Abstract
Purpose
Pulmonary arterial hypertension (PAH) is a fatal disease which is characterized by an increase in pulmonary arterial pressure leading to increases in right ventricular afterload. Human umbilical cord blood derived-mesenchymal stem cells (hUCB-MSCs) administered via the jugular vein have been previously shown to improve PAH by reversal treatment. However, the effect of low dosage and transfusion timing of hUCB-MSCs on PAH has not yet been clearly established. Obviously, low dosage treatment can lead to a reduction in costs. This is the first study on early transfusion effect.
Materials and Methods
This study was divided into two parts. The first part is an investigation of dose-dependent effect. hUCB-MSCs were administered into 3 groups of rats (UA: 3×106 cells, UB: 1.5×106 cells, UC: 3×105 cells) via the external jugular vein at week 1 after monocrotaline (MCT) injection. The second part is a search for optimal treatment timing in 3×105 cells dose of hUCB-MSCs administered at day 1 for UD group (low dose of hUCB-MSCs at day 1), at day 1 and week 1 for the UE group (dual transfusion of low dose of hUCB-MSCs at day 1 and week 1) and at 1 week for the UF group (reversal treatment of low dose hUCB-MSC at week 1) after MCT injection.
Results
The administration of 3×105 hUCB-MSCs was as effective as the 3×106 dose in decreasing mean right ventricle (RV) pressure and pulmonary pathological changes. Early treatment with hUCB-MSCs improved mean RV pressure, pulmonary pathological changes and heart collagen 3 protein expression levels in PAH.
Pulmonary arterial hypertension (PAH) is a life threatening disease, and is noted for an increase in pulmonary vascular resistance, right ventricular hypertrophy and pulmonary vessel remodeling.1 If it is not treated, it could lead to death. Inflammation and endothelial dysfunction play a significant role in the pathophysiological changes of PAH.234 PAH has been considered a refractory disease to most of the conventional pharmacological therapies.5 At present, alternative pharmacological treatment is being developed, nevertheless, mortality remains still too frequent.
Stem cell therapies have the potential to curesome pulmonary diseases.67 A number of studies demonstrated the efficacy of mesenchymal stem cell administration in many lung diseases including acute lung injury,8 bronchopulmonary dysplasia,910 chronic obstructive pulmonary disease,1112 fibrosing pulmonary injury,1314 pulmonary ischemia reperfusion injury,15 radiation induced lung injury,16 sepsis,17 and PAH.7918 Mesenchymal stem cells have an effect on lung inflammation and injury through paracrine interactions rather than direct interaction. 1920 The administration of human umbilical cord blood derived-mesenchymal stem cells (hUCB-MSCs) has recently been proposed for the treatment of PAH in animal models.21 hUCB-MSCs have advantages in that 1) they can be obtained relatively conveniently and less invasively,22 2) it is possible to differentiate into vascular cell types,23 and 3) they have an ability to secrete paracrine factors regulating inflammatory cytokines.24 We previously reported that hUCB-MSCs improved PAH via suppressing inflammatory cytokine level in lung tissues.6 These molecules have an immunosuppressive effect and lead to the regeneration of injured tissues by the way of paracrine mechanisms.25
In these earlier studies, diverse dosage, treatment timing and frequency were used, and the results of these studies showed variable rates of improvement. However, there is little information on the effects of a low-dose of and the administration timing of hUCB-MSCs, especially early timing. The purpose of this study was to examine the effect of low dose early treatment of hUCB-MSCs on PAH and compare it with the reversal therapy.
Study protocols were approved by the Institutional Animal Care and Use Committee of Ewha Womans University School of Medicine. Six-week old male Sprague-Dawley rats (180–220 g; Orient, Seongnam, Korea) were induced to PAH by subcutaneous injection of 60 mg/kg monocrotaline (MCT). We performed this study in two-parts (Fig. 1). The first part of the experiment was to determine the effective dose range because low-dose transplantation has an advantage from a clinical standpoint (Fig. 1A). We tested three different doses of hUCB-MSCs; 3×106 cells/rat (UA group), 1.5×106 cells/rat (UB group) and 3×105 cells/rat (UC group) in MCT-induced PAH rats. Thus, the hUCB-MSCs were diluted in 500 µL of Dulbecco's modified Eagle's medium and were directly administered into the external jugular vein of rats placed in a supine position. The transfusion of hUCB-MSCs was performed randomly .The second part of the experiment was to optimize the timing and frequency of hUCB-MSCs transplantation (Fig. 1B). The experimental groups were divided into three groups; UD group (early treatment group of low dose hUCB-MSCs at day 1), UE group (dual transfusion group of low dose hUCB-MSCs at day 1 and week 1), UF group (reversal treatment group of low dose hUCB-MSC at week 1).
