Abstract
Purpose
Pulmonary surfactants for preterm infants contain mostly animal-derived surfactant proteins (SPs), which are essential for lowering surface tension. We prepared artificial pulmonary surfactants using synthetic human SP analogs and performed in vitro and in vivo experiments.
Materials and Methods
We synthesized peptide analogues that resemble human SP-B (RMLPQLVCRLVLRCSMD) and SP-C (CPVHLKRLLLLLLLLLLLLLLLL). Dipalmitoylphosphatidylcholine (DPPC), phosphatidylglycerol (PG), and palmitic acid (PA) were added and mixed in lyophilized to render powdered surfactant. Synsurf-1 was composed of DPPC:PG:PA:SP-B (75:25:10:3, w/w); Synsurf-2 was composed of DPPC:PG:PA:SP-C (75:25:10:3, w/w); and Synsurf-3 was composed of DPPC:PG:PA:SP-B:SP-C (75:25:10:3:3, w/w). We performed in vitro study to compare the physical characteristics using pulsating bubble surfactometer and modified Wilhelmy balance test. Surface spreading and adsorption test of the surfactant preparations were measured. In vivo test was performed using term and preterm rabbit pups. Pressure-volume curves were generated during the deflation phase. Histologic findings were examined.
Results
Pulsating bubble surfactometer readings revealed following minimum and maximum surface tension (mN/m) at 5 minutes: Surfacten® (5.5±0.4, 32.8±1.6), Synsurf-1 (16.7±0.6, 28.7±1.5), Synsurf-2 (7.9±1.0, 33.1±1.6), and Synsurf-3 (7.1±0.8, 34.5±1.0). Surface spreading rates were as follows: Surfacten® (27 mN/m), Synsurf-1 (43 mN/m), Synsurf-2 (27 mN/m), and Synsurf-3 (27 mN/m). Surface adsorption rate results were as follows: Surfacten® (28 mN/m), Synsurf-1 (35 mN/m), Synsurf-2 (29 mN/m), and Synsurf-3 (27 mN/m). The deflation curves were best for Synsurf-3; those for Synsurf-2 were better than those for Surfacten®. Synsurf-1 was the worst surfactant preparation. Microscopic examination showed the largest aerated area of the alveoli in the Synsurf-3 group, followed by Synsurf-1 and Surfacten®; Synsurf-2 was the smallest.
Respiratory distress syndrome in preterm infants is caused by a lack of pulmonary surfactant that results in respiratory failure immediately after birth, with various comorbidities in preterm infants.1 Lack of surfactant leads to a failure to lower surface tension (ST) followed by alveolar collapse.2 The first clinical trial that applied artificial surfactant to preterm infants was performed by Fujiwara, et al.3 who selected bovine surfactant mixed with phospholipids. For a couple of decades thereafter, various commercial agents were developed, and many randomized controlled trials confirmed the use of surfactants as a standard treatment of choice.4 Such practice have enabled markedly improved survival in preterm infants.5
Artificial surfactants can be categorized into two different compositional features according to type of surfactant proteins (SPs). First are animal derived SPs. These SPs are minced or extracted from lung tissue from pigs or cows. Most of the currently used commercial products contain animal derived SPs. The second type of surfactants contains synthetic peptides. Only two synthetic products have been approved commercially, but mostly are inferior to animal SP containing surfactants.6789
Of four types of SPs, SP-B and SP-C are known to be physically active. Complete synthesis of full length human SP-B is impossible, because it is a very heavy molecule and complex in structure. In particular, its disulfide bond cannot be stably synthesized. The structure of fully synthesized human SP-C is extremely unstable.10 Therefore, based on this problem, analogues of these SPs may be of better use, instead of the whole sequence peptides. Accordingly, we chose two analogue peptide sequences for SP-B and SP-C from previous research and aimed to certify the efficacy of each individually or a mixture thereof.
We chose part of the C-terminal sequence of human SP-B as follows: RMLPQLVCRLVLRCSMD. For SP-C analogue, we chose the following: CPVHLKRLLLLLLLLLLLLLLLL. Those peptides were synthesized via Anygen Co., Ltd., Jangseong-gun, Korea. Dipalmitoylphosphatidylcholine (DPPC), phosphatidylglycerol (PG), and palmitic acid (PA) were prepared by Sigma-Altrich Co. (St. Louis, MO, USA). Synthetic surfactant Synsurf-1 was made by a mixture of DPPC:PG:PA:SP-B=75:25:10:3 (w/w). Synsurf-2 comprised DPPC:PG:PA:SP-C=75:25:10:3 (w/w) and Synsurf-3 DPPC:PG:PA:SP-B:SP-C=75:25:10:3:3 (w/w).
Three kinds of SP compounds were dissolved in a small amount of trifluoroacetic acid and mixed with DPPD:PD:PA (75:25:10, w/w) in CHCL3:CH3OH (2:1, v/v). Further melting down was accelerated with 10% ethanol solution and evaporated at 40–45℃ temperature over 15 minutes. Finally, lyophilization was performed to render Synsurf-1, -2, and -3 as powders.
