Abstract
Purpose
Evidence on the contribution of genes to the hereditary predisposition to pulmonary arterial hypertension (PAH) is limited.
Materials and Methods
In this study, we hypothesized that single nucleotide variants in vascular endothelial growth factor (VEGF) gene may alter gene function and expression and may be associated with PAH risk. Five putatively functional loci (rs699947C>A and rs833061T>C in the promoter, rs3025040C>T, rs10434G>A and rs3025053G>A in the 3'-UTR) in the VEGF gene were genotyped and analyzed in a retrospective study of 587 patients with PAH and 736 healthy subjects from southern China.
Results
We found that the rs833061T>C polymorphism was significantly associated with PAH risk, while the other single nucleotide polymorphisms were not. Compared to carriers with TT genotype, those with rs833061C variant genotype (CT/CC) had an increased risk of PAH (odds ratio=1.47, 95% confidence interval=1.18–1.83, p=0.001). Functional assays indicated that CT/CC variant genotype had significantly higher mRNA levels of VEGF in peripheral blood mononuclear cells than TT genotype (p=0.021). Luciferase reporter assay indicated that having a C allele conferred a significantly higher transcription activity than that with a T allele.
Pulmonary arterial hypertension (PAH) is a progressive, debilitating, incurable disease of pulmonary vasculature and is characterized by elevations in mean pulmonary artery pressure exceeding 25 mm Hg at rest and a pulmonary capillary wedge pressure of less than 15 mm Hg.1 With the development of vascular remodeling and increased right ventricular pressure overload, patients with PAH usually die from right ventricular failure.2 Over the last several decades, despite numerous etiologic and therapeutic efforts, mortality from PAH remains unacceptably high, and its prognosis remains dismal.3 The identification of novel biomarkers associated with PAH susceptibility and development might help to explain the precise mechanisms of PAH and facilitate personal targeted therapies for improved overall survival rates.4
Vascular endothelial growth factor (VEGF) is a pivotal mediator of angiogenesis and endothelial cell biology, VEGF also plays important roles in various human diseases via diversified and complex ranges of signaling pathways.56 Evidence suggests that VEGF acts on endothelial cells specifically and has multifarious influences that include mediating increased angiogenesis, vascular permeability, vasculogenesis, and endothelial cell growth.789 The biological role of VEGF in PAH has been gradually recognized due to PAH having intimate connections with changes in vascular architecture. One study suggested that increased expression of VEGF by mesenchymal stem cells could result in enhanced vessel formation during treprostinil treatment in the patients with pulmonary hypertension, whereas silencing the VEGF gene blocked the pro-angiogenic effect.10 In addition, other evidence has suggested that VEGF levels are elevated in patients with PAH, compared to healthy individuals.11
The VEGF gene is located on chromosome 6p21.3, spanning over ~16 kb and encoding a 232-amino acid protein. To date, genetic variations, including single nucleotide polymorphism (SNP), in the VEGF gene have been investigated, revealing intimate connections to a multitude of complex diseases: studies demonstrated that the SNP rs2010963G>C in the 5'-untranslated region (UTR) of the VEGF gene is a genetic risk factor for cardiac septal defects, by increasing the expression of VEGF.1213 Further, Han, et al.14 investigated the associations between VEGF SNPs and coronary heart disease (CHD) risk in the Chinese Han population and found that -460 T/C had an increased risk of CHD. To date, few reports have evaluated the associations between polymorphisms of VEGF and PAH risk. In this study, we hypothesized that genetic variants in the VEGF gene could alter its biological function and expression and, thus, may be associated with PAH susceptibility.
Conducting independent retrospective studies, we tested the relationship between five putative functional SNPs (rs699947C>A and rs833061T>C in the promoter, rs3025040C>T, rs10434G>A and rs3025053G>A in the 3'-UTR) in the VEGF gene and PAH risk. Consecutive functional experiments were performed to determine the biological effects of these polymorphisms.
In this study, a total of 587 PAH patients were enrolled from the Panyu Hexian Memorial Hospital and Nanfang Hospital during March 2009 to December 2014; 736 age- (±5 years) and sex-matched healthy controls were also registered in the physical examination program during the same period. The diagnosis of patients with PAH was determined by a hemodynamic measurement during right-heart catheterisation for all patients. In brief, PAH was diagnosed according to a mean pulmonary arterial pressure ≥25 mm Hg associated with normal pulmonary capillary wedge pressure. Hemodynamic evaluation by right-heart catheterisation was performed at baseline in all subjects according to a previously described protocol.15 All participants were genetically unrelated and of Han Chinese ethnicity, and none had received a blood transfusion within the last six months. After written informed consent was obtained from each participant, they underwent an interview to collect information regarding demographics and other selected factors and were asked to donate 5 mL of peripheral venous blood. The study was approved by the Institutional Review Board of Panyu Hexian Memorial Hospital.
