Abstract
Purpose
Removal of CO2 is much efficient during high-frequency oscillatory ventilation (HFOV) for preterm infants. However, an optimal carbon dioxide diffusion coefficient (DCO2) and tidal volume (VT) have not yet been established due to much individual variance. This study aimed to analyze DCO2 values, VT, and minute volume in very-low-birth-weight (VLBW) infants using HFOV and correlates with plasma CO2 (pCO2).
Materials and Methods
Daily respiratory mechanics and ventilator settings from twenty VLBW infants and their two hundred seventeen results of blood gas analysis were collected. Patients were treated with the Dräger Babylog VN500 ventilator (Drägerwerk Ag & Co.) in HFOV mode. The normocapnia was indicated as pCO2 ranging from 45 mm Hg to 55 mm Hg.
Results
The measured VT was 1.7 mL/kg, minute volume was 0.7 mL/kg, and DCO2 was 43.5 mL2/s. Mean results of the blood gas test were as follows: pH, 7.31; pCO2, 52.6 mm Hg; and SpO2, 90.5%. In normocapnic state, the mean VT was significantly higher than in hypercapnic state (2.1±0.5 mL/kg vs. 1.6±0.3 mL/kg), and the mean DCO2 showed significant difference (68.4±32.7 mL2/s vs. 32.4±15.7 mL2/s). The DCO2 was significantly correlated with the pCO2 (p=0.024). In the receiver operating curve analysis, the estimated optimal cut-off point to predict the remaining normocapnic status was a VT of 1.75 mL/kg (sensitivity 73%, specificity 80%).
High-frequency oscillatory ventilation (HFOV) is now widely used as an artificial ventilator that actively performs 180−900 breaths per minute with a very small tidal volume (VT).1 It is used as rescue therapy for severe respiratory failure in premature infants, and it contributes to reduce the incidence of bronchopulmonary dysplasia.2345
The settings of the HFOV are based on the fraction of inspired oxygen (FiO2) for oxygen (O2) saturation, mean arterial pressure (MAP), which is about 2−4 cm H2O higher than the average airway pressure of conventional mechanical ventilation, amplitude of chest vibration to the umbilicus, and frequency of approximately 10−15 Hz.678 On the basis of the carbon dioxide diffusion coefficient (DCO2) and VT, physicians can confirm the effect of oxygenation and ventilation.9
In particular, the DCO2 indicates the effectiveness of CO2 removal. It has various values depending on the size of the lung, and it is calculated as the product of the squared VT and the frequency.1011 Setting parameters in HFOV, according to the lung pathology, is left to the discretion of the physician, and appropriate DCO2 and VT values have not yet been established. When the DCO2 is below 40 mL2/s, it has been reported that the probability of a pCO2 below 50 mm Hg is estimated to be 49%, and the appropriate DCO2 value for extremely low-birth-weight (ELBW) infants has been reported as 18.5 mm Hg.12 VT refers to VT averaged over several cycles, and it is generally recommended to set it to 1.5−2 mL/kg.12 This, however, depends on the lung mechanics and ventilator mechanics, since the volume of the lung increases as the frequency of ventilation decreases. For greater lung compliance, an ideal VT should be maintained in order to prevent hyperinflation.13 One study reported that the set VT values varied between from 1.46 mL/kg for ELBW infants and 1.57 mL/kg for infants weighing 1000−2000 g.14 In another preterm study of infants <32 weeks of gestation, the targeted VT ranged from 1.5 mL/kg to 2.5 mL/kg, and only 51% of infants had normocapnia.12
In the present study, we aim to analyze the respiratory mechanics, especially DCO2 values, VT, and minute volume and correlate them with plasma CO2 (pCO2) in very-low-birth-weight (VLBW) infants using HFOV.
This retrospective study comprised 20 VLBW infants, who received HFOV without volume guarantee for treatment of respiratory failure. These patients had all been admitted to the neonatal intensive care unit of Gangnam Severance Hospital between March 2015 and February 2016. Their two hundred seventeen results of blood gas analysis were collected. Ethical approval was obtained from the ethics committees of Gangnam Severance Hospital and informed consent was waived (IRB approval number; 3-2017-0120).
HFOV was delivered using a Dräger Babylog VN500 ventilator (Drägerwerk Ag & Co., Lübeck, Germany) with a Venturi system. It generates active inspiration and active expiration to induce a sinusoidal pressure signal around a set mean airway pressure. The Dräger Babylog VN500 ventilator uses a double hot-wire anemometer, which has been demonstrated to be a very accurate way of measuring frequency of expired VT during HFOV, as there is greater linearity of response across a range of frequencies.15