The physical surface properties were compared between our newly synthesized surfactants and one commercial product, Surfacten® (Mitsubishi-Tokyo Pharma Corporation, Osaka, Japan), which contains bovine protein as SPs. To measure ST, we used pulsating bubble surfactometer (PBS; Electronetics Corporation, CT, USA) and modified Wilhelmy balance test (Acoma, Tokyo, Japan). The dissolution condition of all the preparations were the same at 30 mg/mL diluted in normal saline. PBS renders the minimum-ST (min-ST) and maximum-ST (max-ST). The ideal artificial surfactant should be less than 10 mN/m as min-ST and over 30 mN/m as max-ST. By using modified Wilhelmy balance equipment, we can obtain ST-area diagram (hysteresis curve), surface spreading rate, and surface adsorption rate. We used ideal surfactant criteria as stated by Robertson and van Golde11 (Table 1).
A New Zealand white rabbit model was used. C-section was done to harvest preterm pups on G27 and term pups on G31 from mother rabbits. Soon after delivery, we performed tracheostomy and gentle forced ventilation of 0.3–0.5 cc air with a syringe over 30 cycles. Study groups were as follows: 1) preterm pups control (preterm control), 2) term pups control (term control), 3) preterm pups with Surfacten® administration (Surfacten® group), 4) preterm pups with Synsurf-1 administration (Synsurf-1 group, SP-B), 5) preterm pups with Synsurf-2 administration (Synsurf-2 group, SP-C), and 6) preterm pups with Synsurf-3 administration (Synsurf-3 group, SP-B&C).
Dosage was 100 mg/kg in all treatment groups (Surfacten®, Synsurf-1, -2, and -3). In all six groups, we plotted pressure-volume curves in the deflation phase. The measured volume was calculated per body weight. Pathologic findings were also analyzed. Soon after the pressure-volume curve test, we blocked the tracheostomy at 10 cm H2O and fixed in formalin solution. Both left and right lung tissue samples were H-E stained to observe histologic findings. The ×100 magnified images were analyzed for aerated area ratio via ImageJ program (V 1.48 for Windows, NIH, Bethesda, MD, USA). Two sites from each of the right and left lungs were used. Animal Institutional Review Board approved this study.
Forty-seven preterm pups and forty-one term pups from 17 mother rabbits were included in this study. The preterm pups' total birth weight was 32.0±4.5 g and term pups' total birth weight was 45.1±13.0 g (Table 2). Min-ST and max-ST as measured by PBS are described in Table 3. The ideal artificial surfactant should show less than 10 nM/m of min-ST and higher than 30 nM/m of max-ST. Surfacten®, Synsurf-2, and Synsurf-3 fulfilled these criteria. Modified Wilhelmy balance test rendered surface spreading rate, surface adsorption rate, and ST area diagrams (Fig. 1). In this test, Surfecten®, Synsurf-2, and Synsurf-3 showed active ST lowering effect. Table 4 outlines the measured physical properties. The physical properties of the four different surfactant preparations are listed in Table 3 and 4. According to Fujiwara's ideal artificial surfactant criteria (Table 1), Synsurf-1 showed poor performance in both PBS and modified Wilhelmy balance tests; however, Synsurf-2 and Synsurf-3 fulfilled those criteria. Pressure-volume curve analyses were expressed in the deflation phase, which implied the deflation phase among the hysteresis curve (Fig. 2). Similar to the Wilhelmy analyses curve results, Synsurf-1 was worst, and Synsurf-2 and Synsurf-3 were excellent. These findings were compatible with the histologic findings (Figs. 3 and 4).
Human pulmonary surfactant consists of 86% phospholipids, 8% neutral lipids, and 6% SP. Although SPs are only 6% of the composition, they are essential elements for lowering ST. Since artificial surfactant replacement therapy was introduced clinically, mortality in premature infants has remarkably improved and extremely premature survival has also been maximized. In the history of artificial surfactant development, early commercial synthetic surfactants included Exosurf® (GlaxoSmithKline, Brentford, UK) and Surfaxin® (Discovery Laboratories, Warrington, PA, USA). Exosurf® contains DPPC only without SP, while Surfaxin® has the synthetic peptide KL4 as an analogue of human SP-B.12 However, animal derived (bovine and porcine) natural SP has been found to be clinically superior to synthetic surfactant. In a Cochrane review, animal natural surfactant was recommend for better safety and outcomes.13 However, many researchers are still trying to find a fully synthetic surfactant, rather than a natural one, which can potentially have hazardous proteins, such as prion fibrils, and requires mass slaughtering of pigs or cows, causing ethical problems and cost benefits.
