Abstract
Purpose
Cancer stem like cells (CSCs), with unlimited self-renewal potential and other stem cell characteristics, occur in several cancers including hepatocellular carcinoma (HCC). Although CSCs can initiate tumors, malignant proliferation, relapse and multi-drug resistance, the ways how to activate them still remain unknown. This study aims to evaluate whether CSC acquire tumorigenic characters under tumor hypoxia, analyzed by microarray analysis.
Materials and Methods
CSCs were purified from HCC patients and Affymetrix microarray was used to investigate their gene expression profiles. The results were validated by real-time polymerase chain reaction (PCR).
Results
The results of the microarray indicated that 18 genes were up-regulated and 10 genes were down-regulated in CSCs. Several genes were identified to be significantly involved in the regulation of CSCs such as HCC. Furthermore, the up-regulated genes were related with metabolism, angiogenesis and hypoxia, whereas the down-regulated genes were related with apoptosis and inflammation.
Hepatocellular carcinoma (HCC) is the sixth most common cancer in the world, and the second most lethal cancer.12 About 80–90% of cirrhotic liver disease due to chronic viral hepatitis B (HBV) or C (HCV) develop into HCC.2 Moreover, most cases of advanced HCC develop hypoxic induction with angiogenesis and growth programs.1 Tumor hypoxia is typically associated with poor patient prognosis,3 partly because low oxygen levels reduce the effectiveness of radiation therapy,4 which kills tumor cells by generating reactive oxygen species.3 Regions of severe oxygen deprivation (hypoxia) arise in tumors due to rapid cell division and aberrant blood vessel formation.5 Localized hypoxia in normal tissues can promote tumor progression by altering cellular metabolism and stimulating angiogenesis.15 The most important factors that affect HCC progression are oxygen and nutrients.6 The liver is an organ with a specific blood supply.5 Approximately 25% of blood enter the liver through the hepatic artery, while 75% enter through the portal vein.15 As such, large amounts of nutrients and oxygen are required for HCC cell proliferation, resulting in localized hypoxia.357
Low oxygen levels are a defining characteristic of solid tumors,8 and responses to hypoxia contribute substantially to the malignant phenotype.9 Hypoxia-induced gene transcription promotes characteristic tumor behaviors, including angiogenesis, invasion, metastasis, de-differentiation and enhanced glycolytic metabolism.19 Normal stem cells are physically located in specific physical and functional anatomical locations or niches that are essential for maintenance of self-renewal and an undifferentiated state.10 On the other hand, cancer stem like cells (CSCs) promote the development of their own perivascular niche through the secretion of proangiogenic factors, prominently vascular endothelial growth factor (VEGF), but remain dependent on the niche.1011 Recent experimental evidence has expanded the role of hypoxia in cancer by demonstrating differential responses to hypoxia between heterogenic subpopulations within the tumor.1213 One of these populations, called CSC, possess many phenotypic similarities to normal stem cells, such as having the ability to up-regulate DNA repair kinases to avert radiation-induced genomic damage.14 Although somewhat controversial, hypoxia has been considered to be increasingly related to the cancer stem cell phenotype.15
CSCs contribute to tumor growth, maintenance, and recurrence after therapy through multiple mechanisms and networks.1016 One important characteristic of these cells is their ability to restrict DNA damage, sustained during radiation or chemotherapy, by reduction of reactive oxygen species (ROS) and enhanced activity of DNA checkpoint kinases.1718 By preventing DNA damage, the cancer stem cell population survive injury and can continue to propagate the tumor.19 In addition, one of the important roles which the cancer stem cell population play in a tumor is to regulate tumor angiogenesis by VEGF signaling.20 The cancer stem cell subpopulation has been shown to be the primary regulator of angiogenesis in a tumor by the production of VEGF.2021 The chaotic vessel formation is an another factor in the constant oxygen flux observed in solid tumors.22 High frequency of cycling (acute) hypoxia correlates to lower patient survival.4 This sensitivity of the tumor structure to oxygen status is driven by the hypoxia inducible factor (HIF) proteins in the cancer stem cell population.23