HFOV was set as a mean airway pressure of 1 to 2 cm H2O higher than that received during conventional mechanical ventilation and the same inspired oxygen fraction. In chest radiograph, the lung volume was evaluated with the right diaphragm at the level of the ninth rib. A frequency of 10−12 Hz and an oscillatory inspiratory/expiratory ratio of 1:1 were used in all infants. An amplitude set above the mean airway pressure value was increased until visible chest wall movement up to the umbilicus level was noted.
If the PCO2 value was outside the target range, the amplitude was adjusted up or down in increments of 10−20% as necessary in the HFOV alone period. Capillary blood gases were measured 1 hour after applying HFOV and then at 6−8-hour intervals or more often as needed using a blood gas analyser. The FiO2 was given as needed to achieve a peripheral capillary oxygen saturation (SpO2) between 90% and 95% by pulse oximetry.
During ventilation, a hot-wire anemometer was placed on the airway opening to continuously measure the flow and VT during HFOV. The VT, Paw, minute volume, and DCO2 were calculated as VT2×frequency (mL2/s) by the ventilator (Dräger Babylog VN500 2.n software, Dräger) and recorded at 1 minute. Data from the ventilator were exported through a standard USB connection.
The following data were also collected from patients included in the study: demographic characteristics, perinatal pathology and treatment, clinical course, initial and final ventilator settings, vital signs, and capillary blood gas analysis. When analysing the blood gas analysis, pCO2 values between 45 and 55 mm Hg were considered normocapnia.
The study population consisted of 20 VLBW infants with a median gestational age of 28.3 weeks and median birth weight of 1050 g. The 1-minute Apgar score was 2.5, and the 5-minute Apgar score was 5.4 points. Age at the start of HFOV was 5.3 days, and the duration of HFOV was 10.3 days. Surfactant replacement therapy was administered to all the infants, and five infants received multiple therapy. No patients with congenital anomalies affecting the cardiac, respiratory or central nervous system were found. Eleven infants had a maternal history of chorioamnionitis, and 15 received steroid treatment (Table 1).
The ventilator parameters were set as follows: inspiratory-to-expiratory (I/E) time ratio, 1:1; frequency, 10.5 Hz; amplitude, 23.6 cm H2O; and MAP, 11.7 cm H2O. The measured VT was 1.7 mL/kg, minute volume was 0.7 mL/kg, and DCO2 was 43.5 mL2/s. Median results of the blood gas test were as follows: pH, 7.31; pCO2, 52.6 mm Hg; and SpO2, 90.5% (Table 2).
In a comparison of the normocapnia and hypercapnia groups, the VT, minute volume, and DCO2 were statistically significantly different. The mean VT of the normocapnia group was 2.1 mL/kg, minute volume was 0.9 mL/kg, and DCO2 was 68.4 mL2/s (Table 3). The relationship between pCO2 and DCO2 was negative. The correlation between the VT and pCO2 was also negative, shown in Fig. 1. When we divided infants into 4 groups according to the DCO2 value, the percentage of infants with normocapnia corresponding to a pCO2 <55 mm Hg was 83% for a DCO2 40−60 mL2/s in each DCO2 group (Table 4). According to the receiver operating characteristic curves, the area under the curve for normocapnic status was 0.833 (p=0.041). The estimated optimal cut-off points to predict the remaining normocapnic status was a VT of 1.75 mL/kg (sensitivity 73%, specificity 80%) (Fig. 2).
HFOV is widely used as a treatment of choice and rescue therapy for respiratory failure in preterm infants. The use of elective HFOV can result in a reduction in the risk of chronic lung disease.7 However, the respiratory mechanics in infants ventilated with the Dräger Babylog VN500 ventilator or other HFOV devices has much individual variance, thus few studies have reported appropriate data. The present study is meaningful, as it is the first one to assess the respiratory mechanics using the Dräger Babylog VN500 ventilator and to analyse the ventilation status of Korean VLBW infants.
Severe hypercapnia and hypocapnia may cause neonatal brain injury. These conditions can induce changes in the cerebral blood volume.16 The association between hypocapnia and periventricular leukomalacia in preterm infants is well documented.1718 Severe hypercapnia is associated with organ dysfunction, including barotrauma, renal dysfunction, cardiovascular dysfunction, and an increased motality risk in the intensive care unit (odds ratio 1.93).19 Furthermore, a high pCO2 value has been shown to be a risk factor for severe intraventricular haemorrhage.20 Therefore, it is essential to maintain normocapnia in patients under ventilator care. However, frequent sampling to check the pCO2 level is limited in VLBW infants.