hUCB-MSCs were obtained from Medipost Inc., and isolated human mesenchymal stem cells were expanded in culture according to the method previously described.6 The cells were positive for the cell surface markers such as CD73, CD90, and CD105, but negative for the hematopoietic cell-specific surface markers such asCD34, CD45, as well as for the major histocompatibility complex class II marker HLA-DR (Fig. 2).
After the mesenchymal cells were isolated from human umbilical cord blood, hUCB-MSCs were cultured at passage 4. The hUCB-MSCs were trypsinized and suspended in Dulbecco's modified Eagles medium at a concentration of 5×106 cells/mL. The cells were then incubated in various non-labeled primary antibodies, followed by fluorescein isothiocyanate-conjugated or phycoerythrin-conjugated immunoglobulin G antibodies. Subsequently, hUCB-MSCs were washed, fixed and analyzed.
Cultured cells were incubated in osteogenic, adipogenic or chondrogenic differentiation medium. The medium was changed every 2 days. After completion of differentiation, differentiation was confirmed by morphology and accumulation of alkaline phosphatase, oil red-O-staining or safranin-O-staining sulfated proteoglycans.
A hemodynamic study was performed at weeks 2 and 4 after MCT injection. The rats were anesthetized with intraperitoneal Zoletil (Virbac, Carros, France) and Rompun (Bayer Korea, Seoul, Korea). A catheter connecting a pressure electricity transducer was inserted in the external jugular vein and then advanced into the right ventricle (RV) for assessment of RV pressure and the carotid artery for arterial pressure.
Body weights were weighed and tissues were harvested. Rats were excised, and RV, left ventricle (LV)+septum (S), lung, kidney and liver were removed, and the tissues were then weighed. RV to LV+S ratio was used as an index of right ventricular hypertrophy.
For determination of pulmonary arteriole medial wall hypertrophy, thickness between the internal and external elastic lamina of two sides of arterioles was measured.6 The percentage of medial wall thickness was calculated as follows; % medial wall thickness=(medial wall thickness 1+medial wall thickness 2)/(vessel diameter)×100. For assessment of neomuscularization, the number of muscularized vessels was counted in a ×200 magnification field. Twenty or more fields were examined in each rat for analysis.
Protein expression levels of endothelin receptor A (ERA, SantaCruz biotechnology, Santacruz, CA, USA), B-cell lymphoma 2 (Bcl-2, SantaCruz biotechnology, Santacruz, CA, USA) in the lung tissues, collagen 1 (SantaCruz biotechnology, Santacruz, CA, USA) and collagen 3 (Abcam, Cambridge, UK) in the heart tissues and glyceraldehyde 3-phosphate dehydrogenase (GAPDH, Santa Cruz Biotechnology, Inc., Santa Cruz, CA, USA) were determined by western blot analysis. Thus, frozen tissues were homogenized in the lysis buffer (Proprep; iNtRON, Seongnam, Korea), and western blot analysis was done as previously described.6
All data are expressed as median (q1, q3). Data were analyzed using SPSS (SPSS v22.0; SPSS Inc., Chicago, IL, USA) for windows. Statistical comparisons were performed as follows. The Mann-Whitney test was used for significant differences between the control (C) and monocrotaline (M) groups. The Kruskal-Wallis test was used for significant differences between M group and U groups. If significant, we further conducted Pairwise by Mann-Whitney test among the U groups. Raw p-value<0.05 was considered statistically significant.
Flurescence-activated cell-sorting (FACS) analyses revealed that the cells used were MSCs. The evidences are as follows. First, the hUCB-MSCs were negative for CD14, CD45, and HLA-DR, implying that we excluded CD14+ cells for macrophages or granulocytes, and CD45+ cells for hematopoietic stem cells. Second, the hUCB-MSCs were positive for CD73, CD90, and CD105 (Fig. 2). According to the International Society for Cellular Therapy, human MSCs are defined as the adherent cells derived from human tissues that are positive for CD73, CD90, and CD105 but negative for CD11b, CD14, CD34, CD79, and HLA-DR surface marker. Therefore, in terms of immunophenotype, the cells used in the present study were considered to be MSCs.
We investigated the immunophenotype of hUCB-MSCs to confirm differentiation potential. hUCB-MSCs have the potential to differentiate into type II alveolar epithelial cells, and are known to have multi-lineage differentiation potential to form bone, adipose and cartilage. As shown in Fig. 3, FACS data indicated that hUCB-MSCs indeed have differentiation potential into type II alveolar epithelial cells. Surfactant protein-C (SP-C), a type II alveolar epithelial cell marker, is not normally expressed in undifferentiated hUCB-MSCs, but it is expressed in hUCB-MSCs after differentiation. SP-C is an extremely hydrophobic 4 kda peptide produced by type II alveolar epithelial cells and it is critical for maintenance of respiratory structure and function. The above results, therefore, had us carefully speculate that hUCB-MSCs can differentiate into type II alveolar epithelial cells.