CSC has emerged as a key factor in malignant tumors that recur after surgery or treatment like transcatheter arterial chemoembolization (TACE).24 The analysis of CSC characteristics, showed that genetic alterations affected by hypoxic stimulation are responsible for the malignant conversion of CSC and play an important role in liver cancer treatment.25 Thus, this study aimed to identify specific characteristics of CSC and analyse differences in the gene expressions under hypoxia and normoxia to explore the mechanism of malignant progression.
To isolate cancer stem-like cells and adipose derived stem cells (ADSCs) from patients with partial hepatectomy, tissues were harvested under anesthesia according to the procedure approved by the Institutional Review Board (IRB; 4-2013-0757) of Yonsei University Health System. Liver cancer tissues and subcutaneous adipose tissues were washed with phosphate buffered saline (PBS), minced into 0.5-mm squares using surgical scissors, and digested at 37℃ for 30 min using 0.2% collagenase type I (Roche, Penzberg, Germany). Enzyme activity was neutralized with Dulbecco's Modified Eagle Medium (DMEM; Gibco BRL, Grand Island, NY, USA), containing low glucose. 10% fetal bovine serum (FBS; WelGENE, Daegu, Korea), and 1% antibiotic/antimycotic solution (Invitrogen, Grand Island, NY, USA). Samples were centrifugated at 1200 g for 10 min to obtain a pellet. After removing the supernatant, the pellet was resuspended in the medium, filtered through a cell strainer (100-µm pore size; BD Biosciences, Seoul, Korea) to remove cellular debris, and incubated overnight at 37℃/5% CO2 in the above DMEM. After 24 h of incubation, the plates were washed with PBS to remove residual nonadherent red blood cells, and the remaining cells were incubated in control medium at 37℃/5% CO2.
Human HCC cells (Huh7) and human pancreatic cancer cells (SNU478) were obtained from Korean Cell Line Bank (Seoul, Korea). They (Huh7, SNU478) were cultured at a density of 10000 cells/cm2 in DMEM high-glucose medium supplemented with 10% inactivated FBS (WelGENE) and 1% antibiotic/antimycotic (Invitrogen), and incubated at /37℃ with 5% CO2.
When cell confluence reached 80%, the cells were detached using 1×trypsin-EDTA. Cells free from serum were suspended individually in DMEM/F12 medium supplemented with 1% b27 supplement, 1% antibiotic-antimycotic (Invitrogen), 20 ng/mL epidermal growth factor (EFG; Invitrogen, Grand Island, NY, USA), and 20 ng/mL basic fibroblast growth factor (bFGF; Invitrogen, Seoul, Korea) [DMEG(+)growth factor (GF)], DMEM-high glucose with FBS {DMEM(+)FBS, or DMEM-high glucose without FBS [DMEM(-)FBS]}. Cells were subsequently cultured in ultra-low attachment 24-well plates (24 well plate coated with Ultra-Low Attachment Surface, Corning, NY, USA) at a density of 10000 cells per well, and were incubated at 37℃ with 5% CO2.
After sphere-forming culture, a photo of each medium condition was observed under a microscope (Olympus America, Melville, NY, USA) on days 3, 7, 15, and 20 to observe cell morphology.
Total RNA was extracted from HCC cells using Trizol reagent (Gibco-BRL) according to the manufacturer's instructions. The pelleted dried total RNA was dissolved in diethylpyrocarbonate-treated water, and the concentration and purity of the RNA were assessed by measuring A260 and A280 on a NanoDrop (ND-100) spectrophotometer (Laboratory & Medical Supplies, Tokyo, Japan). From each total RNA sample, 1 µg of RNA was used as a template for cDNA synthesis using reverse transcriptase (Invitrogen), according to the manufacturer's instructions. The cDNA was then analyzed and quantified using real-time quantitative PCR.
Cells were collected from each medium type at days 7, 15, and 20. Cells were washed in PBS and immediately fixed in 10% formaldehyde solution. Each cell sample was then exposed to CD133, CD90, E-cadherin, and beta-catenin primary antibodies. All primary antibodies were purchased from Santa Cruz Biotechnology (Santa Cruz Biotechnology, Dallas, TX, USA). After a 24-h incubation, the anti-rabbit IgG HRL-F (ab) and 2-PE anti-goat IgG-FITC (Santa Cruz, TX, USA) secondary antibodies were added. Immunostained cells were observed with a fluorescence microscope. For confocal microscopy, cells were fixed in glass chamber slides with 10% paraformaldehyde in PBS for 30 min at room temperature. After two washes with PBS, cells were permeabilized with 100% methanol for 5 min at room temperature. Cells were washed twice with PBS and blocked for 45 min in 5% BSA in PBS. Confocal microscopy studies were carriedout using a laser-scanning microscope from OLYMPUS (Tokyo, Japan).
