Abstract
Purpose
Parkinson's disease (PD) is a common age-dependent neurodegenerative disease. MiR-212 has been demonstrated to exert protective effects in several neurological disorders. The present study aimed to investigate the role and underlying molecular mechanism of miR-212 in PD.
Materials and Methods
1-methyl-4-phenylpyridinium (MPP+)-induced SH-SY5Y cells were applied as a PD model in vitro. RTqPCR was used to measure the expression of miR-212 and Kruppel-like factor 4 (KLF4) mRNA. Western blot analysis was performed to detect the protein levels of KLF4, Notch1 and Jagged1. Cell viability and apoptosis were determined by the Cell Counting Kit-8 and flow cytometry, respectively. Quantitative analysis of caspase-3 activity, lactate dehydrogenase (LDH), reactive oxygen species (ROS), superoxide dismutase (SOD), tumor necrosis factor-α (TNF-α), and interleukin-1 beta (IL-1β) was conducted with corresponding ELISA kits. Dual-luciferase reporter assay was employed to evaluate the relationship between miR-212 and KLF4.
Results
MiR-212 was downregulated in MPP+-induced SH-SY5Y cells. Also, miR-212 alleviated MPP+-induced SH-SY5Y cell damage, embodied by increased cell viability, decreased caspase-3 activity, LDH release, ROS production, TNF-α, and IL-1β expression, as well as elevated SOD levels. KLF4 was a direct target of miR-212, and miR-212 repressed KLF4 expression in a post-transcriptional manner. Moreover, miR-212-mediated protection effects were abated following KLF4 expression restoration in MPP+-induced SH-SY5Y cells, represented as lowered cell viability and enhanced apoptotic rate. Furthermore, Notch signaling was involved in the regulation of miR-212/KLF4 axis in MPP+-induced SH-SY5Y cells.
Parkinson's disease (PD) is an neurodegenerative disease characterized by the loss of dopaminergic neurons and muscular rigidity, affecting several million patients globally, especially older adults.1 Due to its complex causes and mechanisms, PD has attracted increasing attention from scientists and clinical physicians.2 Occurrence of inflammatory response, overproduction of oxidative stress, and activation of apoptotic cascade have been reported to be associated with the development of PD.3 Although many disease-modifying therapies for PD have been discovered and developed,4 it is still necessary to explore novel molecular mechanisms involved in PD for further breakthroughs in PD treatment.
MicroRNAs (miRNAs), a class of endogenous single-stranded noncoding transcript with 18−23 nucleotides (nt), play vital roles in a series of physiological and pathological processes.5 MiRNAs could regulate gene expression at the post-transcriptional level by targeting the 3′-untranslated region (3′-UTR) of messenger RNAs (mRNAs), leading to mRNA degradation or translational repression. Increasing evidence has described the participation of miRNA dysregulation in the pathogenesis of neurodegenerative disorders, including PD.67 For instance, Yang, et al.8 demonstrated that miR-22 overexpression promoted cell survival in 6-hydroxydopamine-induced PC12 cells via regulating transient receptor potential melastatin 7 (TRPM7) expression. MiR-590-3p suppressed mitochondrial dysfunction and oxidative stress through modulating its target gene JMJD1C.9 MiR-7 repressed 1-methyl-4-phenylpyridinium (MPP+)-triggered neuronal apoptosis by targeting Bax and sirtuin2 (Sirt2).10 MiR-212, necessary for the proper development, maturation, and function of neurons,11 has been implicated in a number of neurodegenerative and neurocognitive disorders, such as Alzheimer's disease, Huntington's disease, autism, Rett syndrome, and schizophrenia.12 Moreover, a previous study reported that miR-212 was downregulated in cerebrospinal fluid of PD patients.13 Nevertheless, little is known about the detailed function and molecular basis of miR-212 in PD progression.
In this study, we used MPP+-induced SH-SY5Y cells as an in vitro PD model to investigate the possible role and mechanism of miR-212. We found that miR-212 was downregulated in MPP+-induced SH-SY5Y cells. Moreover, miR-212 overexpression alleviated MPP+-induced damage in SH-SY5Y cells. Furthermore, the neuroprotective effect of miR-212 might be mediated by Kruppel-like factor 4 (KLF4)/Notch pathway in SH-SY5Y cells.