The optimal dose of hUCB-MSCs has not been established for PAH to assess the feasibility of low-dose hUCB-MSCs transfusion via the jugular vein. Therefore, we investigated the dosedependent effects on PAH in MCT-induced PAH rat. RV pressure was significantly increased in M group compared with C group at weeks 2 [C vs. M; 8.0 mmHg (6.0, 10.0) vs. 29 mmHg (28.0, 42.5)] and 4 [C vs. M; 11.0 mmHg (8.0, 12,0) vs. 49.5 mmHg (37.3, 69.3)]. Decreased RV pressure was observed in all three groups (UA, UB, and UC), but there was no significant difference among the three groups at week 2 [UA vs. UB vs. UC; 14.5 mmHg (13.3, 18.0) vs. 13.0 mmHg (12.5, 16.5) vs. 16.0 mmHg (13.5, 20.0)] and 4 [UA vs. UB vs. UC; 20.5 mmHg (18.5, 21.8) vs. 15.5 mmHg (14.0, 20.0) vs. 14.0 mmHg (13.5, 16.5)] (Fig. 4A). RV hypertrophy showed tendency to decrease in all three groups compared with M group, although the low-dose group (UC) showed slightly higher RV/LV+S ratio at week 2 [C vs. M vs. UA vs. UB vs. UC; 0.26 (0.25, 0.26) vs. 0.36 (0.36, 0.37) vs. 0.29 (0.28, 0.30) vs. 0.29 (0.14, 0.31) vs. 0.31 (0.28, 0.36)] and 4 [C vs. M vs. UA vs. UB vs. UC; 0.28 (0.25, 0.29) vs. 0.80 (0.65, 0.97) vs. 0.44 (0.24, 0.52) vs. 0.43 (0.24, 0.51) vs. 0.69 (0.55, 0.71)]. However, the differences between M and U groups were not statistically significant (Fig. 4B). Medial wall thickening of the pulmonary arterioles was improved, however, there was no significant difference among the three U groups at week 4 [C vs. M vs. UA vs. UB vs. UC; 21.24 (15.82, 22.75) vs. 42.31 (40.58, 44.17) vs. 31.63 (27.19, 32.25) vs. 34.78 (27.01, 36.05) vs. 32.52(31.9, 35.53)] (Fig. 5A). The number of intra-acinar arteries was improved in UA and UB groups, but there was no significant difference among the three groups at week 4 [C vs. M vs. UA vs. UB vs. UC; 0.80 (0.78, 1.06) vs. 1.91(1.43, 2.25) vs. 1.31 (0.99, 1.48) vs. 1.07 (0.94, 1.21) vs. 1.45 (1.35, 1.55] (Fig. 5B). The representative data of Victoria blue staining are shown in Fig. 5C. The above results suggest that the lowest-dose (3×105 cells/rat) of hUCB-MSCs can also be effective in treating PAH rats. Because the low dose therapy has potential clinical significance in cost effectiveness, we carried out second experiment of low-dose hUCB-MSCs with added factor of treatment timing.
To verify the therapeutic effect on MCT-induced PAH rats according to different timings using low dose hUCB-MSCs, we compared mean RV pressure among three groups (UD, UE, UF). The result showed that hUCB-MSCs decreased the mean RV pressure in all three groups at weeks 2 [C vs. M vs. UD vs. UE vs. UF; 11.0 mmHg (11.0, 12.0) vs. 27.0 mmHg (25.0, 33.0) vs. 16.0 mmHg (15.0, 16.0) vs. 14.0 mmHg (13.0, 16.0) vs. 16.0 mmHg (16.0, 18.0)] and 4 [C vs. M vs. UD vs. UE vs. UF; 11.0 mmHg (10.0, 11.0) vs. 44.0 mmHg (38.0, 45.0) vs. 15.0 (11.0, 23.0) vs. 13.0 mmHg (11.0, 18.0) vs. 13.5 mmHg (13.0, 14.0), not significant]. The difference among U groups was not statically significant (Fig. 6A). Therefore, not only reversal therapy but also prophylaxis of hUCB-MSCs improved PAH in terms of RV pressure.
To explore whether these therapeutic strategies have an effect on RV hypertrophy, we compared RV/LV+S ratio (Table 1). As shown in Fig. 6B, RV/LV+S ratio was increased in M group compared with C group but there was no significant difference between M and U groups at weeks 2 [C vs. M vs. UD vs. UE vs. UF; 0.29 (0.29, 0.33) vs. 0.40 (0.40, 0.44) vs. 0.40 (0.34, 0.57) vs. 0.32 (0.27, 0.37) vs. 0.34 (0.32, 0.40)] and 4 [C vs. M vs. UD vs. UE vs. UF; 0.31 (0.31, 0.32) vs. 0.81 (0.67, 0.92) vs. 0.71 (0.66, 0.78) vs. 0.62 (0.58, 0.65) vs. 0.68 (0.54, 0.69)].
Next, we investigated pathological changes to confirm the preventive effects of hUCB-MSCs on PAH. As seen in Fig. 7A and C, medial wall thickness of pulmonary arteriole was slightly decreased in the UD, UE, and UF groups but not significant at weeks 2 [C vs. M vs. UD vs. UE vs. UF; 26.78 (23.86, 29.70) vs. 38.22 (32.23, 44.63) vs. 40.43 (38.04, 43.77) vs. 34.76 (29.11, 39.93) vs. 35.40 (31.98, 36.86)] and 4 [C vs. M vs. UD vs. UE vs. UF; 26.89 (25.82, 29.33) vs. 40.90 (40.89, 49.22) vs. 33.62 (31.55, 38.21) vs. 36.16 (36.02, 39.73) vs. 36.67 (30.47, 39.11)]. Furthermore, the number of intra-acinar arteries was significantly decreased in all three groups at week 4 [C vs. M vs. UD vs. UE vs. UF; 0.71 (0.70, 0.79) vs. 1.80 (1.78, 2.05) vs. 1.10 (0.86, 1.38) vs. 1.13 (1.12, 1.24) vs. 1.57 (1.41, 1.65)] (Fig. 7B).
We examined the protein expression levels of ERA, Bcl-2 in the lung tissues and collagen 1, collagen 3 in the heart tissues after hUCB-MSCs transfusion (Fig. 8). In the lung tissues, Bcl-2 was significantly decreased in the UF groups compared with the M group at week 4 [M vs. UD vs. UE vs. UF; 2.33 (2.26, 2.41) vs. 1.08 (0.91, 1.51) vs. 1.09 (0.74, 1.44) vs. 0.76 (0.65, 0.80)], whereas collagen 3 in the heart tissues was significantly decreased in the UD and UF groups at week 4 [M vs. UD vs. UE vs. UF; 1.81 (1.46, 2.16) vs. 1.17 (1.03, 1.49) vs. 1.21 (1.10, 1.39) vs. 1.09 (1.09, 1.17)]. There were no significant changes between M and U groups in ERA and collagen 1.