There are four types of human SPs, which are SP-A, SP-B, SP-C, and SP-D. Two hydrophilic proteins, SP-A and SP-D, involve surfactant synthesis and release, anti-inflammatory action, and immunologic response. SP-B and SP-C are very hydrophobic and amphipathic, which confer direct mechanical or physical properties in the mixture of surfactant.14 It is impossible to reconstruct intact human SPs based on the known full sequences, because of their instability and complicated structure.
We selected SP-C analogue sequences (CPVHLKRLLLLLLLLLLLLLLLL) from Otsubo, et al.15 and Bae, et al.16 study. Otsubo, et al.15 and Otsubo and Takei17 named it as SP-CL16 according to its poly Leucine sequence. The authors demonstrated good surface activity for SP-CL16 as a SP-C analogue in 2002. However, subsequent validation of its surface properties was not performed until the study of Bae, et al.16 in 2015, which showed stable reproducibility. SP-CL16 has poly-Leucine residues, unlike human SP-C, which has poly-Valine residues. This property confers more stable amphipathic features. Furthermore, there is another advantage of having poly-Leucine. If an α-helical structure of SP-C is abnormally unfolded and changes to a β-sheet structure, it undergoes fibril formation of lung surfactant, which is similar as an abundance of amyloid fibrils composed of abnormal SP-C in pulmonary alveolar proteinosis. However, the poly-Leu sequence of synthetic SP-C has more stable α-structure than poly-Val sequence.18
Unlike the previous SP-CL16 research, we intended to add a SP-B counterpart in our pulmonary surfactant. There are studies that emphasize including both SP-B and SP-C in synthetic surfactants. Even though SP-B is not structurally perfect to human one, it seems that SP-B is necessary.1920 Selecting the sequence as a SP-B analogue, we chose RMLPQLVCRLVLRCSM D, which was studied by Bae, et al.21 It does not contain the full sequence, but 17 residues of the C-terminal. It is simple, short, and very stable when synthesized, and it is easy to produce. The authors concluded that the sequence had possible potential as an artificial synthetic SP-B analogue that can be mixed with DPPC and PG. Normally, functional human SP-B, which consists of 79 residue peptides, has its characteristic disulfide bond rendering V-shape. It is not clear whether the SP-B analogue in this study has disulfide bond structure in itself, although its addition in Synsurf-3 brought better outcomes than containing SP-C analogue alone as PSs in aerated area ratio. The literature on SP-B defect revealed severe pulmonary diseases, such as congenital alveolar proteinosis.22 This might explain how our SP-B analogue acted as certain human SP-B mimicking function in Synsurf-3.
Our study showed good results of synthetic SP analogue containing surfactants. The physical surface properties measured through PBS and modified Wilhelmy surface balance test were ideal for Synsurf-2 and -3. These physical activity measurements are very essential to validate pulmonary surfactant effects. Although we had limitations using very small rabbit fetuses, our deflation curves and histologic findings were comparable between Synsurf-2, -3, and Surfacten®. When we plotted the pressure-volume curve, especially in the deflation phase, we found that there was a significant difference in volume at the pressure of 15 cm H2O (Fig. 2). Statistical analysis was conducted at 15 cm H2O; however, we also noted a difference at 0 cm H2O as well. The difference did not come from lung tissue selection bias, but from pre-treatment with the different preparations. This reflects the different functional residual capacities that the four different surfactants resulted in. It is noteworthy that Synsurf-1 was the worst. This means that SP-B alone cannot generate ST lowering effect in artificial surfactant. Nevertheless, synthetic SP-B (RMLPQLVCRLVLRCSMD) and SP-C (CPVHLKRLLLLLLLLL LLLLLLL) provided the best synthetic surfactant. Limitations of the present study include that we could not evaluate the efficacy of our Synsurfs in comparison to other available commercial preparations, for example Curosurf® (Chiesi Farmaceutici, Parma, Italy) (porcine SP) or Surfaxin® (synthetic SP). Further study should be performed to conform reproducibility of the same results in a larger sample size.
References
1. Jobe AH, Hillman N, Polglase G, Kramer BW, Kallapur S, Pillow J. Injury and inflammation from resuscitation of the preterm infant. Neonatology. 2008; 94:190–196. PMID: 18832854.