Human neuroblastoma SH-SY5Y cells were obtained from American Type Culture Collection (ATCC, Manassas, VA, USA). Cells were maintained in Dulbecco's modified Eagle's medium (DMEM, Gibco, Rockville, MD, USA) supplemented with 10% fetal bovine serum (Gibco) and 1% penicillin/streptomycin (Gibco) at 37℃ in a humidified incubator with 5% CO2.
MPP+ iodide was purchased from Sigma-Aldrich (St. Louis, MO, USA). To establish the PD model in vitro, SH-SY5Y cells were treated with various concentrations (0, 1, 2, and 4 mM) of MPP+ for 24 h or 2 mM of MPP+ for different treated times (0, 6, 12, and 24 h).
The KLF4-overexpression vector (pcDNA-KLF4) and pcDNA empty vector were obtained from Invitrogen (Waltham, MA, USA). MiR-212 mimics, miR-212 inhibitor (anti-miR-212), siRNA specifically against KLF4 (si-KLF4), and all control oligonucleotides (NC and si-NC) were synthesized by Sangon Biotech (Shanghai, China). All plasmids and oligonucleotides were transfected into SH-SY5Y cells with Lipofectamin™ 2000 transfection reagent (Invitrogen) referring to the manufacturer's instructions.
The Cell Counting Kit-8 (CCK-8, Dojindo Laboratories, Kumamoto, Japan) was applied to measure cell viability according to the manufacturer's instructions. Briefly, at indicated time points, CCK-8 solution (10 µL) was added into each cell culture medium for 2 h at 37℃, followed by the detection of absorbance at 450 nm with a microplate reader (Bio-Rad Laboratories, Hercules, CA, USA).
The apoptotic rate of SH-SY5Y cells was analyzed by the Annexin V apoptosis detection kit I (BD Biosciences, San Jose, CA, USA) and flow cytometry (BD Biosciences).
The Caspase-3 Human Instant ELISATM Kit (Invitrogen) was used to measure the caspase-3 activity according to the instructions. Briefly, cell lysates were incubated with 100 µM of enzyme-specific substrates at 37℃ for 4 h, followed by the measurement of absorbance at 450 nm with a microplate reader (Bio-Rad Laboratories). Additionally, lactate dehydrogenase (LDH) release, superoxide dismutase (SOD) level, reactive oxygen species (ROS) production, and tumor necrosis factor-α (TNF-α), and interleukin-1 beta (IL-1β) expression were measured using corresponding commercially available ELISA kits (Invitrogen), according to the provided instructions.
Online software algorithms (TargetScan, http://www.targetscan.org; MiRanda, http://www.microrna.org) were applied to search for the potential targets of miR-212. The 3′-UTR sequences of KLF4 containing the putative binding sites of miR-212 were amplified from human genomic DNA and then inserted into the pmirGLO Dual-Luciferase miRNA Target Expression Vector (Promega, Madison, WI, USA) to generate wild-type KLF4 reporter vector (KLF4-WT). Site-directed mutagenesis of the miR-212 binding sites in the KLF4 3′-UTR was carried out using GeneArtTM Site-Directed Mutagenesis System (Invitrogen), and then inserted into pmirGLO vector to generate mutant- type KLF4 reporter vector (KLF4-Mu). For reporter assays, SH-SY5Y cells were transfected with KLF4-WT or KLF4-Mu together with miR-212 mimics or anti-miR-212 using Lipofectamine™ 2000 (Invitrogen). The Dual-Luciferase Reporter Assay system (Promega) was used to measure the luciferase activity in cell lysates.
Total RNA was extracted from treated cells using TRIzol reagent (Invitrogen), and RNA (1 µg) was converted into cDNA using the cDNA Reverse Transcription Kit (Invitrogen) according to the manufacturer's instructions. qPCR was performed using HotStart-IT SYBR Green qPCR Master Mix (TOYOBO, Osaka, Japan) in the ABI PRISM® 7300 real-time PCR system (Applied Biosystems, Foster City, CA, USA). 2−ΔΔCt method was used to calculate the relative gene expression levels with GAPDH and U6 as internal controls. The primer sequences used for PCR amplification were as follows: miR-212, 5′-CCCTCTGG GACATCTTTGACG-3′ (forward) and 5′-TGCTCCGCC TCCCCTGCGTCTC-3′ (reverse); KLF4, 5′-CAAGTCCCGCCG CTCCATTACCAA-3′ (forward) and 5′-CCACAGCCGTCCC AGTCACAGTGG-3′ (reverse); Notch1, 5′-CTGGACCCCATG GACATC-3′ (forward) and 5′-AGGATGACTGCACACATT GC-3′ (reverse); Jagged1, 5′-GAATGGCAACAAAACTTGCAT-3′ (forward) and 5′-AGCCTTGTCGGCAAATAGC-3′ (reverse); GAPDH, 5′-AAGGTGAAGGTCGGAGTCAAC-3′ (forward) and 5′-GGGGTCATTGATGGCAACAATA-3′ (reverse); and U6, 5′-GCTTCGGCAGCACATATACTAAAAT-3′ (forward) and 5′-CGCTTCACGAATTTGCGTGTCAT-3′ (reverse).