The sphere-forming cells were separated into single cells using 1×trypsin EDTA, centrifuged to precipitate, and cleansed with 0.5% BSA and 2 mM EDTA in PBS. PE conjugated antihuman CD133/2 monoclonal antibody (mAb) (Miltenyi Biotec, Bergisch Gladbach, Germany) was then added. After acquiring a cell pellet by the method described above, the primary antibody specific to Connexin 32 was incubated with the pellet at 4℃ for 24 h. The secondary antibody was added at room temperature for 1 h, and the fluorescence was measured using flow cytometry. BD human pluripotent stem cell transcription factor analysis kit (BD Biosciences, San Jose, CA, USA) was used to measure the pluripotency markers Oct4, Nanog, and Sox2.
We performed global gene expression analysis using Affymetrix GeneChip® (Affymetrix, Santa Clara, CA, USA) Human Gene 2.0 ST Arrays. The sample was prepared according to the instructions and recommendations provided by the manufacturer. Total RNA was isolated using RNeasy Mini Kit columns as described by the manufacturer (Qiagen, Hilden, Germany). Quality of RNA was assessed by Agilent 2100 bioanalyser using the RNA 6000 Nano Chip (Agilent Technologies, Santa Clara, CA, USA), and quantity was determined by Nanodrop-1000 Spectrophotometer (Thermo SCIENTIFIC). Three hundred ng of RNA sample was used as input into the Affymetrix procedure as recommended by protocol (http://www.affymetrix.com). Briefly, 300 ng of total RNA from each sample was converted to double-strand cDNA. Using a random hexamer incorporating a T7 promoter, amplified RNA (cRNA) was generated from the double-stranded cDNA template through an IVT (in-vitro transcription) reaction and purified with Affymetrix sample cleanup module. cDNA was regenerated through a random-primed reverse transcription using a dNTP mix containing dUTP. The cDNA was then fragmented by UDG and APE 1 restriction endonucleases and end-labeled by terminal transferase reaction incorporating a biotinylated dideoxynucleotide. Fragmented end-labeled cDNA was hybridized to the GeneChip® Human Gene 2.0 ST arrays for 17 h at 45℃ and 60 rpm as described in the Gene Chip Whole Transcript (WT) Sense Target Labeling Assay Manual (Affymetrix). After hybridization, the chips were stained and washed in a Genechip Fluidics Station 450 (Affymetrix) and scanned by using a Genechip Array scanner 3000 7G (Affymetrix). The expression intensity data were extracted from the scanned images using Affymetrix Command Console software version 1.1 (Affymetrix, Santa Clara, CA, USA) and stored as CEL files.
The intensity values of CEL files were normalized to remove bias between the arrays (M1), using the Robust Multi-array Average (RMA) algorithm implemented in the Affymetrix Expression Console software (version 1.3.1; http://www.affymetrix.com). The whole normalized data were imported into the programming environment R (version 3.0.2; Affymetrix, Santa Clara, CA, USA) and overall signal distributions of each array were compared by plotting using tools available from the Bioconductor Project (http://www.bioconductor.org) (M2) to check good normalization. After confirming whether the data were properly normalized, differentially expressed genes (DEGs), that showed over 2-fold difference between the average signal values of the control groups and treatment groups, were selected in manual. In addition, the normalized data of selected DEGs were also imported into the programming environment R for the statistical t-test. Genes with p-value less than 0.05 were extracted as significant DEGs for further study (M2). In order to classify the co-expression gene groups which have similar expression patterns, hierarchical clustering analysis was performed with the Multi Experiment Viewer (MEV) software version 4.4 (http://www.tm4.org) (M3). Finally, using the web-based tool the Database for Annotation, Visualization, and Integrated Discovery (DAVID), DEGs were functionally annotated and classified based on the information of gene function such as OMIMDISEASE, GENE ONTOLOGY, KEGG PATHWAY, and BIOCARTA databases to reveal regulatory networks that are involved in DAVID Bioinformatics Resource 6.8 (https://david.ncifcrf.gov/) (M4).