2. Miedema M, de Jongh FH, Frerichs I, van Veenendaal MB, van Kaam AH. Changes in lung volume and ventilation during surfactant treatment in ventilated preterm infants. Am J Respir Crit Care Med. 2011; 184:100–105. PMID: 21493733.


3. Fujiwara T, Maeta H, Chida S, Morita T, Watabe Y, Abe T. Artificial surfactant therapy in hyaline-membrane disease. Lancet. 1980; 1:55–59. PMID: 6101413.


4. Soll R, Ozek E. Multiple versus single doses of exogenous surfactant for the prevention or treatment of neonatal respiratory distress syndrome. Cochrane Database Syst Rev. 2009; (1):CD000141. PMID: 19160177.


5. Kim SM, Park YJ, Chung SH, Choi YS, Kim CH, Bae CW. Early prophylactic versus late selective use of surfactant for respiratory distress syndrome in very preterm infants: a collaborative study of 53 multi-center trials in Korea. J Korean Med Sci. 2014; 29:1126–1131. PMID: 25120324.


6. Zhu Y, Miller TL, Chidekel A, Shaffer TH. KL4-surfactant (Lucinactant) protects human airway epithelium from hyperoxia. Pediatr Res. 2008; 64:154–158. PMID: 18391844.


7. Moya F, Sinha S, Gadzinowski J, D'Agostino R, Segal R, Guardia C, et al. One-year follow-up of very preterm infants who received lucinactant for prevention of respiratory distress syndrome: results from 2 multicenter randomized, controlled trials. Pediatrics. 2007; 119:e1361–e1370. PMID: 17533176.


8. Lu KW, Taeusch HW. Combined effects of polymers and KL(4) peptide on surface activity of pulmonary surfactant lipids. Biochim Biophys Acta. 2010; 1798:1129–1134. PMID: 20188696.


9. Soll RF, Blanco F. Natural surfactant extract versus synthetic surfactant for neonatal respiratory distress syndrome. Cochrane Database Syst Rev. 2001; (2):CD000144. PMID: 11405951.


10. Kallberg Y, Gustafsson M, Persson B, Thyberg J, Johansson J. Prediction of amyloid fibril-forming proteins. J Biol Chem. 2001; 276:12945–12950. PMID: 11134035.


11. Robertson B, van Golde LMG. Pulmonary surfactant: from molecular biology to clinical practice. Amsterdam: Elsevier Science Publishers;1992. p. 561–592.
12. Moya F. Synthetic surfactants: where are we? Evidence from randomized, controlled clinical trials. J Perinatol. 2009; 29(Suppl 2):S23–S28. PMID: 19399006.


13. Seger N, Soll R. Animal derived surfactant extract for treatment of respiratory distress syndrome. Cochrane Database Syst Rev. 2009; (2):CD007836. PMID: 19370695.