Using the public HapMap database (http://HapMap.ncbi.nlm.nih.gov/), we searched for putatively functional SNPs located in the predicted 2000 bp promoter, exons and 3'-UTR of the VEGF gene. Five such SNPs in the VEGF gene (i.e., rs699947C>A and rs833061T>C in the promoter, rs3025040C>T, rs10434G>A and rs3025053G>A in the 3'-UTR) were identified and had a minor allele frequency >5% in the Chinese population. These SNPs were chosen for genotyping and their associations with PAH risk were analyzed in this study.
Genomic DNA was extracted from 2 mL of peripheral blood using a DNA Blood Mini Kit (Qiagen, Valencia, CA, USA) according to the manufacturer's instructions. The genotypes for each SNP were identified by TaqMan allelic discrimination experiment performing in ABI PRISM 7500 Sequence Detection Systems (Applied Biosystems, Foster City, CA, USA). The primers and probes were designed using Primer Express 3.0 (Applied Biosystems) and synthesized by Shanghai GeneCore Biotechnologies (Shanghai, China). Approximately 10% of the samples for each of the 5 SNPs were randomly selected to perform repeat assays.
Forty samples of peripheral blood mononuclear cells (PBMCs) were purified from PAH patients and healthy controls, respectively. In short, blood was collected from patients and healthy subjects using one-step cell separation (Becton Dickinson, Mountain View, CA, USA). The samples were vortexed and then centrifuged at 1800 reactive centrifugal force at ambient temperature for 30 minutes. The PBMC cell layer was transferred to a 15-mL tube. After that, the PBMCs were washed twice with phosphate buffer saline and lysed in RNeasy RLT buffer (Qiagen, Valencia, CA, USA). Total RNA was extracted using Trizol Reagent (Invitrogen, Carlsbad, CA, USA) and then reversely transcribed into complementary DNA using oligo primer and Superscript II (Invitrogen). The relative mRNA levels of VEGF were detected on the ABI Prism 7500 sequence detection system (Applied Biosystems) based on the SYBR-Green method. The β-actin was used as an internal reference gene. The primers used for VEGF were 5'-CCTCGCAGTCCGAGCCGGA-3' (forward) and 5'-GACCCAAAGTGCTCCTCGAAG-3' (reverse) and for β-actin were 5'-GGCGGCACCACCATGTACCCT-3' and 5'-AGGGGC CGGACTCGTCATACT-3'. Relative quantification of VEGF mRNA was performed according to the 2-ΔCt method.16 All assays were executed in triplicate, at least three times independently.
Two luciferase reporters consisting of the rs833061T or rs833061C allele were constructed to determine whether this polymorphism could alter the transcriptional activity of VEGF promoter (Fig. 1A). The full length VEGF promoter region (from -2148 to +324 nt relative to the transcription start site, 2475 bp) contained the common rs833061T allele was commercially synthesized by Sangon Biotech Co., Ltd. (Shanghai, China) and inserted into the pGL3 basic vector (Promega, Madison, WI, USA). The mutated T allelic reporter construct was acquired from the rs833061T construct by site-directed mutagenesis using the QuikChange site-directed mutagenesis kit (Stratagene, La Jolla, CA, USA). All constructive vectors were confirmed by direct sequencing.
Human pulmonary artery smooth muscle cells (PASMCs) purchased from the Chinese Academy of Sciences Cell Bank (Shanghai, China) were used to perform bioassays. For the luciferase assay, PASMCs were seeded into 24-well plates and incubated for 24 h. The cells were then transfected with 2.5 µg of the VEGF promoter luciferase plasmid containing a wild-type or variant allele using lipofectamine® 2000 Transfection Reagent (Invitrogen). As an internal control, each plasmid was cotransfected with 10 ng of pRL-TK plasmid having the Renilla luciferase gene (Promega). After 48 h of incubation, cells were collected and dissociated. A Dual-Luciferase Reporter Assay System (Promega) was used to assess the luciferase activity, which was normalised against the activity of the Renilla luciferase gene. Triplicate experiments were done for each plasmid construct independently.
The differences in the distribution of demographic characteristics and genotypes between patient samples and controls were compared using chi-square test. The associations between each SNP and PAH susceptibility were tested using logistic regression with or without adjusted for surrounding factors. The hereditary effects of each SNP in codominant, dominant, additive, and recessive models were also estimated by logistic analyses. The best genetic model for each SNP was identified using the Akaike's information criterion (AIC) value.17 Student's t test was used to compare the transcriptional activities of the luciferase reporter genes between different constructs and that of VEGF expression levels. All tests were two-sided using the R software (version 3.0.2; The R Foundation for Statistical Computing). p<0.05 was considered statistically significant.