A cancer stem-like cells were isolated from the tissue of a liver cancer patient, and its morphology was analyzed by an optical microscope (Fig. 1A). Similar to normal mesenchymal stem cell, the cancer stem-like cell had a spinal shape. To identify the cancer stem cell, CD133 was used as a marker to differentiate from adipose-derived stem cell, and cancer stem cell sorting was performed using a FACs. As shown in Fig. 1B, about 50% of the cancer stem cell population was isolated as compared to the control cells such as cancer cell lines. The expressions of Oct4, Nanog and SOX2, the stem cell markers, were measured by a FACs (Fig. 1C). Huh7, a HCC cell line, SUN478, a pancreatic cancer cell line, and mesenchymal stem cell were used as control samples to compare and measure the results of three different stem cell markers. Oct4, a potency marker, was expressed at very low levels in Huh7 and SNU478 cells, while Nanog involved in Oct4 expression and proliferation, and Sox2 which has multipotency properties were highly expressed in cancer stem cells. These findings showed that isolated cancer stem cells have a similar pattern to adipose derived stem cells, or mesenchymal stem cells (MSCs), indicating that isolated cancer stem cells have similar characteristics to MSCs, unlike cancer cell line. Finally, to identify the cancer stem cells, immunocytochemistry assay was used to confirm the expression of surface markers of cancer stem cells (Fig. 1D). The expressions of CD105, a common marker of mesenchymal stem cells (MSCs), CD133, a marker of CD90 expression and cancer stem cells, and beta-catenin, a self-renewal related marker, were identified. CD13, known as a therapeutic-specific marker of hepatic cancer stem cells, was clearly expressed. This indicated that cancer stem cells, isolated from the tissue of liver cancer, have the property of stem cells, not tumors.
With the identification of the spheroid formation derived from self-assembly of cancer stem-like cells, the properties of these cells were analyzed (Fig. 2A). High expression of CD73, a positive marker of mesenchymal stem cells, was identified in spheroid-formed cancer stem cells, while CD45, or lymphocytic antigen, known as a negative marker of MSCs, was weakly positive (Fig. 2B). As these cells were isolated from patient liver cancer tissue, it suggests that the immune response occurs in the inflammatory tissue around liver cancer. To confirm epithelialmesenchymal transition (EMT), which is one of the properties of cancer stem cells, the expression of E-cadherin and vimentin, an EMT-related marker, was analyzed. Fig. 2C shows high expressions of E-cadherin, an epithelial marker, and vimentin, a mesenchymal linage marker, verifying the property of cancer stem cell, which is thought to be the transition stage of cells. The expression of angiogenesis marker, another property of cancer stem cells, was also identified (Fig. 2D). The expressions of VEGF and CD34 in spheroid-formed cells proved the property of angiogenesis. It could, therefore, be assumed that these expressions are relevant to the tumor vasculature in the stage of tumorigenesis.
Cancer stem-like cells which have the property of cancer stem cells as the cause of liver cancer were incubated under hypoxia and normoxia for 24 h, and a microarray analysis was performed using the mRNA of cells. A hypoxic stimulus on cancer stem cells resulted in variable genetic alterations in angiogenesis, metabolism, and apoptosis. Of 26000 human genes, alterations were identified in 412 genes. Among them, 219 genes reacted specifically under hypoxia, 207 showed increased gene expression, and 12 showed decrease gene expression (Fig. 3A). Of the genes involved in cell formation, genes which are related to a cell itself or cell organelle accounted for 70%, and genes which induce catalytic function accounted for 42% (Fig. 3B). It is of an interest to note that membrane interacting with extracellular signals or genes involved in binding activity accounted for 31%, which was the second largest genetic change following the catalytic activity. It is highly likely that cancer stem-like cells are heavily involved in external signals as well as signal releasing by active proliferation and development of cancer cells. It is noted in the figure that genes involved in biological processes such as angiogenesis, apoptosis, and metabolism showed little change: variable genetic activation and changes making up 3% to 22%.
