 87A:588–97.


45. Rodrigo A, Valle ′ s G, Saldan ̃a L, Rodriguez M, Martinez ME, Munuera L, et al. Alumina particles influence the interactions of cocultured osteoblasts and macrophages. J Orthop Res. 2006; 24:46–54.


46. Wennerberg A, Albrektsson T, Johansson C, Andersson B. Experimental study of turned and grit-blasted screw-shaped implants with special emphasis on effects of blasting material and surface topography. Biomaterials. 1996; 17:15–22.


47. Li LH, Kong YM, Kim HW, Kim YW, Kim HE, Heo SJ, Koak YK. Improved biological performance of Ti implants due to surface modification by micro-arc oxidation. Biomaterials. 2004; 25:2867–75.


48. Giordano C, Sandrini E, Busini V, Chiesa R, Fumagalli G, Giavaresi G, et al. A new chemical etching process to improve endosseous implant osseointegration: in vitro evaluation on human osteoblast-like cells. Int J Artif Organs. 2006; 29:772–80.


49. Xavier SP, Carvalho PS, Beloti MM, Rosa AL. Response of rat bone marrow cells to commercially pure titanium submitted to different surface treatments. J Dent. 2003; 31:173–80.


50. Schneider GB, Perinpanayagam H, Clegg M, Zaharias R, Seabold D, Keller J, et al. Implant surface roughness affects osteoblast gene expression. J Dent Res. 2003; 82:372–6.
51. Schneider GB, Whitson SW, Cooper LF. Restricted and coordinated expression of beta3-integrin and bone sialoprotein during cultured osteoblast differentiation. Bone. 1999; 24:321–7.
Fig. 1.
Surface morphologhy of titanium discs for osteoblastic cell culture. SEM images of machined titanium (A) and RBM (B). Bar = 10 μm. EDA Data of machined titanium (C) and RBM (D). Surfaces were examined using the JSM-6480LV SEM at working distance of 10 mm and accelerating voltage of 20 kV.
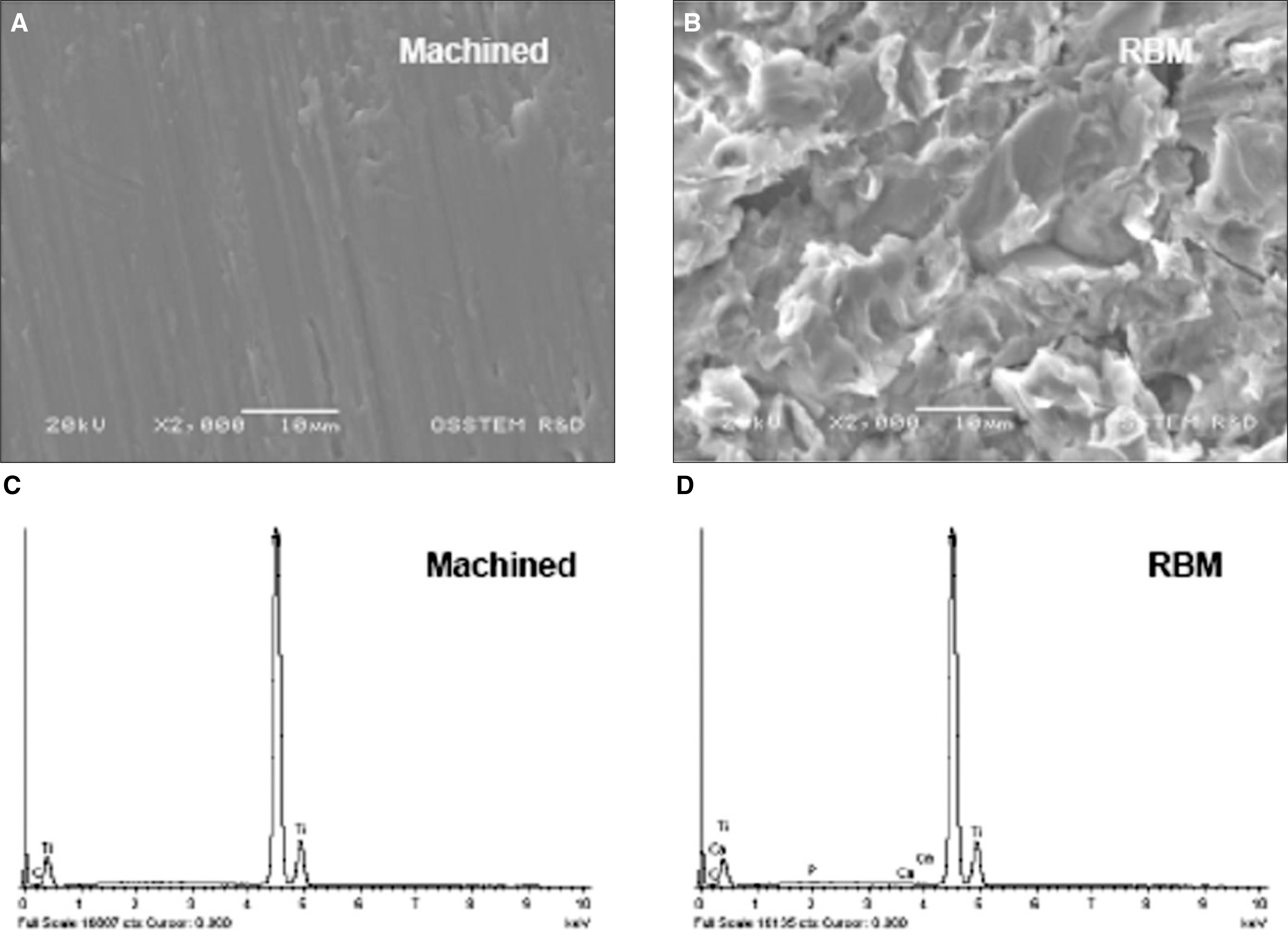
Fig. 2.
Effect of surface roughness on the adhesion of MG-63 cells. MG-63 cells were stained with cresyl violet dye and counted using spectrophotometer on surfaces of differing roughness. Data are