Total proteins were extracted from treated cells with cell lysis buffer (RIPA, Beyotime, Shanghai, China). Then, proteins were subjected to 10% SDS-PAGE and transferred to PVDF membranes (Sigma-Aldrich). The PVDF membranes were blocked in TBST buffer (TBS supplemented with 0.1% Tween-20) containing 5% (wt/vol) skimmed milk powder for 2 h at room temperature. Followed by three washes in TBST, the PVDF membranes were then incubated with primary antibodies, including anti-β-actin (Santa Cruz Biotechnology, Santa Cruz, CA, USA), anti-KLF4 (Santa Cruz Biotechnology), anti-Notch1 (Santa Cruz Biotechnology), and anti-Jagged1 (Santa Cruz Biotechnology) respectively overnight at 4℃. After being washed three times in TBST for 10 min, the PVDF membranes were further probed with HRP-conjugated secondary antibodies (Abcam, Cambridge, UK). Lastly, the membranes were exposed to Hyperfilm-ECL (Thermo Fisher Scientific, Waltham, MA, USA) for 5 min, and visualized using a Fluor S Multimager and Quantity One 4.1 (Bio-Rad Laboratories).
In order to explore the effect of miR-212 on PD, RT-qPCR was first performed to detect the expression pattern of miR-212 in MPP+-induced SH-SY5Y cells. The results revealed that, compared with the control group, miR-212 expression was markedly lower in SH-SY5Y cells following MPP+ treatment (Fig. 1A). Then, miR-212 mimics and anti-miR-212 were synthesized and transfected into SH-SY5Y cells to examine their efficiency. As displayed in Fig. 1B, the expression level of miR-212 was significantly promoted by introduction with miR-212 mimics, while miR-212 expression was greatly inhibited after transfection with anti-miR-212 in SH-SY5Y cells, compared with the negative control. Subsequently, we further observed the effect of miR-212 on viability by gain- and loss-of-function analysis in MPP+-treated SH-SY5Y cells. SH-SY5Y cells were transfected with miR-212 mimics or anti-miR-212, followed by treatment with various concentrations of MPP+ (0−4 mM) for 24 h or with 2 mM for different times (0−24 h). As expected, MPP+ treatment led to an obvious viability suppression of SH-SY5Y cells in a dose- or time-dependent manner (Fig. 1C-F). However, MPP+-induced vitality diminishment at 2 mM and 4 mM for 24 h (Fig. 1C) or 2 mM for 12 h and 24 h (Fig. 1D) was markedly ameliorated by the restoration of miR-212 expression. Conversely, miR-212 downregulation aggravated MPP+-induced viability suppression in SH-SY5Y cells, compared with corresponding control (Fig. 1E and F). All these results implied that miR-212 weakened MPP+-induced decreases in the viability of SH-SY5Y cells.
Oxidative stress, activation of apoptotic cascade, and neuroinflammation have been confirmed to play central roles in the pathogenesis of PD.14 Hence, to further investigate the potential effect of miR-212 on MPP+-induced SH-SY5Y cells injury, SH-SY5Y cells transfected with miR-212 mimics or anti-miR-212 were stimulated with 2 mM MPP+ for 24 h, followed by detection of caspase-3 activity, SOD generation, ROS production, IL-1β expression, TNF-α levels, and LDH release. These experimental data demonstrated that MPP+ treatment results in a significant enhancement in caspase-3 activity (Fig. 2A), LDH release (Fig. 2B), ROS production (Fig. 2D), and TNF-α (Fig. 2E) and IL-1β (Fig. 2F) secretions, as well as a dramatic decrease in SOD level (Fig. 2C). However, these MPP+-elicited effects were attenuated by miR-212 overexpression and were exacerbated following miR-212 downregulation (Fig. 2). Taken together, these results suggested that miR-212 might alleviate MPP+-induced damage in SH-SY5Y cells.