HFOV is effective for ventilation control compared to conventional mechanical ventilation.21 Targeting the ideal DCO2 may induce a more stable PCO2 level, which has the potential to reduce both hypercarbia and hypocarbia. However, the DCO2 has various values depending on the size of the lung and underlying conditions, and the absolute target value has not yet been confirmed.22 In a previous study, the mean values of DCO2 according to body weight were reported according to the weight ranges in extremely low birth weight infants, in those weighing between 1000 g and 2000 g, and in those weighing over 2000 g.14 In the present study, we confirmed that DCO2 and pCO2 values were more useful to maintain normocapnia with proper ventilation than DCO2 values of above 40 mL2/s in VLBW infants. In a neonatal animal model, no significant changes on CO2 elimination are observed during HFOV with I/E ratios of 1:1 and 1:2 both.23
Considering the correlation between lung pressure and lung inflation, maintenance of adequate VT between under-inflation and over-inflation is essential to prevent barotrauma.2425 It has been reported that the VT improved CO2 elimination, and it had a greater effect than the frequency of ventilation.2326 HFOV is useful for maintaining the VT within the target range of normocapnia, and it is associated with a low incidence of hypocapnia and hypercapnia compared to conventional ventilation. The most important consideration is that the optimal initial value of VT is not yet clearly known. VT decreases with increasing frequency, and it increases with increasing compliance and the tracheal tube diameter. One study used a target VT of 2 mL/kg, as the expected dead space was thought to be approximately 2.2 mL/kg in healthy, awake neonates.27
To date, few studies have evaluated the optimal VT target in neonates during HFOV, because these values vary with gestational or postnatal age and underlying disease. In a study of infants who had been ventilated by HFOV at all time during their hospital days, the median gestation age was 27.2 weeks (range, 23.3−41.0 weeks) and respiratory distress syndrome (RDS) was most commonly diagnosed (56%), followed by lung hypoplasia (14%). The median delivered normocapnic VT during HFOV was 1.67 mL/kg, and the median suggested hypocapnic and hypercapnic VTs were 1.94 mL/kg and 1.54 mL/kg, respectively.28 Other studies demonstrated that the median delivered normocapnic VT during HFOV was 1.74 mL/kg, median hypocapnic VT was 1.97 mL/kg, and median hypercapnic VT was 1.54 mL/kg in preterm infants during the initial phase of RDS.12 A previous study showed that the adequate VT was 1.46 mL/kg for ELBW infants, 1.57 mL/kg for infants weighing 1000−2000 g, and 2.27 mL/kg for infants weighing over 2000 g. In the present study, the mean VT for normocapnia was 2.1 mL/kg, and the minute volume was 0.9 mL/kg in VLBW infants.14
Another study showed that an increase in VT from 2 to 3 mL/kg resulted in a decrease in the pCO2 level from 60±11 mm Hg to 51±8 mm Hg with concomitant increases in the amplitude and DCO2 in a newborn piglet model, and the DCO2 value was 68.4 mL2/s.29 In this study, the correlation between the VT and pCO2 was also significantly negative.
The present study has a limitation in the exact analysis of normocapnia and hypercapnia because of the small number of patients and failure to distinguish between arterial, venous, and capillary blood. The individual variances about lung pathology and underlying condition were not adjusted. Because fixed frequency was not used though 10−12 Hz small ranges, the effect of decreased VT with increasing frequency was ignored. Actually, when the frequency changes from 10 Hz to 12 Hz, the dependency of oscillatory volume on frequency changes from 6.6 mL to 4.6 mL in 10 mbar MAP.30 Because of the small number of patients, we couldn't divide the result of the blood test by frequency of the HFOV. We also examined only short-term physiological and respiratory variables; thus, the potential long-term benefits are only speculative.
In conclusion, it is advisable to maintain a normocapnia state with a VT of 1.75 mL/kg in VLBW infants and a DCO2 value of ≥40 mL2/s. Further studies are needed to establish this topic further.
ACKNOWLEDGEMENTS
This work was supported by a National Research Foundation of Korea grant, funded by the Korea government (Number 2011-0014207).
References
1. Bouchut JC, Godard J, Claris O. High-frequency oscillatory ventilation. Anesthesiology. 2004; 100:1007–1012. PMID: 15087640.