The results in the present study suggested that low-dose (3×105 cells/rat) reversal treatment of hUCB-MSCs via jugular vein did improve PAH and low dose early treatment also partially prevented MCT-induced PAH in our rat model. The ability to use low-dose stem cells has great potential to help commercialize hUCB-MSCs because low dose transfusion has an advantage of competitive price compared with other therapeutic strategies, and the cost for stem cell therapy can cause a significant economic burden to households. Furthermore, early transfusion of hUCB-MSCs has a significant implication because progression of the disease is relentless and refractory and early treatment was as effective as reversal treatment on RV pressure in this study.
This research is meaningful because low dose treatment reduces the costs of therapy and is the first to investigate the early transfusion effect of hUCB-MSCs on PAH. Early treatment attenuated expression levels of collagen 3 protein in the heart tissues: abnormally high expression levels caused by MCT were suppressed by an early transfusion of hUCB-MSCs, and therefore, early treatment may improve RV systolic function because increased deposition of collagen protein contributes to the deterioration of cardiac systolic function. These results led us to conclude that dual or reversal treatment of hUCB-MSCs is more effective than early treatment.
In the present study, we used hUCB-MSCs that could be differentiated into type II alveolar epithelial cells. Because the specific dose range of hUCB-MSCs for PAH has not yet been determined, we performed a dose escalation test. Dose raged from 3×106 cells/rat to 3×105 cells/rat showed similar levels of improvement, demonstrating that low-dose cell therapy has effects on MCT-induced PAH rats.
The main effects of the early transfusion of hUCB-MSCs on PAH are as follows. First, early stage treatment decreased mean RV pressure. Effects of reversal treatment have been previously confirmed by changes of several protein expression levels related to PAH and immunomodulation in MCT-induced PAH rats.67 However, the present study is a first report on early stage treatment. Second, early treatment improved pulmonary pathology. The number of neomuscularized intra-acinar arteries were decreased. Third, early treatment attenuated protein expression levels of collagen 3 in the heart tissues. Therefore, the effects of prevention therapy are comparable to that of reversal therapy.
These findings may have important implications for early stage therapy of PAH. PAH is progressively worse and occasionally fatal despite various medical interventions. PAH ordinarily leads to death within 2-3 years after first diagnosis, therefore, hUCB-MSCs injection at the initial phase is critical for prognosis because it is difficult to get back the lung structure and function once it becomes worsen. There is a report that early intervention may improve the prognosis of PAH. In 2002, Sitbon, et al.26 reported that the survival of patients with medical treatment was determined by the severity at baseline which was determined by clinical outcomes and hemodynamics, and suggested that early medical care can bring a favorable prognosis. Our data suggested that hUCB-MSCs in a PAH rat model are capable of differentiate into type II alveolar epithelial cells: we confirmed that differentiation induced hUCB-MSCs were possible to be specialized into type II alveolar epithelial. In 2003, Ortiz, et al.27 studied that MSCs may limit the injurious effect of bleomycin by replacing type II alveolar epithelial cells. Therefore, the present data indicate that early transfusion of hUCB-MSCs has beneficial effects on the prevention of PAH.
Low-dose hUCB-MSCs may improve PAH by regulating protein expression levels in lung and heart. The exact mechanism of low-dose hUCB-MSCs' effect is not currently known. Nevertheless, it has been suggested that hUCB-MSCs control the cardiac collagen deposition via paracrine mechanism. In this study, hUCB-MSCs decreased collagen 3 protein expression in the heart tissues, suggesting that low-dose hUCB-MSCs may improve RV function because increased collagen contents contribute to cardiac malfunction. Another possibility is that hUCB-MSCs downregulated Bcl-2 protein expression in the lung tissues, which is related with apoptosis. We earlier showed that high dose hUCB-MSCs have similar effects although slightly better. Therefore, it is likely that low-dose hUCB-MSCs act similarly as high-dose transfusions, resulting in the recovery of protein expression levels.
MSCs not only have reversal therapeutic effects on diverse diseases but also have prophylactic effects on several diseases. MSCs have been used in preclinical and clinical trials in treatments for graft-versus-host disease28 and autoimmune disease such as systemic sclerosis and type 1 diabetes mellitus.2930 In 2007, Mei, et al.31 that MSCs injection into the jugular vein significantly prevented lipopolysaccharide-induced acute pulmonary inflammation in mice. In 2008, Polchert, et al.32 suggested that activated MSCs by IFN-γ could prevent graft-versus-host disease. MSCs also have prophylactic effects on lung disease. In 2005, Zhao, et al.33 showed that bone marrow derived endothelial-like progenitor cells could prevent the MCT-damaged lung and microvasculature structure, and in 2013, Pierro, et al.34 reported that hUCB-MSCs prevented bronchopulmonary dysplasia by paracrine effect without adverse lung effect.