represented as the average ± standard deviation. RBM surface, the rough surface, were promoted the cell adhesion compared to smooth machined surface. *: Statistically significant compared with cells cultured on machined surfaces.(P<0.05)
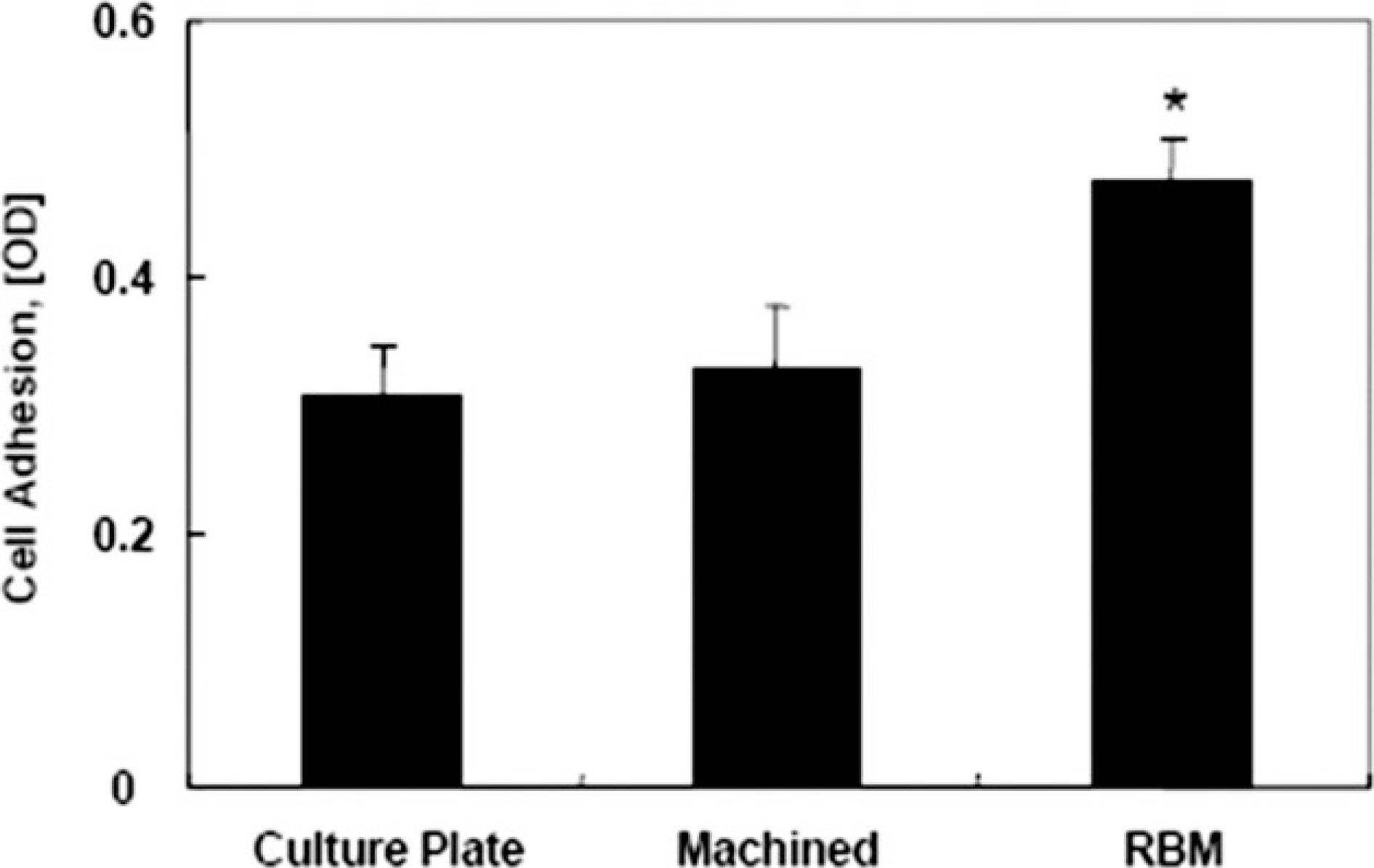
Fig. 3.
Results of MG-63 cell proliferation experiments. Data are represented as the average±standard deviation. The differences between the number of cells on RBM and machined surfaces during 5 days is statistically significant. *: Statistically significant compared with cells cultured on machined surfaces.(P<0.05)
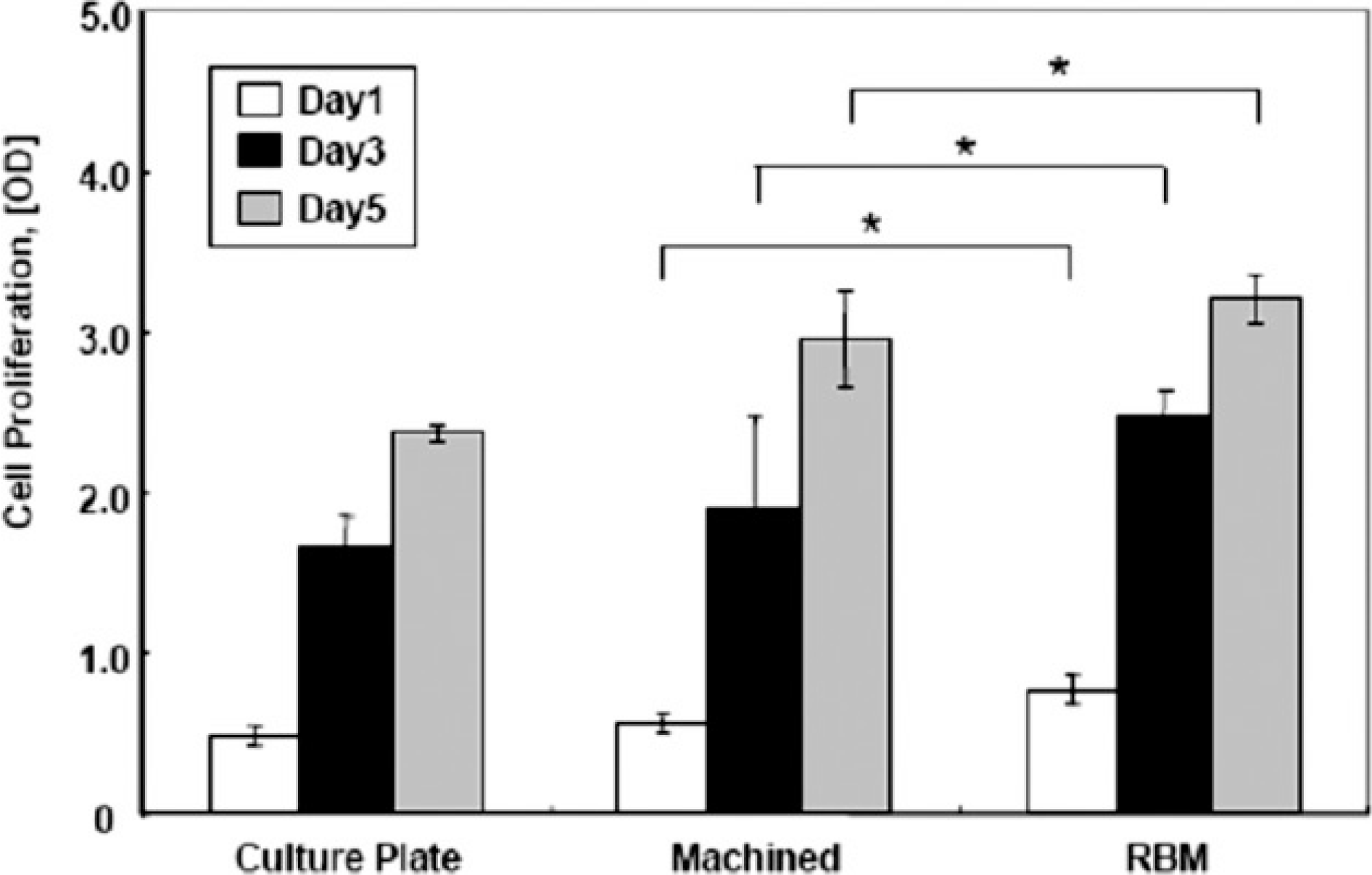
Fig. 4.
Effect of surface roughness on the osteoblastic differentiation of MG-63 cells. Data are represented as the average± standard deviation. (A) ALP (alkaline phosphatase) activity of MG-63 cells cultured either on machined Ti and RBM surfaces. Culture plate without osteogenic differentiation was served as a internal negative control. And a culture