The baseline characteristics of the patients and controls are described in Table 1. As expected, no noticeable deviations in age, sex, alcohol consumption, and family history of PAH were observed between patients and the controls (p>0.05 for all). However, compared with the healthy subjects, a greater number of patients with PAH were smokers (p<0.05). These factors were further adjusted in later multivariate logistics regression analysis to test the relevancies of VEGF polymorphisms in PAH risk.
All of the genotype distributions of the studied SNPs between the patients and controls are summarized Table 2. Genotype frequencies of all SNPs in the controls were in agreement with the Hardy-Weinberg equilibrium (p>0.05). Among the five polymorphisms, the proportion of genotype frequencies in the rs833061T>C SNP differed significantly between patients and controls (p=0.015). Compared to those with rs833061TT genotype, carriers with CT variant genotype exhibited a significantly increased risk of PAH [odds ratio (OR)=1.39, 95% confidence interval (CI)=1.11–1.75; p=0.004], as did carriers with CC variants ha (OR=1.73, 95% CI=1.03–2.93; p=0.04). The pernicious effect of C variant genotypes on PAH risk was significant after adjustment for other factors, including age, sex, alcohol consumption, smoking, and family history of PAH, with an OR of 1.44 for TC heterozygotes (95% CI=1.14–1.80; p=0.002) and an OR of 1.77 for CC variant homozygotes (95% CI=1.04–3.01; p=0.034). With dichotomous analysis, C variant genotype (CT/CC) conferred a 1.47-fold increased risk of PAH (adjusted OR=1.47, 95% CI=1.18–1.83; p=0.001), compared to the rs833061TT genotype. Furthermore, regarding the additive genetic mode that best fit the genetic model as indicated by the smallest AIC value among all assumed models, the C variants conferred a harmful dose-effect on PAH risk (adjusted OR=1.39, 95% CI=1.15–1.68; p=0.0003). However, there was no significant difference between the patients and controls in genotype frequencies for the four other SNPs studied.
We further analyzed the effect of rs833061T>C genotype on VEGF gene expression. The mRNA expressions of VEGF in 40 PBMCs were significantly higher in the PAH patients than in the healthy controls (p=0.014) (Fig. 1C and D). In addition, the PBMCs with rs833061C variant genotypes from PAH patients had a much higher VEGF expression level than those with rs833061TT genotype (p=0.021). These results indicated that the rs833061T>C genotype plays a pivotal role in the regulation of VEGF gene expression.
To the best of our knowledge, this is the first study to provide evidence demonstrating an association between genetic variants in the VEGF gene and PAH risk in Chinese individuals. We evaluated the relationships among five common SNPs in the VEGF gene in patients with PAH and found that the rs833061T>C SNP in the VEGF gene promoter region was significantly associated with an increased risk of PAH. Bioassays results further indicated that rs833061C variants could promote the expression of VEGF.
A great deal of information has shown that PAH is a complex, multifactorial condition that involves diverse biochemical pathways and cell types leading to alterations in vascular structure, vessel reactivity, and interactions of the vessel wall with circulating blood elements.181920 Among its complex pathophysiological pathways, vascular remodeling places a crucial role in PAH initiation.21 In addition, studies have demonstrated that the VEGF signaling pathway is closely involved in vascular remodeling activities.21222324 Zhang, et al.23 reported that VEGF contributes to vascular remodeling in human arteries via a direct effect on human T cells, enhancing their recruitment to the vessel. Lee, et al.25 demonstrated that VEGF could induce vascular and extravascular remodeling and enhance TH2-mediated sensitization and inflammation in the lungs. Abnormal expression of VEGF has been identified to be correlated with various human diseases:2627 for example, one study proposed the up-regulation of VEGF indirectly provided the necessary oxygen and nutrients for tumor growth, and therefore facilitates tumour invasion and metastasis.28 Another study suggests that VEGF elicits the release of nitric oxide via the activation of tyrosine kinase receptors, thereby inducing coronary artery disease (CAD), suggesting important implications regarding the efficacy of endogenous and exogenous VEGF in patients with risk factor for CAD.29 A number of studies have identified the gene expression of PBMCs to occur efficiently and specifically in patients with PAH.30 In this study, VEGF expression in PBMCs was also tested, and patients with PAH had higher expression of VEGF than healthy controls. This finding agreed with previous data that suggested that breath condensate levels of VEGF are elevated in patients with PAH, compared to healthy volunteers.11