2. Cools F, Askie LM, Offringa M, Asselin JM, Calvert SA, Courtney SE, et al. Elective high-frequency oscillatory versus conventional ventilation in preterm infants: a systematic review and meta-analysis of individual patients' data. Lancet. 2010; 375:2082–2091. PMID: 20552718.


3. Guo YX, Wang ZN, Li YT, Pan L, Yang LF, Hu Y, et al. High-frequency oscillatory ventilation is an effective treatment for severe pediatric acute respiratory distress syndrome with refractory hypoxemia. Ther Clin Risk Manag. 2016; 12:1563–1571. PMID: 27799777.


4. Poddutoor PK, Chirla DK, Sachane K, Shaik FA, Venkatlakshmi A. Rescue high frequency oscillation in neonates with acute respiratory failure. Indian Pediatr. 2011; 48:467–470. PMID: 21555797.


5. Sun H, Cheng R, Kang W, Xiong H, Zhou C, Zhang Y, et al. High-frequency oscillatory ventilation versus synchronized intermittent mandatory ventilation plus pressure support in preterm infants with severe respiratory distress syndrome. Respir Care. 2014; 59:159–169. PMID: 23764865.


6. Weber K, Courtney SE, Pyon KH, Chang GY, Pandit PB, Habib RH. Detecting lung overdistention in newborns treated with high-frequency oscillatory ventilation. J Appl Physiol (1985). 2000; 89:364–372. PMID: 10904073.


7. Claris O, Salle BL. High frequency oscillatory ventilation and the prevention of chronic lung disease. Pediatr Pulmonol Suppl. 1997; 16:33–34. PMID: 9443187.


8. Lia Graciano A, Freid EB. High-frequency oscillatory ventilation in infants and children. Curr Opin Anaesthesiol. 2002; 15:161–166. PMID: 17019196.


9. Ochiai R. [What should we know about respiratory physiology for the optimal anesthesia management?]. Masui. 2016; 65:442–451. PMID: 27319088.
10. Boynton BR, Hammond MD, Fredberg JJ, Buckley BG, Villanueva D, Frantz ID 3rd. Gas exchange in healthy rabbits during high-frequency oscillatory ventilation. J Appl Physiol (1985). 1989; 66:1343–1351. PMID: 2496093.


11. Fredberg JJ, Glass GM, Boynton BR, Frantz ID 3rd. Factors influencing mechanical performance of neonatal high-frequency ventilators. J Appl Physiol (1985). 1987; 62:2485–2490. PMID: 3475269.


12. Iscan B, Duman N, Tuzun F, Kumral A, Ozkan H. Impact of volume guarantee on high-frequency oscillatory ventilation in preterm infants: a randomized crossover clinical trial. Neonatology. 2015; 108:277–282. PMID: 26330156.


13. Chang HK. Mechanisms of gas transport during ventilation by high-frequency oscillation. J Appl Physiol Respir Environ Exerc Physiol. 1984; 56:553–563. PMID: 6368498.


14. González-Pacheco N, Sánchez-Luna M, Ramos-Navarro C, Navarro-Patiño N, de la Blanca AR. Using very high frequencies with very low lung volumes during high-frequency oscillatory ventilation to protect the immature lung. A pilot study. J Perinatol. 2016; 36:306–310. PMID: 26741575.


15. John J, Harcourt ER, Davis PG, Tingay DG. Dräger VN500's oscillatory performance has a frequency-dependent threshold. J Paediatr Child Health. 2014; 50:27–31. PMID: 24118670.


16. Ito H, Ibaraki M, Kanno I, Fukuda H, Miura S. Changes in the arterial fraction of human cerebral blood volume during hypercapnia and hypocapnia measured by positron emission tomography. J Cereb Blood Flow Metab. 2005; 25:852–857. PMID: 15716851.


17. Hatzidaki E, Giahnakis E, Maraka S, Korakaki E, Manoura A, Saitakis E, et al. Risk factors for periventricular leukomalacia. Acta Obstet Gynecol Scand. 2009; 88:110–115. PMID: 18951221.