To further investigate the underlying molecular mechanism of miR-212 in protecting against MPP+-induced injury in SH-SY5Y cells, TargetScan software was used to search for the endogenous targets of miR-212. Interestingly, the 3′-UTR of KLF4 was predicted to have a higher probability of binding to miR-212 (Fig. 3A). Thus, dual-luciferase reporter assay was performed to test whether KLF4 was a direct target of miR-212 in SH-SY5Y cells. As displayed in Fig. 3B, the luciferase activities of KLF4-WT reporter were repressed by upregulation of miR-212, while it was enhanced after suppression of miR-212 (Fig. 3B). Nevertheless, no difference was found in the luciferase activities of KLF4-Mu reporter following a change of miR-212 expression (Fig. 3B). Furthermore, miR-212 mimics or anti-miR-212 were transfected into SH-SY5Y cells to confirm the regulation role of miR-212 on KLF4 expression. RT-qPCR assay displayed that there was little influence on KLF4 mRNA levels when transfection with miR-212 mimics or anti-miR-212, compared with the control (Fig. 3C). Western blot assay presented that miR-212 upregulation repressed the expression of KLF4 protein, while miR-212 downregulation promoted KLF4 protein expression (Fig. 3D). These results indicated that miR-212 may modulate KLF4 expression in a post-transcriptional manner. Taken together, these data suggested that KLF4 is a direct target of miR-212 and that miR-212 inhibits the expression of KLF4 by binding to its 3′UTR.
To explore the role of KLF4 on MPP+-induced damage in SH-SY5Y cells, siRNA of KLF4 (si-KLF4) and KLF4 overexpression plasmid (KLF4) were used to reduce or elevate the expression levels of KLF4. As shown in Fig. 4A, the expression of KLF4 was remarkably down-regulated by transfection of si-KLF4, while it was substantially up-regulated upon introduction with pcDNA-KLF4, in comparison to their counterparts. Then, transfected SH-SY5Y cells were treated with 2 mM MPP+ for 24 h, followed by evaluation of cell viability and apoptosis. The results revealed that cell viability was markedly enhanced after KLF4 downregulation, whereas KLF4 overexpression led to a drop of cell viability, compared with corresponding controls, in MPP+-induced SH-SY5Y cells (Fig. 4B). As expected, MPP+-induced apoptosis was decreased by KLF4 knockdown, while it was in creased following KLF4 upregulation (Fig. 4C and D). Subsequently, to further investigate whether the protection effect of miR-212 was mediated by KLF4, SH-SY5Y cells were transfected with miR-212 mimics alone, or in combination with KLF4, prior to treatment with MPP+. Western blot assay displayed that miR-212-triggered reduction of KLF4 expression was significantly reversed by cotransfection with KLF4-overexpressing plasmid (Fig. 4E). Further, miR-212-mediated viability improvement was remarkably abated by the restoration of KLF4 expression in MPP+-induced SH-SY5Y cells (Fig. 4F). Moreover, the inhibitory effect of miR-212 on MPP+-induced apoptosis was greatly undermined by overexpressing KLF4 (Fig. 4G and H). All these results hinted that miR-212 exerts neuroprotective effect by regulating KLF4 in MPP+-induced SH-SY5Y cells.
Notch signaling, an important regulator of the nervous system, has been shown to be able to maintain immature neurons, modulate neurite outgrowth of differentiated neurons, and control synaptic plasticity and olfactory functions in the adult brain.15 Moreover, KLF4 was previously reported as an upstream regulator of Notch signaling in multiple biological processes.16 Thus, we further determined whether KLF4 could regulate Notch signaling in MPP+-induced SH-SY5Y cells by analyzing the expression levels of Notch signaling molecules following KLF4 overexpression or downregulation. The data revealed that KLF4 depletion leads to an apparent suppression of Notch1 and Jagged1 expression (Fig. 5A). Conversely, enforced expression of KLF4 considerably elevated the expression of Notch1 and Jagged1 (Fig. 5B). These results suggested that KLF4 could stimulate Notch signaling pathway. Further, we found that miR-212 upregulation suppressed the expression of Notch1 and Jagged1, whereas this inhibitory effect of miR-212 was reversed by the restoration of KLF4 expression (Fig. 5C and D). Taken together, these results suggested that miR-212 abates MPP+-induced injury of SH-SY5Y cells by blocking Notch signaling pathway via regulating KLF4.