Among the verified 219 genes, 26 genes were the target of genetic screening, excluding well-known genes from the perspective of principle and function or genes with low expression. Twenty six genes which were subject to screening showed genetic changes more than twice times. The result, however, was not significantly different from the group affected by a hypoxia stimulus, which was provided by an inverter every 24 hours, twice per day (data not shown). To identify changes in 26 genes of cancer stem-like cells under hypoxia, a real-time qPCR was performed. As shown in Fig. 4, in comparison with normoxic condition, gene expression increased by more than two times. Angiogenesis-related genes and the genes involved in cell signals and structure were highly expressed, while genes involved in metabolism and cell growth showed little expression. NDRG1 associated cell proliferation epithelial and endothelial cells, ALDOC and PDK1 are key enzymes in glycolysis, bHLH transcription factors such as c-Myc and HIF-1 which are important in development or cell activity, have been associated to cancer because of their effects on cell growth and metabolism. Finally, Pim-1 is a proto-oncogene which encodes for the serine/threonine kinase. Pim-1 is involved primarily in cytokine signaling and initiated by STAT3 and STAT5, and its production is regulated by the cytokines that regulate the STAT pathway. Also, as expected, microarray analysis revealed that the expressions of ERCCGL and ERRFI1, were three times more than under normoxia condition, however, real time qPCR showed no difference in the expressions or slightly different within an error range. Under the condition of hypoxia, the over-expression of gene related with metabolism produces energy for rapid growth of tumor, and the over-expression of gene related with angiogenesis provides not only energy, but metastasis of tumor to other tissues. Therefore, the tumor grows by the metabolism-related genes such as ALDOC, PDK1. At the same condition, VEGFA induces angiogenesis, and PIM makes tumor cell signal increase through the growth of CSC. These findings suggest that hypoxic condition would make culture cells much harder than normoxia condition.
Advanced HCC develop hypoxic induction of angiogenesis and growth,5 which become cancerous.1 Many patients can completely recover from liver cancer after TACE,424 chemoradiotherapy or surgery,4 but are at risk of recurrence. In that case, liver cancer is highly likely to become malignant because many stem cells involved in liver regeneration are exposed to cancer tumorigenicity,16 or are transformed into cancer stem cells or cancer stem-like cells due to genetic changes.1022 When a stem cell converts into a cancer stem cell or cancer stem-like cell, it can develop into a tumor which has a poor prognosis,18 exacerbated by the addition of stem cell proliferation rate to tumor survival mechanism.22
It is known that cancer stem cells, which are mesenchymal cells in the cancer tissue,1022 have a great influence on a patient's poor prognosis.11 For that reason, therefore, studies have been carried out for the development of anti-cancer drug to maximize therapeutic effects, targeting cancer stem cells.182226 Nevertheless, there remain numerous difficulties in anti-cancer drug development targeting genetic characterization and cancer stem cells,1727 as cancer stem cells originate from cancer tissue or are created by genetic alteration of mesenchymal stem cells. Also, as for the tumor mass growth affected by tumorigenesis, there are differences in cell signaling because oxygen in inner space and outer surface within the tumor mass varies, and local environmental condition of nutrients is also different.21 As a result, a single tumor mass could have a heterotypic phenotype.10 Therefore, genetic exchange should be identified based on characterization of cancer stem cells under different conditions;19 results of genetic exchange of cancer stem cells under hypoxia and normoxia have significant implications.415 Microarray datum in the present study indicated that catalysis-related gene exchange of cancer stem cells, which are exposed to hypoxic condition, is considered to be a natural change that increases the survival probability of cells through cell-cell interaction. Furthermore, the present results showed that angiogenesis and apoptosis-related gene exchanges in vivo tumor growth are similar to those of cancer cells in the necrotic zone of inner space which is exposed to hypoxic condition. It is highly possible that cells in the necrotic zone undergo change, relying on vasculature in the tumor mass for supplying oxygen and nutrients.
In the stage of tumorigenesis, tumor vasculature has a great influence on growth and metastasis.1 In vivo tumorigenesis, cancer cells under hypoxic condition secrete angiogenic factors, so that endothelial cells' sprouting derives from neighboring blood vessels, and abnormal vascularization of a tumor takes place.28 Abnormal blood vessels formed by this procedure facilitate metastasis also, taking cancer cells to other sites.6 Cancer cells or cancer stem cells under hypoxic condition undergo changes in structure, and increase growth and metastasis through epithelial mesenchymal transition (EMT) or mesenchymal epithelial transition (MET).8929 The present finding showed that the increasing expression of angiogenesis- and structure-related genes under hypoxia is similar to patterns of tumorigenesis described in existing theories.