The effect of the early transfusion of MSCs in several diseases might be due to immune regulation ability by paracrine effect. MSCs have been shown to have a preventive effect on graft versus host disease through prophylactic immunosuppressive pathway by T cell regulation. Prophylactic treatment of bone marrow-derived MSCs by airway delivery improves arrested alveolar and vascular growth in part through paracrine activity in a bronchopulmonary dysplasia model.35 These findings suggest that early stage administration of hUCB-MSCs has immunomodulatory ability and recover injured alveolar and vascular cells as well.
The exact mechanism of hUCB-MSCs' prevention effect in PAH is not clearly understood. Whether it is an anti-apoptotic effect, inhibition of neo-muscularization of pulmonary arterioles or inhibition of cardiac fibrosis remains unknown. Various factors may influence these therapeutic actions on PAH. One probable mechanism of the prevention effect is controlling cardiac remodeling via paracrine mechanism. It has been known that MSCs are capable of cardiac repair in an infarcted rat heart,36 and we showed in the present study, that a preventive administration of hUCB-MSCs attenuated cardiac hypertrophy and increased cardiac protein expression levels of collagen 3, thus suggesting that hUCB-MSCs can possibly control cardiac hypertrophy and fibrosis by regulating a number of molecules such as collagen. The administration of hUCB-MSCs via the external jugular vein has shown an ability to control the collagen deposition and anti-apoptotic mechanisms by both preventive and reversal treatment. It is, therefore, highly likely that this identical mechanism may be involved in both therapeutic actions. However, the exact mechanism of preventive effect in PAH rat models is still unclear.
Limitations of this study are as follows. First of all, preventive intervention on PAH may not have important clinical meaning because the prevalence rate of PAH is low and early diagnosis is difficult. Second, the mechanism has not yet been elucidated although there are several reports that MSCS improved MCT-induced PAH rats. Third, it has not been confirmed that hUCB-MSCs have a potential to differentiate into type II alveolar epithelial cells in animal models. Fourth, this study was performed using small number of animals.
In conclusion, prophylactic early application of hUCB-MSCs on PAH may provide a basis for the development of a new approach to the treatment of PAH. The present study demonstrated that low-dose hUCB-MSCs treatment is effective in MCT-induced PAH rats. Also, low-dose early treatment improved PAH as well, although dual or reversal treatment is more effective.
Figures and Tables
Fig. 1
Study design. (A) Dose-escalation test protocol. The hUCB-MSCs are transfused at 1 week after MCT injection. UA group (n=5) was assigned to receive high-dose hUCB-MSCs (3×106 cells/rat), UB group (n=5) was intermediate-dose hUCB-MSCs (1.5×106 cells/rat) and UC group (n=6) was low-dose hUCB-MSCs (3×105 cells/rat). (B) Treatment time test protocol. Low-dose (3×105 cells/rat) of hUCB-MSCs were transfused at day 1 (UD group, n=4), day 1+week 1 (UE group, n=4) or week 1 (UF group, n=4). MCT, monocrotaline; hUCB-MSCs, human umbilical cord blood derived-mesenchymal stem cells; UD, early treatment of low dose hUCB-MSCs at day 1; UE, dual transfusion of low dose hUCB-MSCs at day 1 and week 1; UF, reversal treatment of low dose hUCB-MSC at week 1.
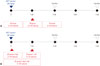
Fig. 2
Characteristics of hUCB-MSCs. (A and B) The hUCB-MSCs were stained with various combinations of saturating amounts of monoclonal antibodies conjugated with fluorescein isothiocyanate or phycoerythrin: CD14, CD45, HLA-DR, CD73, CD90, and CD105. At least 104 events were analyzed by flow cytometry with the cellquest software. Immunophenotype of hUCB-MSCs. MSC-clone of human cord blood at passage 4 was labeled with antibodies against the indicated antigens and they were analyzed by flow cytometry. FITC-A, isothiocyanate antibody; PE-A, phycoerythrin antibody; hUCB-MSCs, human umbilical cord blood derived-mesenchymal stem cells.
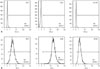
Fig. 3
Differentiation potentiality of hUCB-MSCs on various mesenchymal lineage cells. (A) Inverted phase contrast microscopy images of hUCB-MSCs in the 4 passage. (B) To evaluate the multi-lineage differentiation capacity of the hUCB-MSCs, we cultured them with osteo, adipo, chondrogenic differentiation media. Osteogenic differentiation was observed, demonstrated by morphological changes and the accumulation of alkaline phosphatase. Adipogenic differentiation was observed, shown by the accumulation of oil red-O-staining. Chondrogenic differentiation was also observed, shown by the accumulation of safranin-O-staining sulfated proteoglycans. Finally, differentiation into type II alveolar epithelial cell was observed, shown by SP-C expression. hUCB-MSCs, human umbilical cord blood derived-mesenchymal stem cells.
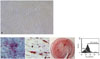
Fig. 4