18. High-frequency oscillatory ventilation compared with conventional mechanical ventilation in the treatment of respiratory failure in preterm infants. The HIFI Study Group. N Engl J Med. 1989; 320:88–93. PMID: 2643039.
19. Nin N, Muriel A, Peñuelas O, Brochard L, Lorente JA, Ferguson ND, et al. Severe hypercapnia and outcome of mechanically ventilated patients with moderate or severe acute respiratory distress syndrome. Intensive Care Med. 2017; 43:200–208. PMID: 28108768.


20. Levene MI, Fawer CL, Lamont RF. Risk factors in the development of intraventricular haemorrhage in the preterm neonate. Arch Dis Child. 1982; 57:410–417. PMID: 7092304.


21. Friesecke S, Stecher SS, Abel P. High-frequency oscillation ventilation for hypercapnic failure of conventional ventilation in pulmonary acute respiratory distress syndrome. Crit Care. 2015; 19:201. PMID: 25929255.


22. Kurata T, Ohta Y, Kondo T, Kuwahira I, Hayashi Y. O2 uptake and CO2 elimination during mechanical ventilation with high frequency oscillation. Tokai J Exp Clin Med. 1991; 16:133–143. PMID: 1780914.
23. Sánchez-Luna M, González-Pacheco N, Santos M, Blanco Å, Orden C, Belik J, et al. Effect of the I/E ratio on CO2 removal during high-frequency oscillatory ventilation with volume guarantee in a neonatal animal model of RDS. Eur J Pediatr. 2016; 175:1343–1351. PMID: 27595847.


24. Muhlethaler V, Malcolm G. Mechanical ventilation in the newborn; a simplified approach. Part 2: High-frequency ventilation. J Paediatr Child Health. 2014; 50:E10–E13. PMID: 20977521.


25. Dargaville PA, Tingay DG. Lung protective ventilation in extremely preterm infants. J Paediatr Child Health. 2012; 48:740–746. PMID: 22970667.


26. Wong R, Deakers T, Hotz J, Khemani RG, Ross PA, Newth CJ. Volume and pressure delivery during pediatric high-frequency oscillatory ventilation. Pediatr Crit Care Med. 2017; 18:e189–e194. PMID: 28212162.


27. Lagneaux D, Mossay C, Geubelle F, Christiaens G. Alveolar data in healthy, awake neonates during spontaneous ventilation: a preliminary investigation. Pediatr Pulmonol. 1988; 5:225–231. PMID: 3237450.


28. Zimová-Herknerová M, Plavka R. Expired tidal volumes measured by hot-wire anemometer during high-frequency oscillation in preterm infants. Pediatr Pulmonol. 2006; 41:428–433. PMID: 16547962.


29. Sánchez Luna M, Santos González M, Tendillo Cortijo F. High-frequency oscillatory ventilation combined with volume guarantee in a neonatal animal model of respiratory distress syndrome. Crit Care Res Pract. 2013; 2013:593915. PMID: 23970963.