PD is a chronic neurodegeneration disease in older adult individuals, characterized by resting tremor, rigidity, bradykinesia, and postural instability.1 To date, a number of documents have revealed that the pathophysiologic mechanisms underlying neurodegeneration are related to oxidative stress and inflammation response.3 SOD, an antioxidant enzyme, acts as a scavenger of ROS to prevent PD development.17 LDH, released by broken cells, can be measured as a surrogate for cell damage.18 IL-1β and TNF-α, two pivotal harmful inflammatory mediators, play vital roles in the development of PD.19 In this study, we employed MPP+-induced SH-SY5Y cells as a PD model in vitro. Our results showed that MPP+ treatment leads to a suppression of SH-SY5Y cell viability in a dose- or time-dependent manner. Moreover, MPP+ treatment promoted caspase-3 activity, LDH release, oxidative stress production, and inflammation response of SH-SY5Y cells. Altogether, our data implied that MPP+ treatment contributes to the damage of SH-SY5Y cells.
Up to now, several miRNAs have been implicated in MPP+-triggered PD model in SH-SY5Y cells. For instance, Wang, et al. 20 found that miR-124 upregulation affected apoptosis and impaired autophagy process in MPTP-treated mice and MPP+-induced SH-SY5Y cells by targeting a BH3-only protein (Bim). Additionally, it was reported that miR-7 upregulation improved viability and inhibited apoptosis in MPP+-induced SH-SY5Y cells by directly targeting KLF4.21 MiR-212, expressed in primary neuronal cultures, has been found to be able to exert a neuroprotective role in a series of human neurological diseases.11 For example, Wong, et al.22 reported that miR-212 overexpression protected neurons against oxidative stress and that miR-212 depletion induced neuronal apoptosis through targeting P300, PTEN, and FOXO3a in Alzheimer's disease. Also, suppression of miR-132/212 destroyed the balance of S-nitrosylation and promoted tau phosphorylation in a neuronal nitric oxide synthase (NOS1)-dependent way, contributing to the progression of Alzheimer's disease.23 Moreover, Gillardon, et al.24 stated that miR-212 may represent a potential therapeutic biomarker and target for human neurodegenerative diseases, such as PD. Our present results revealed that miR-212 is downregulated in MPP+-induced SH-SY5Y cells. miR-212 overexpression mitigated MPP+-induced decreases in SH-SY5Y cell viability. Moreover, miR-212 alleviated MPP+-induced SH-SY5Y cell damage, as evidenced by decreases in caspase-3 activity, LDH release, oxidative stress, and inflammation response. All these results implied that miR-212 plays a protective role against MPP+-induced cell damage of SH-SY5Y cells.
Blocking translation initiation is one of the main mechanisms of miRNA in regulating gene expression.25 Therefore, TargetScan software was employed to search for the candidate targets of miR-212. Interestingly, the data predicted that KLF4 contained a conserved sequence complementary with miR-212 at its 3′UTR. Subsequent dual-luciferase reporter assay, RT-qPCR, and western blot assay showed that miR-212 represses KLF4 expression in a post-transcriptional manner. KLF4, a member of the family of zinc-finger transcription factors, was reported to be implicated in multiple pathophysiological processes, such as cell growth, proliferation, differentiation, and inflammation.26 In this study, we found that exogenous expression of KLF4 lowered viability and promoted apoptosis in MPP+-stimulated SH-SY5Y cells. Moreover, KLF4 overexpression effectively reversed the effect of miR-212 on vitality and apoptosis in MPP+-administrated SH-SY5Y cells. Similarly, a recent document displayed that knockdown of KLF4 inhibited SH-SY5Y cell apoptosis under MPP+ treatment, and KLF4 upregulation abated the protective effect of miR-7 on MPP+-elicited apoptosis.21 Moreover, KLF4 was demonstrated to enhance oxidative stress, block proliferation, and promote LDH release in human dopamine neuroblastoma M17 cells induced by MPP+.27