RV pressure and RV/LV+S ratio in MCT-induced PAH rats according to dose of hUCB-MSCs. (A) RV pressure was significantly decreased in UA, UB and UC groups at weeks 2 and 4. There was no significant difference among the three groups. (B) RV/LV+S ratio slightly decreased in UA, UB and UC groups. *p<0.05, compared with C, †p<0.05, compared with M. MCT, monocrotaline; PAH, pulmonary arterial hypertension; hUCB-MSCs, human umbilical cord blood derived mesenchymal stem cells; RV, right ventricle; LV, left ventricle; S, septum; C, control group; M, monocrotaline group; UA, 3×106 cells/rat; UB, 1.5×106 cells/rat; UC, 3×105 cells/rat.
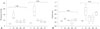
Fig. 5
Pathologic findings in MCT-induced PAH rats according to dose of hUCB-MSCs at week 4. (A) Low-dose of hUCB-MSCs also effectively decreased pulmonary arteriole medial wall thickening. (B) The number of intra-acinar arteries was decreased in UA and UB groups. (C) Low-dose hUCB-MSCs also attenuated pulmonary pathological changes in MCT-induced PAH rat (Victoria blue staining, ×400). *p<0.05, compared with C, †p<0.05, compared with M. No, number; C, control group; M, monocrotaline group; UA, 3×106 cells/rat; UB, 1.5×106 cells/rat; UC, 3×105 cells/rat; MCT, monocrotaline; PAH, pulmonary arterial hypertension; hUCB-MSCs, human umbilical cord blood derived mesenchymal stem cells.
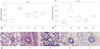
Fig. 6
RV pressure and RV/LV+S ratio according to treatment timing by low-dose treatment of hUCB-MSCs in MCT-induced PAH rats. (A) RV pressure was decreased in UD, UE, and UF group. (B) No effect on RV hypertrophy by low-dose treatment of hUCB-MSCs in MCT-induced PAH rats. *p<0.05, compared with C, †p<0.05, compared with M. MCT, monocrotaline; PAH, pulmonary arterial hypertension; hUCB-MSCs, human umbilical cord blood derived mesenchymal stem cells; RV, right ventricle; LV+S, left ventricle+septum; C, control group; M, monocrotaline group; UD, early treatment group of low dose hUCB-MSCs at day 1; UE, dual transfusion group of low dose hUCB-MSCs at day 1 and week 1; UF, reversal treatment group of low dose hUCB-MSC at week 1; hUCB-MSCs, human umbilical cord blood derived-mesenchymal stem cells.
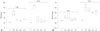
Fig. 7
Pulmonary pathologic finding according to treatment timing by low-dose treatment of hUCB-MSCs in MCT-induced PAH rats. (A) Medial wall thickening of pulmonary arterioles was decreased in UD, UE, and UF groups at week 4, but not significant. (B) The number of mucularized intra-acinar arteries was significantly decreased in UD, UE, and UF groups at weeks 4. (C) Low-dose hUCB-MSCs attenuated pulmonary pathological changes in MCT-induced PAH rat (Victoria blue staining, ×400). *p<0.05, compared with C, †p<0.05, compared with M. MCT, monocrotaline; PAH, pulmonary arterial hypertension; hUCB-MSCs, human umbilical cord blood derived mesenchymal stem cells; C, control group; M, monocrotaline group; UD, early treatment group of low dose hUCB-MSCs at day 1; UE, dual transfusion group of low dose hUCB-MSCs at day 1 and week 1; UF, reversal treatment group of low dose hUCB-MSC at week 1.
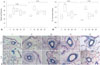
Fig. 8
Expressions of ERA, Bcl-2, collagen 1, and collagen 3 according to treatment timing by low-dose treatment of hUCB-MSCs in MCT-induced PAH rats. (A) Protein expression of ERA was significantly increased in the lung tissues of M group compared with C group at week 4. (B) Protein expression of Bcl-2 was significantly decreased in the lung tissues of UF groups compared with M group at week 4. (C) Collagen 1 in the heart tissues was significantly increased in M group compared with C group at week 4. (D) Collagen 3 in the heart tissues was significantly decreased UD and UF group compared with M group at week 4. *p<0.05, compared with C, †p<0.05, compared with M. MCT, monocrotaline; PAH, pulmonary arterial hypertension; hUCB-MSCs, human umbilical cord blood derived mesenchymal stem cells; C, control group; M, monocrotaline group; UD, early treatment group of low dose hUCB-MSCs at day 1; UE, dual transfusion group of low dose hUCB-MSCs at day 1 and week 1; UF, reversal treatment group of low dose hUCB-MSC at week 1; Dual, twice-transfusion of hUCB-MSCs group; ERA, endothelin receptor A; Bcl-2, B-cell lymphoma 2; GAPDH, glyceraldehyde 3-phosphate dehydrogenase.
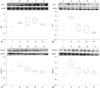
Table 1
Changes of Body and Organ Weight in UD, UE, and UF Groups (g)
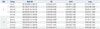
RV, right ventricle; LV, left ventricle; S, septum; C, control group; M, monocrotaline group; UD, early treatment group of low dose hUCB-MSCs at day 1; UE, dual transfusion group of low dose hUCB-MSCs at day 1 and week 1; UF, reversal treatment group of low dose hUCB-MSC at week 1; hUCB-MSCs, human umbilical cord blood derived-mesenchymal stem cells.
*p<0.05, compared with C, †p<0.05, compared with M.
ACKNOWLEDGEMENTS
The authors gratefully acknowledge the support provided by Basic Science Research Program through the National Research Foundation of Korea funded by the Ministry of Education (2013 R1A1A3004619), and intramural research promotion grants from Ewha Womans University School of Medicine.
References
1. Simonneau G, Gatzoulis MA, Adatia I, Celermajer D, Denton C, Ghofrani A, et al. Updated clinical classification of pulmonary hypertension. J Am Coll Cardiol. 2013; 62:25 Suppl. D34–D41.