plate with osteogenic differentiation was a internal positive control. On RBM surface, the ALP activity enhanced about 10% than machined surface (B). Mineralization properties of the machined Ti and RBM surface. MG-63 cells were cultured as before for 14 days with osteogenic differentiation medium. Total calcium deposition measured by alizarin red-s stain via extraction with acetic acid. (C-F) Effect of surface roughness on osteogenesis-related local factor levels determined by using an ELISA kit. Osteocalcin levels were measure in the conditioned media using an ELISA kit specific for human osteoclacin (C). Osteopontin levels were measured in the conditioned media using an ELISA kit specific for human osteopontin (D). PGE2 levels were measured in the conditioned media using an ELISA kit specific for humans PGE2 (E). And Active of latent TGF-β1 (transforming growth factor-β1) levels were measure in the conditioned media using an ELISA kit specific for human TGF-β1 (F). (G) RT-PCR analysis of roughness-influenced osteogenic marker gene. Type I collagen, osteonectin, osterix and Runx-2 RNA levels in MG-63 cells exposure for 72hrs on a machined Ti or RBM surface. RT-PCR products were subjected to electrophoresis on 2% agarose gel and visualized UV exposure. A representative analysis is shown inside of the each figure. And the level of specific bands was normalized for the β-actin cDNA content. Statistically significant compared with cells cultured on machined surfaces by unpaired t-tests.(*P<0.01, **P<0.05, ***P<0.001)
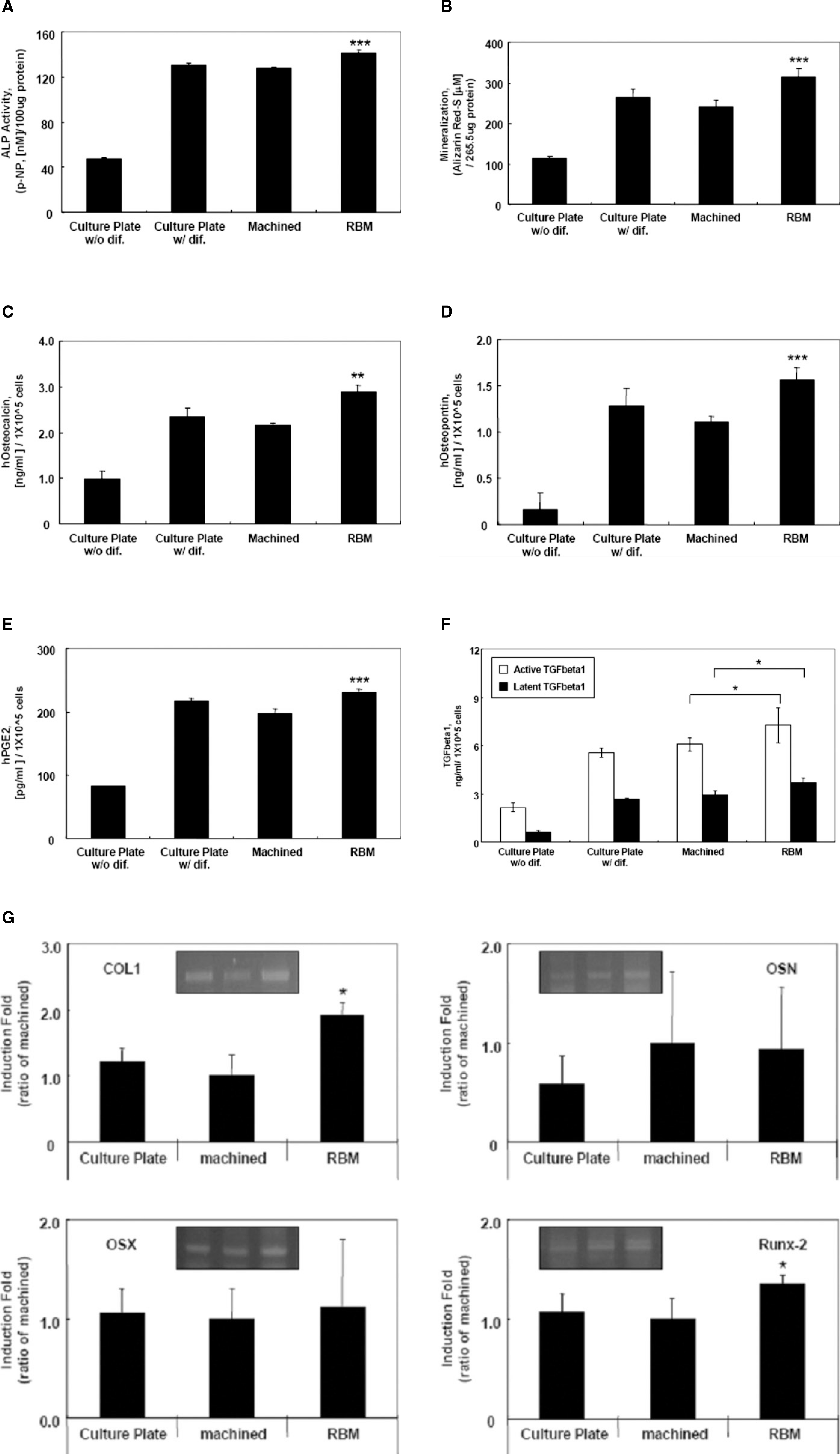
Table 1.
Rrimer sequences of osteogenic marker and conditions for polymerase chain reaction
Table 2.
Roughbess values of a machined Ti and a RBM surfaces measured by profilometer
Machied | RBM | |
---|---|---|
Ra, [um] | 0.222 (±0.039) | 1.523 (±0.156) |
Ra2max, [um] | 0.244 (±0.032) | 1.663 (±0.163) |
Rz, [um] | 1.566 (±0.199) | 10.514 (±1.177) |
Rt, [um] | 1.727 (±0.278) | 11.648 (±1.378) |