Notch signaling has been reported to be important to the normal development of neurons, oligodendrocytes and astrocytes, and to affect neurological disorders of the central nervous system.28 In our current study, we found that the expression of Notch1 and Jagged1 were strikingly decreased after downregulating KLF4, while Notch1 and Jagged1 expression were increased when KLF4 was upregulated. In accordance with our finding, Yu, et al.16 also presented that the expressions of Notch1, Notch2, and Jagged1 were strikingly inhibited in KLF4-depletion cells, and KLF4 was verified to be a central regulator of sprouting angiogenesis by affecting Notch signaling.29 Moreover, we also found that miR-212 upregulation suppressed the expression of Notch1 and Jagged1, whereas the suppression effect of miR-212 was strikingly reversed by the regaining of KLF4 expression. All these results indicated that miR-124 inactivates Notch signaling via regulation of KLF4 in MPP+-induced SH-SY5Y cells.
In conclusion, our study suggests that miR-212 might attenuate MPP+-induced neuronal damage by regulating KLF4/Notch signaling pathway in SH-SY5Y cells, indicating the potential value of miR-212 as a biomarker and therapeutic target of PD. However, more in vivo data are required to further validate the role and mechanism of miR-212 in PD progression.
Figures and Tables
Fig. 1
MiR-212 attenuates MPP+-induced suppression of the viability of SH-SY5Y cells. (A) SH-SY5Y cells were treated with 2 mM of MPP+ for 12 h, and then RT-qPCR assay was performed to assess miR-212 expression patterns. (B) The expression levels of miR-212 were detected in SH-SY5Y cells transfected with miR-212 mimics or anti-miR-212. (C-F) SH-SY5Y transfected with miR-212 mimics or anti-miR-212 were treated with various concentrations (0, 1, 2, and 4 mM) of MPP+ for 24 h or with 2 mM of MPP+ for different times (0, 6, 12, and 24 h), followed by the detection of cell viability using CCK-8 at optical density 450 nm. *p<0.05 vs. negative control.
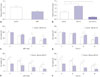
Fig. 2
MiR-212 ameliorates MPP+-induced damage of SH-SY5Y cells. SH-SY5Y cells transfected with miR-212 mimics or anti-miR-212 were treated with or without 2 mM MPP+ for 24 h, followed by ELISA assays of caspase-3 activity (A), LDH release (B), SOD level (C), ROS production (D), TNF-α expression (E), and IL-1β secretion (F). *p<0.05 vs. corresponding control. LDH, lactate dehydrogenase; SOD, superoxide dismutase; ROS, reactive oxygen species; TNF-α, tumor necrosis factor-α; IL-1β, interleukin-1 beta.
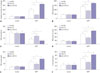
Fig. 3
KLF4 is a direct target of miR-212 in SH-SY5Y cells. (A) Sequence alignment between miR-212 and the 3′-UTR of KLF4. (B) Luciferase reporter assay was performed in SH-SY5Y cells after transfection with KLF4-WT or KLF4-Mu together with miR-212 mimics or anti-miR-212. SH-SY5Y cells were transfected with miR-212 mimics or anti-miR-212, followed by the measurement of KLF4 mRNA (C) and protein (D). p<0.05 vs. control. KLF4, Kruppel-like factor 4; 3′UTR, 3′-untranslated region; KLF4-WT, wild-type KLF4 reporter vector; KLF4-Mu, mutant-type KLF4 reporter vector; mRNA, messenger RNA.
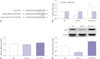
Fig. 4
MiR-212-mediated protection effect is abated following KLF4 expression restoration in MPP+-induced SH-SY5Y cells. SH-SY5Y cells transfected with si-NC, si-KLF4, control, or KLF4 were stimulated with 2 mM MPP+ for 24 h, followed by the detection of KLF4 protein (A), cell viability (B), and apoptosis (C and D). SH-SY5Y cells were transfected with miR-212 mimics alone or in combination with KLF4 prior to treatment with MPP+, followed by the analysis of KLF4 protein (E), cell viability (F), and apoptosis (G and H). *p<0.05 vs. corresponding control. KLF4, Kruppel-like factor 4.
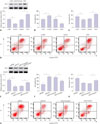
Fig. 5
Notch signaling pathway is involved in the regulation of miR-212/KLF4 axis in MPP+-induced SH-SY5Y cells. (A) Western blot assay of Notch1 and Jagged1 proteins in SH-SY5Y cells transfected with si-NC or si-KLF4 under MPP+ treatment. (B) Western blot analysis of Notch1 and Jagged1 proteins in SH-SY5Y cells transfected with control or KLF4 with the treatment of MPP+. (C and D) SH-SY5Y cells transfected with miR-212 mimics alone, or together with KLF4 prior to the treatment of MPP+, followed by the detection of KLF4, Notch1 and Jagged1 proteins expression. β-actin was used as the internal reference. *p<0.05 vs. respective control. KLF4, Kruppel-like factor 4.
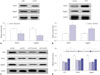
References
2. Abeliovich A, Gitler AD. Defects in trafficking bridge Parkinson's disease pathology and genetics. Nature. 2016; 539:207–216.