The VEGF gene has been directly implicated in angiogenesis. The development of PAH is associated with active vascular remodeling involving vascular growth. Evidence suggests that increased expression of VEGF by PBMCs might accelerate propagation of endothelial cells and development of microvessels, thus leading to the vascular remodeling.31 Therefore, our findings suggest that abnormal levels of VEGF might play an important role in the pathogenesis of PAH.
A previous reports suggested conspicuous links between VEGF polymorphisms and human diseases disk.32 This report also assessed the link between common SNPs in VEGF with PAH risk. The results indicated that a locus alteration from T to C of the rs833061 polymorphism in the VEGF gene promoter region increased the risk for developing PAH. To interpret these results, a series of biomedical experiments were undertaken and found that rs833061C variants could promote the transcriptional activity of the VEGF gene and increase VEGF expression. For the rs833061T<C polymorphism, several reports also verified its association with other diseases. Jung, et al.33 demonstrated that rs833061T<C was associated with the development of recurrent implantation failure in Korean women. Another systematic review outlined significant correlations of rs833061T<C with the risk of diabetic nephropathy.34 In light thereof, we could postulate that the rs833061T>C SNP in the promoter of the VEGF gene confers an increased risk for PAH via a molecular mechanism of rs833061C variants elevating the expression of VEGF.
There were several limitations in this study that should be addressed. Firstly, the moderate sample size might reduce the statistical power with which to detect small effects. Secondly, all subjects were recruited consecutively from hospitals; therefore, selection bias could not be avoided. Finally, this study was performed on a Chinese population, and caution should be exercised when extrapolating the data to other regions and ethnic groups. The genotype distribution of selected SNP in controls in this study was in accordance with Hardy-Weinberg expectations. In addition, using Power and Sample Size Calculation (PS, version 3.0; http://biostat.mc.vanderbilt.edu/twiki/bin/view/Main/PowerSampleSize) to considerer the relevance between the rs833061T>C polymorphism and PAH risk, we obtained 86.3% statistical power in our findings. Furthermore, this association was supported by additional functional experiments.
In conclusion, this hospital-based, retrospective study indicated that the potentially functional SNP rs833061T>C in the VEGF gene promoter region could be associated with an increased risk of PAH in the Chinese Han population. This study also suggested that this polymorphism is functional and influences the susceptibility to PAH by altering gene expression levels. Accordingly, this may be a novel biomarker for PAH. Larger studies in different population groups are needed to corroborate these results.
Figures and Tables
Fig. 1
Effects of rs833061T>C SNP on VEGF expression. (A) Graphic illustration of the reporter constructs comprising the VEGF gene promoter with 2475 bp containing rs833061T or C allele. (B) Luciferase expression levels of different VEGF promoter constructs in PASMCs. The Renilla luciferase activity of each reporter was normalized against the internal control of firefly luciferase. Columns, mean from three separate assays; bars, standard deviation. (C) Comparison of relative mRNA levels in PBMCs between patients and healthy controls. (D) Relative mRNA expression of the VEGF gene by rs833061T>C genotypes. VEGF, vascular endothelial growth factor; PASMCs, pulmonary artery smooth muscle cells; PBMCs, peripheral blood mononuclear cells; SNP, single nucleotide polymorphism.
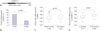
Table 1
Baselines Information between PAH Patients and Healthy Controls
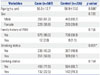
Table 2
Associations between VEGF Polymorphisms and PAH Risk (continued)
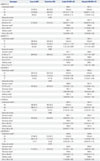
VEGF, vascular endothelial growth factor; PAH, pulmonary arterial hypertension; OR, odds ratio; CI, confidence interval.
*Statistically significant, p<0.05, †Models adjusted for surrounding factors including age, sex, smoke, drink, and history of PAH, ‡Akaike information criterion (AIC) value in the logistic regression analysis.
ACKNOWLEDGEMENTS
This study was supported by the Scientific Foundation of Science and Technology Department of Guangdong Province, China (grants 2012A030400033) and the Science and Technology Program of Guangdong Province (grants 2016A020215014).
References
1. Sitbon O, Morrell N. Pathways in pulmonary arterial hypertension: the future is here. Eur Respir Rev. 2012; 21:321–327.