30. Stachow R. High-frequency ventilation−basics and practical applications. Lübeck, Germany: Drägerwerk AG;1995.
Fig. 1
Correlation analysis between DCO2 and pCO2 and between VT and pCO2. DCO2 values showed negative correlation with pCO2. VT showed negative correlation with pCO2. DCO2, carbon dioxide diffusion coefficient; pCO2, plasma CO2; VT, tidal volume.
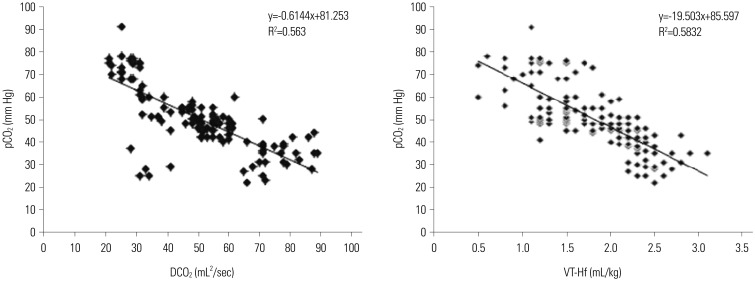
Fig. 2
ROC curve analysis. On the ROC curve analysis, setting of the tidal volume at 1.75 mL/kg was able to maintain normocapnia with a sensitivity of 73%, specificity of 80%, and an AUC of 0.833. ROC, receiver operating characteristic curves; AUC, area under the curve.
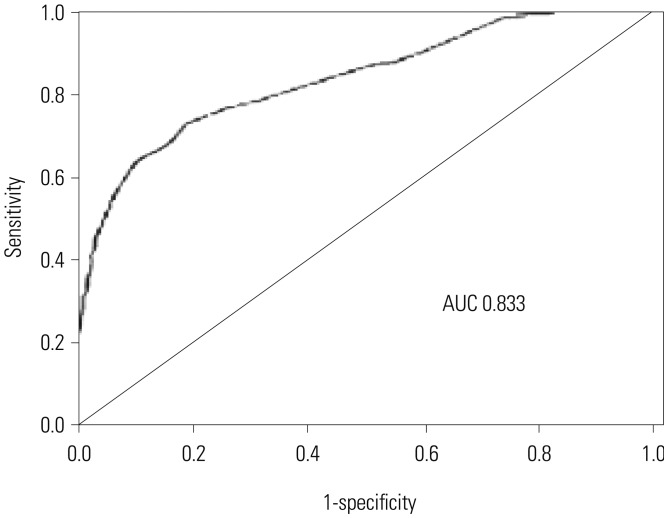
Table 1
Patient Characteristics
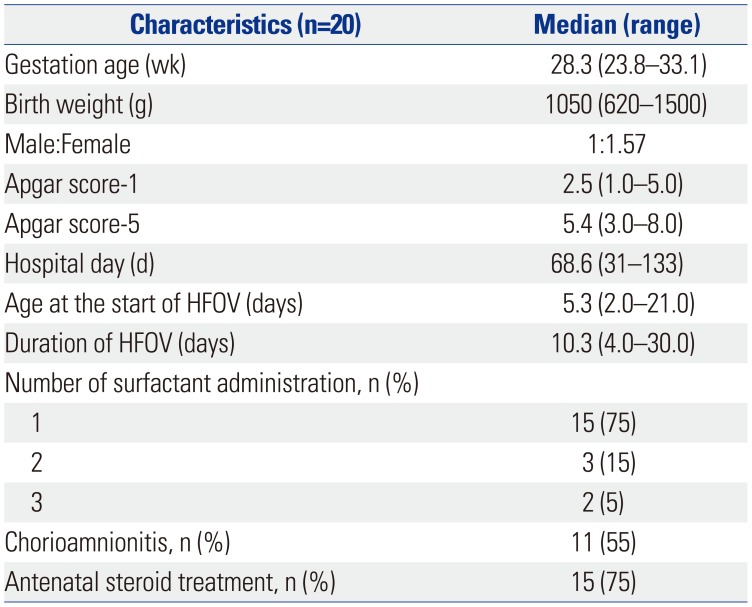
Table 2
Ventilator Parameters and Results of Blood Gas Analysis
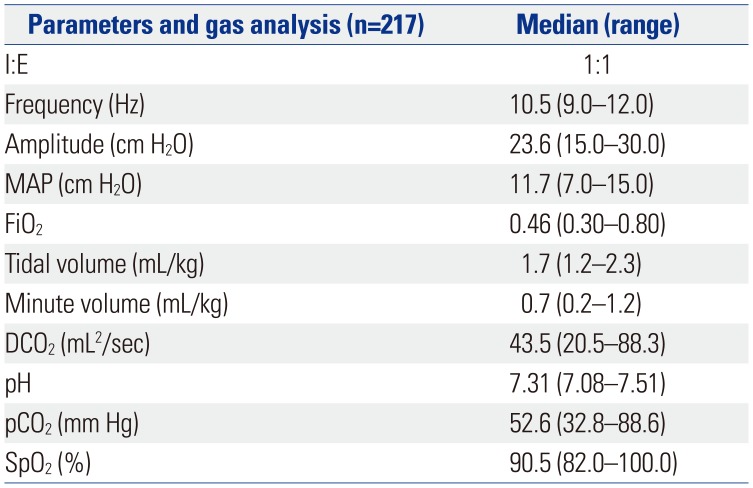
Table 3
Comparisons of Parameters between Normocapnia and Hypercapnia Group
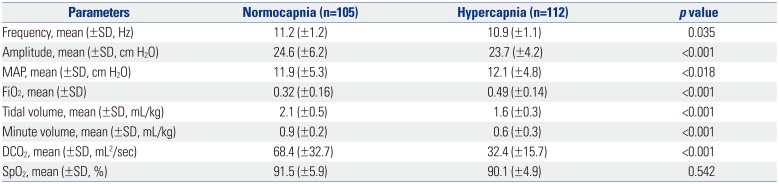