Considering different types of genetic data in this research, more studies are needed to examine how genetic change in vitro or in vivo under hypoxia affects cancer stem cells, also paying more attention to the importance of unknown genes, rather than already-known genes. Finally, the genetic data in the present research are expected to help us understand the causes as well as governing principles of tumor growth under hypoxia, and can be exploited as background knowledge for developing relevant treatments.
Figures and Tables
Fig. 1
Identification of hepatocellular carcinoma (HCC) derived cancer stem-like cells. (A) Microscopic image of cancer stem-like cells isolated from the cancer tissue of a HCC patient, showing spinal shape of stem cell morphology. (B) For characterization of cancer stem cells (CSCs); a positive portion of CD133 expression, which functions as a marker to differentiate from adipose derived stem cells, was analyzed using flow cytometry. (C) Oct4, Nanog, and Sox2 expression portion, the stem cell marker of isolated CSCs, were measured using flow cytometry. HCC cell line (Huh7), pancreatic cancer cell line (SNU478) and mesenchymal stem cells were compared and analyzed. Oct4, a potency marker, was expressed at very low levels in Huh7 and SNU478, while Nanog which is involved in Oct4 expression and proliferation, and Sox2 which has a multipotency property were highly expressed in isolated CSCs. (D) To identify the expression of surface markers in CSCs, immunocytochemistry assay was used. CD105 and CD90 which are the common MSCs marker, CD133 which is a marker of CSCs, and beta-catenin which is a self-renewal related marker were expressed. CD13, which is known as a therapeutic-specific marker in hepatic CSCs, was also clearly expressed. Scale bar=200 µm.
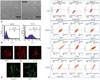
Fig. 2
Characterization of hepatocellular carcinoma-derived cancer stem-like cells. (A) Spheroid formation derived from self-assembly of cancer stem cells. (B) High expression of CD73 as a positive marker of mesenchymal stem cells in spheroid formed cancer stem cells (×100). (C) The expression of E-cadherin and vimentin, the related marker of Epithelial-mesenchymal transition, was identified (×100). (D) The expression of an angiogenesis marker influenced by cancer stem cells was identified. The expressions of vascular endothelial growth factor (VEGF) and CD34 were identified in spheroid formed cells (×100). LM, light microscopy.
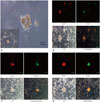
Fig. 3
Gene profiling of hypoxia-stimulated cancer stem-like cells by microarray analysis. The changes in gene expression were compared between hypoxia and normoxia. (A) The alteration of gene expression was measured in 419 genes of 26000 human genes. The alteration was also measured in 237 genes under hypoxia. (B) The function of altered genes was classified and analyzed according to cellular component, molecular function, biological process, and panther-chart pathway. Variable gene expressions were identified. In particular, genes involved in cell signaling and cell formation were significantly altered.
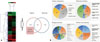
Fig. 4
Gene validation of hypoxia-stimulated cancer stem-like cells. Each gene expression was identified through a real-time qPCR. Most gene expressions were found to increase two to ten times over those under hypoxia. The expressions of NDRG1, ER01L, ALDOC, GNRH, ADM, CSPR2, BHLH, and PIM1 increased more than five times, and the expression of HIG2 increased more than 20 times. In particular, genes involved in cell signaling and cell formation showed an increase in their expression.
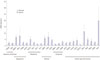
ACKNOWLEDGEMENTS
This study was supported by a faculty research grant of Yonsei University College of Medicine for 2012 (6-2012-0008).
References
2. Ferenci P, Fried M, Labrecque D, Bruix J, Sherman M, Omata M, et al. World Gastroenterology Organisation Guideline. Hepatocellular carcinoma (HCC): a global perspective. J Gastrointestin Liver Dis. 2010; 19:311–317.
3. Harris AL. Hypoxia--a key regulatory factor in tumour growth. Nat Rev Cancer. 2002; 2:38–47.
4. Samanta D, Gilkes DM, Chaturvedi P, Xiang L, Semenza GL. Hypoxia-inducible factors are required for chemotherapy resistance of breast cancer stem cells. Proc Natl Acad Sci U S A. 2014; 111:E5429–E5438.