2. Wilcox SR, Kabrhel C, Channick RN. Pulmonary hypertension and right ventricular failure in emergency medicine. Ann Emerg Med. 2015; 66:619–628.


3. McLaughlin V. Managing pulmonary arterial hypertension and optimizing treatment options: prognosis of pulmonary artery hypertension. Am J Cardiol. 2013; 111:8 Suppl. 10C–15C.


4. Park YM, Chung WJ, Choi DY, Baek HJ, Jung SH, Choi IS, et al. Functional class and targeted therapy are related to the survival in patients with pulmonary arterial hypertension. Yonsei Med J. 2014; 55:1526–1532.


6. Guru SK, Pathania AS, Kumar S, Ramesh D, Kumar M, Rana S, et al. Secalonic acid-D represses HIF1α/VEGF-mediated angiogenesis by regulating the Akt/mTOR/p70S6K signaling cascade. Cancer Res. 2015; 75:2886–2896.


7. Massena S, Christoffersson G, VÅgesjö E, Seignez C, Gustafsson K, Binet F, et al. Identification and characterization of VEGF-A-responsive neutrophils expressing CD49d, VEGFR1, and CXCR4 in mice and humans. Blood. 2015; 126:2016–2026.


8. Fearnley GW, Bruns AF, Wheatcroft SB, Ponnambalam S. VEGF-A isoform-specific regulation of calcium ion flux, transcriptional activation and endothelial cell migration. Biol Open. 2015; 4:731–742.


9. Khayati F, Pérez-Cano L, Maouche K, Sadoux A, Boutalbi Z, Podgorniak MP, et al. EMMPRIN/CD147 is a novel coreceptor of VEGFR-2 mediating its activation by VEGF. Oncotarget. 2015; 6:9766–9780.


10. Smadja DM, Levy M, Huang L, Rossi E, Blandinières A, Israel-Biet D, et al. Treprostinil indirectly regulates endothelial colony forming cell angiogenic properties by increasing VEGF-A produced by mesenchymal stem cells. Thromb Haemost. 2015; 114:735–747.


11. Seyfarth HJ, Sack U, Gessner C, Wirtz H. [Angiogenin, bFGF and VEGF: angiogenic markers in breath condensate of patients with pulmonary hypertension]. Pneumologie. 2015; 69:207–211.


12. Vannay A, Vásárhelyi B, Környei M, Treszl A, Kozma G, Györffy B, et al. Single-nucleotide polymorphisms of VEGF gene are associated with risk of congenital valvuloseptal heart defects. Am Heart J. 2006; 151:878–881.


13. Smedts HP, Isaacs A, de Costa D, Uitterlinden AG, van Duijn CM, Gittenberger-de Groot AC, et al. VEGF polymorphisms are associated with endocardial cushion defects: a family-based case-control study. Pediatr Res. 2010; 67:23–28.


14. Han X, Liu L, Niu J, Yang J, Zhang Z, Zhang Z. Association between VEGF polymorphisms (936c/t, -460t/c and -634g/c) with haplotypes and coronary heart disease susceptibility. Int J Clin Exp Pathol. 2015; 8:922–927.
15. Sitbon O, Humbert M, Jaïs X, Ioos V, Hamid AM, Provencher S, et al. Long-term response to calcium channel blockers in idiopathic pulmonary arterial hypertension. Circulation. 2005; 111:3105–3111.