3. Andican G, Konukoglu D, Bozluolcay M, Bayülkem K, Bayülkem S, Burcak G. Plasma oxidative and inflammatory markers in patients with idiopathic Parkinson's disease. Acta Neurol Belg. 2012; 112:155–159.


4. Kalia LV, Kalia SK, Lang AE. Disease-modifying strategies for Parkinson's disease. Mov Disord. 2015; 30:1442–1450.


6. Harraz MM, Dawson TM, Dawson VL. MicroRNAs in Parkinson's disease. J Chem Neuroanat. 2011; 42:127–130.


7. Quinlan S, Kenny A, Medina M, Engel T, Jimenez-Mateos EM. MicroRNAs in neurodegenerative diseases. Int Rev Cell Mol Biol. 2017; 334:309–343.


8. Yang CP, Zhang ZH, Zhang LH, Rui HC. Neuroprotective role of microRNA-22 in a 6-hydroxydopamine-induced cell model of Parkinson's disease via regulation of its target gene TRPM7. J Mol Neurosci. 2016; 60:445–452.


9. Wang J, Le T, Wei R, Jiao Y. Knockdown of JMJD1C, a target gene of hsa-miR-590-3p, inhibits mitochondrial dysfunction and oxidative stress in MPP+-treated MES23.5 and SH-SY5Y cells. Cell Mol Biol (Noisy-le-grand). 2016; 62:39–45.
10. Li S, Lv X, Zhai K, Xu R, Zhang Y, Zhao S, et al. MicroRNA-7 inhibits neuronal apoptosis in a cellular Parkinson's disease model by targeting Bax and Sirt2. Am J Transl Res. 2016; 8:993–1004.
11. Wanet A, Tacheny A, Arnould T, Renard P. miR-212/132 expression and functions: within and beyond the neuronal compartment. Nucleic Acids Res. 2012; 40:4742–4753.