14. Walther FJ, Waring AJ, Sherman MA, Zasadzinski JA, Gordon LM. Hydrophobic surfactant proteins and their analogues. Neonatology. 2007; 91:303–310. PMID: 17575474.


15. Otsubo E, Takei T, Nomura M. Synthesis, purification and surface activities of the human pulmonary surfactant protein-C (SP-C) analogue, SP-CL16 (6-28). Biol Pharm Bull. 2001; 24:1362–1365. PMID: 11767102.
16. Bae CW, Chung SH, Choi YS. Development of a synthetic surfactant using a surfactant protein-C peptide analog: in vitro studies of surface physical properties. Yonsei Med J. 2016; 57:203–208. PMID: 26632402.


17. Otsubo E, Takei T. Effects of the human pulmonary surfactant protein-C (SP-C), SP-CL16 (6-28) on surface activities of surfactants with various phospholipids. Biol Pharm Bull. 2002; 25:1303–1306. PMID: 12392083.
18. Johansson J. Membrane properties and amyloid fibril formation of lung surfactant protein C. Biochem Soc Trans. 2001; 29(Pt 4):601–606. PMID: 11498036.


19. Almlén A, Walther FJ, Waring AJ, Robertson B, Johansson J, Curstedt T. Synthetic surfactant based on analogues of SP-B and SP-C is superior to single-peptide surfactants in ventilated premature rabbits. Neonatology. 2010; 98:91–99. PMID: 20110733.


20. Seehase M, Collins JJ, Kuypers E, Jellema RK, Ophelders DR, Ospina OL, et al. New surfactant with SP-B and C analogs gives survival benefit after inactivation in preterm lambs. PLoS One. 2012; 7:e47631. PMID: 23091635.