16. Schmittgen TD, Livak KJ. Analyzing real-time PCR data by the comparative C(T) method. Nat Protoc. 2008; 3:1101–1108.


17. Uh HW, Mertens BJ, Jan van der Wijk H, Putter H, van Houwelingen HC, Houwing-Duistermaat JJ. Model selection based on logistic regression in a highly correlated candidate gene region. BMC Proc. 2007; 1:Suppl 1. S114.


18. Humbert M, Morrell NW, Archer SL, Stenmark KR, MacLean MR, Lang IM, et al. Cellular and molecular pathobiology of pulmonary arterial hypertension. J Am Coll Cardiol. 2004; 43:12 Suppl S. 13S–24S.


19. Soubrier F, Chung WK, Machado R, Grünig E, Aldred M, Geraci M, et al. Genetics and genomics of pulmonary arterial hypertension. J Am Coll Cardiol. 2013; 62:25 Suppl. D13–D21.


20. de Jesus Perez VA. Molecular pathogenesis and current pathology of pulmonary hypertension. Heart Fail Rev. 2016; 21:239–257.


21. Guignabert C, Tu L, Girerd B, Ricard N, Huertas A, Montani D, et al. New molecular targets of pulmonary vascular remodeling in pulmonary arterial hypertension: importance of endothelial communication. Chest. 2015; 147:529–537.


22. Morin-Brureau M, Lebrun A, Rousset MC, Fagni L, Bockaert J, de Bock F, et al. Epileptiform activity induces vascular remodeling and zonula occludens 1 downregulation in organotypic hippocampal cultures: role of VEGF signaling pathways. J Neurosci. 2011; 31:10677–10688.


23. Zhang J, Silva T, Yarovinsky T, Manes TD, Tavakoli S, Nie L, et al. VEGF blockade inhibits lymphocyte recruitment and ameliorates immune-mediated vascular remodeling. Circ Res. 2010; 107:408–417.


24. Li Y, Zhu H, Klausen C, Peng B, Leung PC. Vascular endothelial growth factor-A (VEGF-A) mediates activin A-induced human trophoblast endothelial-like tube formation. Endocrinology. 2015; 156:4257–4268.


25. Lee CG, Link H, Baluk P, Homer RJ, Chapoval S, Bhandari V, et al. Vascular endothelial growth factor (VEGF) induces remodeling and enhances TH2-mediated sensitization and inflammation in the lung. Nat Med. 2004; 10:1095–1103.


26. Gnudi L, Benedetti S, Woolf AS, Long DA. Vascular growth factors play critical roles in kidney glomeruli. Clin Sci (Lond). 2015; 129:1225–1236.


27. Kieran MW, Kalluri R, Cho YJ. The VEGF pathway in cancer and disease: responses, resistance, and the path forward. Cold Spring Harb Perspect Med. 2012; 2:a006593.


28. Baker GJ, Yadav VN, Motsch S, Koschmann C, Calinescu AA, Mineharu Y, et al. Mechanisms of glioma formation: iterative perivascular glioma growth and invasion leads to tumor progression, VEGF-independent vascularization, and resistance to antiangiogenic therapy. Neoplasia. 2014; 16:543–561.


29. Métais C, Li J, Li J, Simons M, Sellke FW. Effects of coronary artery disease on expression and microvascular response to VEGF. Am J Physiol. 1998; 275(4 Pt 2):H1411–H1418.
30. Bull TM, Coldren CD, Geraci MW, Voelkel NF. Gene expression profiling in pulmonary hypertension. Proc Am Thorac Soc. 2007; 4:117–120.


31. Hojo Y, Ikeda U, Zhu Y, Okada M, Ueno S, Arakawa H, et al. Expression of vascular endothelial growth factor in patients with acute myocardial infarction. J Am Coll Cardiol. 2000; 35:968–973.


32. García-Closas M, Malats N, Real FX, Yeager M, Welch R, Silverman D, et al. Large-scale evaluation of candidate genes identifies associations between VEGF polymorphisms and bladder cancer risk. PLoS Genet. 2007; 3:e29.