2. Rabinovitch M. Molecular pathogenesis of pulmonary arterial hypertension. J Clin Invest. 2012; 122:4306–4313.


3. Huertas A, Perros F, Tu L, Cohen-Kaminsky S, Montani D, Dorfmüller P, et al. Immune dysregulation and endothelial dysfunction in pulmonary arterial hypertension: a complex interplay. Circulation. 2014; 129:1332–1340.


4. Price LC, Wort SJ, Perros F, Dorfmüller P, Huertas A, Montani D, et al. Inflammation in pulmonary arterial hypertension. Chest. 2012; 141:210–221.


5. Humbert M, Sitbon O, Simonneau G. Treatment of pulmonary arterial hypertension. N Engl J Med. 2004; 351:1425–1436.


6. Lee H, Lee JC, Kwon JH, Kim KC, Cho MS, Yang YS, et al. The effect of umbilical cord blood derived mesenchymal stem cells in monocrotaline-induced pulmonary artery hypertension rats. J Korean Med Sci. 2015; 30:576–585.


7. Kim KC, Lee JC, Lee H, Cho MS, Choi SJ, Hong YM. Changes in caspase-3, B cell leukemia/lymphoma-2, interleukin-6, tumor necrosis factor-α and vascular endothelial growth factor gene expression after human umbilical cord blood derived mesenchymal stem cells transfusion in pulmonary hypertension rat models. Korean Circ J. 2016; 46:79–92.


8. Li J, Li D, Liu X, Tang S, Wei F. Human umbilical cord mesenchymal stem cells reduce systemic inflammation and attenuate LPSinduced acute lung injury in rats. J Inflamm (Lond). 2012; 9:33.


9. Hansmann G, Fernandez-Gonzalez A, Aslam M, Vitali SH, Martin T, Mitsialis SA, et al. Mesenchymal stem cell-mediated reversal of bronchopulmonary dysplasia and associated pulmonary hypertension. Pulm Circ. 2012; 2:170–181.


10. Chang YS, Ahn SY, Yoo HS, Sung SI, Choi SJ, Oh W, et al. Mesenchymal stem cells for bronchopulmonary dysplasia: phase 1 dose-escalation clinical trial. J Pediatr. 2014; 164:966–972.


11. Zhang WG, He L, Shi XM, Wu SS, Zhang B, Mei L, et al. Regulation of transplanted mesenchymal stem cells by the lung progenitor niche in rats with chronic obstructive pulmonary disease. Respir Res. 2014; 15:33.


12. Jin Z, Pan X, Zhou K, Bi H, Wang L, Yu L, et al. Biological effects and mechanisms of action of mesenchymal stem cell therapy in chronic obstructive pulmonary disease. J Int Med Res. 2015; 43:303–310.


13. Barczyk M, Schmidt M, Mattoli S. Stem cell-based therapy in idiopathic pulmonary fibrosis. Stem Cell Rev. 2015; 11:598–620.


14. Weiss DJ. Concise review: current status of stem cells and regenerative medicine in lung biology and diseases. Stem Cells. 2014; 32:16–25.