21. Bae CW, Choi YM, Kang JH, Lee SC, Kim KL, Hahm KS. A study of surface physical properties of new surfactant using synthetic peptides of surfactant protein-B. Korean J Pediatr. 2000; 43:1059–1067.
22. Nogee LM, de Mello DE, Dehner LP, Colten HR. Brief report: deficiency of pulmonary surfactant protein B in congenital alveolar proteinosis. N Engl J Med. 1993; 328:406–410. PMID: 8421459.
Fig. 1
Diagrams generated by modified Wilhelmy balance test. (A) Surface spreading rate test. Synsurf-1 was slow. Synsurf-2 and -3 and Surfacten® were similarly fast in regards to surface spreading rate test. (B) Surface adsorption was best for Synsurf-3, followed by Surfacten®, Synsurf-2, and Synsurf-1 in that order. (C) In surface tension area diagram, all four preparations showed hysteresis curves. Among these, Surfacten® showed the lowest minimum-ST, which was the best. Synsurf-2 and -3 showed similarly in the middle and Synsurf-1 was the worst. ST, surface tension.
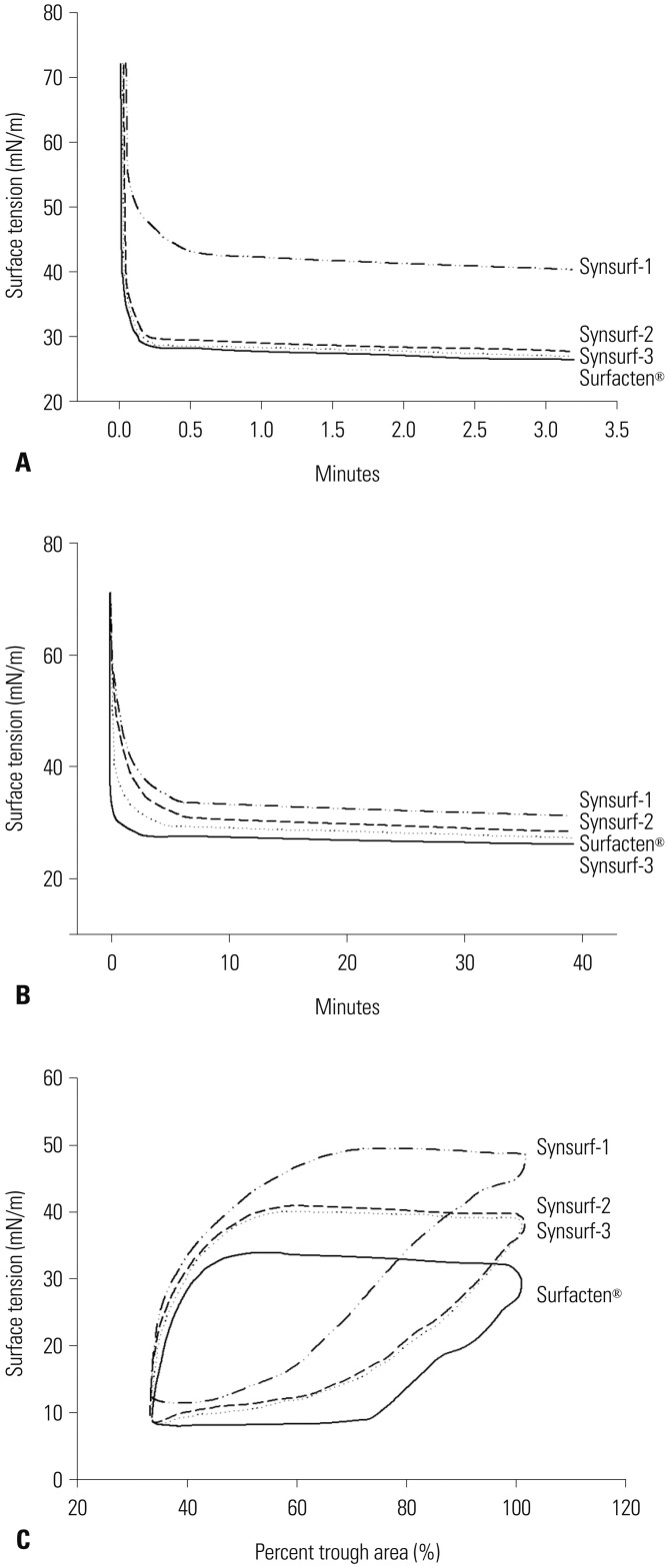
Fig. 2
Pressure volume curves. (A) shows the difference between term (n=9) and preterm controls (n=13, p<0.001). (B) shows deflation curves for the four different surfactant preparations. Synsurf-2 (n=7, p=0.0068), Synsurf-3 (n=6, p=0.0031), and Surfacten® (n=18, p<0.001) showed higher pressure volume curves in the term group than the preterm group; Synsurf-1 (n=6, p=0.4811) showed no difference. Mann Whitney U test was used to compare volumes at 15 cm H2O pressure.
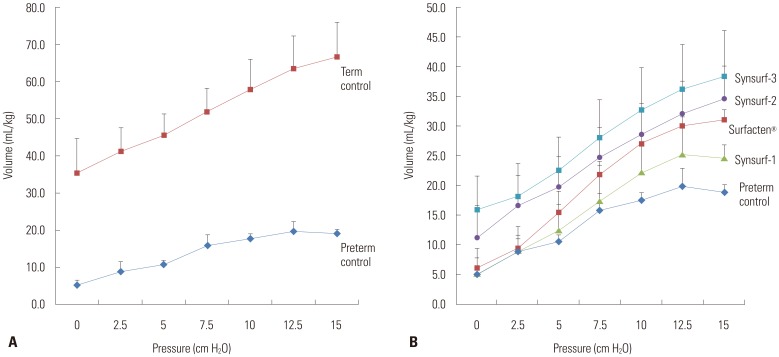
Fig. 3
Histologic differences among six groups (hematoxylin and eosin stain, ×100). Four surfactant preparations-treated lung tissues demonstrated better aerated features than preterm controls. For statistical analysis, alveolar areas were compared which and revealed significant differences, as shown in Fig. 4.
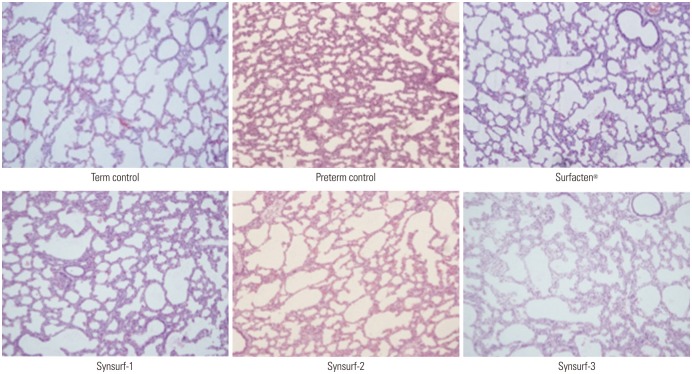
Fig. 4
Aerated area percent comparison. Tissues treated with Sufacten® (n=34), Synsurf-1 (n=24), Synsurf-2 (n=31), and Synsurf-3 (n=30), demonstrated significantly higher aerated area rate than controls (n=49, p<0.001). Synsurf-3 was significantly higher than Surfactant® (p=0.020), while Synsurf-1 and Synsurf-2 were not different from Surfactant ’ (p=0.729 and 0.722). Unpaired t-test was used.
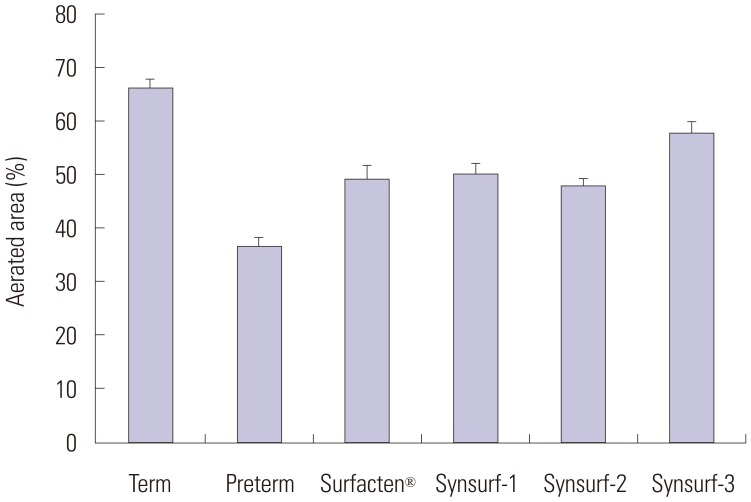
Table 1
In Vitro Criteria for Surface Properties of Ideal Artificial Pulmonary Surfactant
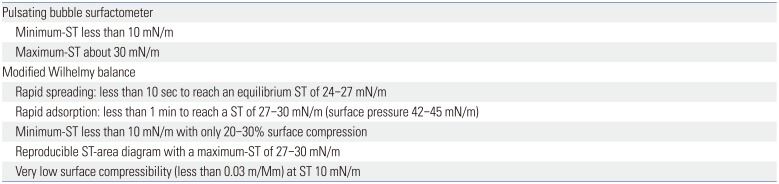
ST, surface tension.
Modified from Robertson, et al. Elsevier Science Publishers; 1992. p.561-92, with permission of Elsevier Science Publishers.11
Table 2
Birth Weight of Newborn Pups in Each Group