12. Aten S, Hansen KF, Hoyt KR, Obrietan K. The miR-132/212 locus: a complex regulator of neuronal plasticity, gene expression and cognition. RNA Dis. 2016; 3:e1375.


13. Burgos K, Malenica I, Metpally R, Courtright A, Rakela B, Beach T, et al. Profiles of extracellular miRNA in cerebrospinal fluid and serum from patients with Alzheimer's and Parkinson's diseases correlate with disease status and features of pathology. PLoS One. 2014; 9:e94839.


14. Maiti P, Manna J, Dunbar GL. Current understanding of the molecular mechanisms in Parkinson's disease: targets for potential treatments. Transl Neurodegener. 2017; 6:28.


15. Imai Y, Kobayashi Y, Inoshita T, Meng H, Arano T, Uemura K, et al. The Parkinson's disease-associated protein kinase LRRK2 modulates notch signaling through the endosomal pathway. PLoS Genet. 2015; 11:e1005503.


16. Yu F, Li J, Chen H, Fu J, Ray S, Huang S, et al. Kruppel-like factor 4 (KLF4) is required for maintenance of breast cancer stem cells and for cell migration and invasion. Oncogene. 2011; 30:2161–2172.


17. Xie XX, Kou ST, Pu ZH, Hou CY, Tian YP. [Effects of scalp catgut embedding on SOD, NO, MDA in the rat with Parkinson's disease]. Zhongguo Zhen Jiu. 2007; 27:753–756.
18. Popovich DG, Lee Y, Li L, Zhang W. Momordica charantia seed extract reduces pre-adipocyte viability, affects lactate dehydrogenase release, and lipid accumulation in 3T3-L1 cells. J Med Food. 2011; 14:201–208.


19. Nagatsu T, Sawada M. Inflammatory process in Parkinson's disease: role for cytokines. Curr Pharm Des. 2005; 11:999–1016.


20. Wang H, Ye Y, Zhu Z, Mo L, Lin C, Wang Q, et al. MiR-124 regulates apoptosis and autophagy process in MPTP model of Parkinson's disease by targeting to Bim. Brain Pathol. 2016; 26:167–176.


21. Kong B, Wu PC, Chen L, Yang T, Yuan YQ, Kuang YQ, et al. microRNA-7 protects against 1-methyl-4-phenylpyridinium iodide-induced cell apoptosis in SH-SY5Y cells by directly targeting Krüpple-like factor 4. DNA Cell Biol. 2016; 35:217–225.


22. Wong HK, Veremeyko T, Patel N, Lemere CA, Walsh DM, Esau C, et al. De-repression of FOXO3a death axis by microRNA-132 and -212 causes neuronal apoptosis in Alzheimer's disease. Hum Mol Genet. 2013; 22:3077–3092.


23. Wang Y, Veremeyko T, Wong AH, El Fatimy R, Wei Z, Cai W, et al. Downregulation of miR-132/212 impairs S-nitrosylation balance and induces tau phosphorylation in Alzheimer's disease. Neurobiol Aging. 2017; 51:156–166.


24. Gillardon F, Mack M, Rist W, Schnack C, Lenter M, Hildebrandt T, et al. MicroRNA and proteome expression profiling in early-symptomatic α-synuclein(A30P)-transgenic mice. Proteomics Clin Appl. 2008; 2:697–705.


26. Ghaleb AM, Yang VW. Krüppel-like factor 4 (KLF4): what we currently know. Gene. 2017; 611:27–37.


27. Chen J, Wang X, Yi X, Wang Y, Liu Q, Ge R. Induction of KLF4 contributes to the neurotoxicity of MPP+ in M17 cells: a new implication in Parkinson's disease. J Mol Neurosci. 2013; 51:109–117.