15. Souidi N, Stolk M, Seifert M. Ischemia-reperfusion injury: beneficial effects of mesenchymal stromal cells. Curr Opin Organ Transplant. 2013; 18:34–43.
16. Xue J, Li X, Lu Y, Gan L, Zhou L, Wang Y, et al. Gene-modified mesenchymal stem cells protect against radiation-induced lung injury. Mol Ther. 2013; 21:456–465.


17. Wannemuehler TJ, Manukyan MC, Brewster BD, Rouch J, Poynter JA, Wang Y, et al. Advances in mesenchymal stem cell research in sepsis. J Surg Res. 2012; 173:113–126.


18. Baber SR, Deng W, Master RG, Bunnell BA, Taylor BK, Murthy SN, et al. Intratracheal mesenchymal stem cell administration attenuates monocrotaline-induced pulmonary hypertension and endothelial dysfunction. Am J Physiol Heart Circ Physiol. 2007; 292:H1120–H1128.


19. Ionescu L, Byrne RN, van Haaften T, Vadivel A, Alphonse RS, Rey-Parra GJ, et al. Stem cell conditioned medium improves acute lung injury in mice: in vivo evidence for stem cell paracrine action. Am J Physiol Lung Cell Mol Physiol. 2012; 303:L967–L977.


20. Lee JW, Fang X, Krasnodembskaya A, Howard JP, Matthay MA. Concise review: mesenchymal stem cells for acute lung injury: role of paracrine soluble factors. Stem Cells. 2011; 29:913–919.


21. Umar S, de Visser YP, Steendijk P, Schutte CI, Laghmani EH, Wagenaar GT, et al. Allogenic stem cell therapy improves right ventricular function by improving lung pathology in rats with pulmonary hypertension. Am J Physiol Heart Circ Physiol. 2009; 297:H1606–H1616.


22. Kern S, Eichler H, Stoeve J, Klüter H, Bieback K. Comparative analysis of mesenchymal stem cells from bone marrow, umbilical cord blood, or adipose tissue. Stem Cells. 2006; 24:1294–1301.


23. Musina RA, Bekchanova ES, Belyavskii AV, Grinenko TS, Sukhikh GT. Umbilical cord blood mesenchymal stem cells. Bull Exp Biol Med. 2007; 143:127–131.


24. Liu CH, Hwang SM. Cytokine interactions in mesenchymal stem cells from cord blood. Cytokine. 2005; 32:270–279.


25. Lee HR, Kim TH, Choi KJ, Choi KC. Effects of octylphenol on the expression of cell cycle-related genes and the growth of mesenchymal stem cells derived from human umbilical cord blood. Int J Mol Med. 2014; 33:221–226.


26. Sitbon O, Humbert M, Nunes H, Parent F, Garcia G, Hervé P, et al. Long-term intravenous epoprostenol infusion in primary pulmonary hypertension: prognostic factors and survival. J Am Coll Cardiol. 2002; 40:780–788.


27. Ortiz LA, Gambelli F, McBride C, Gaupp D, Baddoo M, Kaminski N, et al. Mesenchymal stem cell engraftment in lung is enhanced in response to bleomycin exposure and ameliorates its fibrotic effects. Proc Natl Acad Sci U S A. 2003; 100:8407–8411.


28. Le Blanc K, Rasmusson I, Sundberg B, Götherström C, Hassan M, Uzunel M, et al. Treatment of severe acute graft-versus-host disease with third party haploidentical mesenchymal stem cells. Lancet. 2004; 363:1439–1441.


29. Gitelman SE, Haller MJ, Schatz D. Autologous nonmyeloablative hematopoietic stem cell transplantation in newly diagnosed type 1 diabetes mellitus. JAMA. 2009; 302:624–625.


30. Couri CE, Oliveira MC, Stracieri AB, Moraes DA, Pieroni F, Barros GM, et al. C-peptide levels and insulin independence following autologous nonmyeloablative hematopoietic stem cell transplantation in newly diagnosed type 1 diabetes mellitus. JAMA. 2009; 301:1573–1579.


31. Mei SH, McCarter SD, Deng Y, Parker CH, Liles WC, Stewart DJ. Prevention of LPS-induced acute lung injury in mice by mesenchymal stem cells overexpressing angiopoietin 1. PLoS Med. 2007; 4:e269.


32. Polchert D, Sobinsky J, Douglas G, Kidd M, Moadsiri A, Reina E, et al. IFN-gamma activation of mesenchymal stem cells for treatment and prevention of graft versus host disease. Eur J Immunol. 2008; 38:1745–1755.


33. Zhao YD, Courtman DW, Deng Y, Kugathasan L, Zhang Q, Stewart DJ. Rescue of monocrotaline-induced pulmonary arterial hypertension using bone marrow-derived endothelial-like progenitor cells: efficacy of combined cell and eNOS gene therapy in established disease. Circ Res. 2005; 96:442–450.


34. Pierro M, Ionescu L, Montemurro T, Vadivel A, Weissmann G, Oudit G, et al. Short-term, long-term and paracrine effect of human umbilical cord-derived stem cells in lung injury prevention and repair in experimental bronchopulmonary dysplasia. Thorax. 2013; 68:475–484.