Preterm control | Term control | Preterm Surfacten® | Preterm Synsurf-1 | Preterm Synsurf-2 | Preterm Synsurf-3 | |
---|---|---|---|---|---|---|
n | 20 | 18 | 23 | 6 | 15 | 6 |
Birth weight (g) | 29.8±3.3 | 58.5±7.0 | 34.6±3.1 | 29.1±1.8 | 34.6±4.8 | 36.2±2.2 |
Table 3
Min-ST and Max-ST of Three Different Synsurfs Checked by Pulsating Bubble Surfactometer at 1 and 5 Minutes
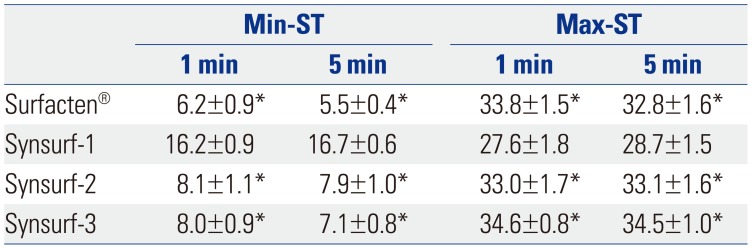
Table 4
Physical Properties of Surfacten and Synsurf Emulsions Measured by Modified Wilhelmy Balance Study