5. Sun HC, Tang ZY. Angiogenesis in hepatocellular carcinoma: the retrospectives and perspectives. J Cancer Res Clin Oncol. 2004; 130:307–319.


6. Hickey MM, Simon MC. Regulation of angiogenesis by hypoxia and hypoxia-inducible factors. Curr Top Dev Biol. 2006; 76:217–257.


7. Kitajima Y, Miyazaki K. The critical impact of HIF-1a on gastric cancer biology. Cancers (Basel). 2013; 5:15–26.


8. Otrock ZK, Hatoum HA, Awada AH, Ishak RS, Shamseddine AI. Hypoxia-inducible factor in cancer angiogenesis: structure, regulation and clinical perspectives. Crit Rev Oncol Hematol. 2009; 70:93–102.


9. Pugh CW, Ratcliffe PJ. Regulation of angiogenesis by hypoxia: role of the HIF system. Nat Med. 2003; 9:677–684.


10. Yagi H, Kitagawa Y. The role of mesenchymal stem cells in cancer development. Front Genet. 2013; 4:261.


11. Krutovskikh V, Partensky C. [New insights in oncology: epigenetics and cancer stem cells]. Cancer Radiother. 2011; 15:716–722.
12. Brooks DL, Schwab LP, Krutilina R, Parke DN, Sethuraman A, Hoogewijs D, et al. ITGA6 is directly regulated by hypoxia-inducible factors and enriches for cancer stem cell activity and invasion in metastatic breast cancer models. Mol Cancer. 2016; 15:26.


13. Heddleston JM, Li Z, Lathia JD, Bao S, Hjelmeland AB, Rich JN. Hypoxia inducible factors in cancer stem cells. Br J Cancer. 2010; 102:789–795.


14. Ji N, Yu JW, Ni XC, Wu JG, Wang SL, Jiang BJ. Bone marrow-derived mesenchymal stem cells increase drug resistance in CD133-expressing gastric cancer cells by regulating the PI3K/AKT pathway. Tumour Biol. 2016; 37:14637–14651.


15. Yeung TM, Gandhi SC, Bodmer WF. Hypoxia and lineage specification of cell line-derived colorectal cancer stem cells. Proc Natl Acad Sci U S A. 2011; 108:4382–4387.


16. Yamashita T, Kaneko S. Orchestration of hepatocellular carcinoma development by diverse liver cancer stem cells. J Gastroenterol. 2014; 49:1105–1110.


17. SchÖning JP, Monteiro M, Gu W. Drug resistance and cancer stem cells: the shared but distinct roles of hypoxia-inducible factors HIF1α and HIF2α. Clin Exp Pharmacol Physiol. 2017; 44:153–161.


18. Klonisch T, Wiechec E, Hombach-Klonisch S, Ande SR, Wesselborg S, Schulze-Osthoff K, et al. Cancer stem cell markers in common cancers-therapeutic implications. Trends Mol Med. 2008; 14:450–460.
19. Yamashita T, Wang XW. Cancer stem cells in the development of liver cancer. J Clin Invest. 2013; 123:1911–1918.


20. Mathonnet M, Perraud A, Christou N, Akil H, Melin C, Battu S, et al. Hallmarks in colorectal cancer: angiogenesis and cancer stemlike cells. World J Gastroenterol. 2014; 20:4189–4196.


21. Pasquier J, Rafii A. Role of the microenvironment in ovarian cancer stem cell maintenance. Biomed Res Int. 2013; 2013:630782.


22. Bao B, Ahmad A, Li Y, Azmi AS, Ali S, Banerjee S, et al. Targeting CSCs within the tumor microenvironment for cancer therapy: a potential role of mesenchymal stem cells. Expert Opin Ther Targets. 2012; 16:1041–1054.


24. Gadaleta CD, Ranieri G. Trans-arterial chemoembolization as a therapy for liver tumours: new clinical developments and suggestions for combination with angiogenesis inhibitors. Crit Rev Oncol Hematol. 2011; 80:40–53.


25. Piao LS, Hur W, Kim TK, Hong SW, Kim SW, Choi JE, et al. CD133+ liver cancer stem cells modulate radioresistance in human hepatocellular carcinoma. Cancer Lett. 2012; 315:129–137.


26. Rosa R, D'Amato V, De Placido S, Bianco R. Approaches for targeting cancer stem cells drug resistance. Expert Opin Drug Discov. 2016; 11:1201–1212.


27. Leake I. Colorectal cancer: targeting self-renewal of cancer stem cells in colorectal cancer-a future treatment option? Nat Rev Gastroenterol Hepatol. 2014; 11:75.

