This article has been corrected. See "Erratum to “Detection of Circulating Tumor Cells in Breast Cancer Patients Using Cytokeratin-19 Real-Time RT-PCR” by Park HS, et al. (Yonsei Med J 2017;58:19-26.)" in Volume 58 on page 473.
Abstract
Purpose
The roles of circulating tumor cells (CTCs) as predictive and prognostic factors, as well as key mediators in the metastatic cascade, have been investigated. This study aimed to validate a method to quantify CTCs in peripheral blood using a real-time reverse transcriptase polymerase chain reaction (RT-PCR) assay for cytokeratin (CK)-19 and to evaluate the utility of this assay in detecting CTCs in breast cancer patients.
Materials and Methods
Real-time monitoring PCR of fluorescently labeled specific hybridization probes for CK-19 mRNA was established. Peripheral blood samples from 30 healthy donors, 69 patients with early breast cancer, 47 patients with locally advanced breast cancer, and 126 patients with metastatic breast cancer were prospectively obtained and analyzed for CTC detection.
Results
CK-19 mRNA was not detectable in healthy subjects using the real-time RT-PCR method. The detection rates of CK-19 mRNA in breast cancer patients were 47.8% for early breast cancer (33/69), 46.8% for locally advanced breast cancer (22/47), and 61.1% for metastatic breast cancer (77/129). The detection rate of CK-19-positive CTCs in metastatic disease was slightly higher than early or locally advanced breast cancer; however, the detection rate according to disease burden was not statistically different (p=0.097). The detection rate was higher in patients with pleural metastasis (p=0.045). CTC detection was associated with poor survival (p=0.014).
The early spread of tumor cells among breast cancer patients is usually undetectable, even by conventional pathologic analyses and high-resolution imaging technologies. With highly sensitive immunocytochemical and molecular assays, it is possible to detect occult metastatic tumor cells at the single-cell stage in peripheral blood, regional lymphatic nodes, and bone marrow before incurable distant metastases occur.1 Circulating tumor cells (CTCs) circulate in the peripheral blood of patients. CTCs can originate from both the primary tumor and its metastases. It is believed that CTCs mediate the hematogenic spread of cancer to distant organs, including bone, lung, brain, and liver. CTCs have potential to form metastases, and for this reason, they can be useful predictors of poor outcomes, such as shorter disease-free and overall survival.234 Furthermore, checking CTC levels may be valuable for follow-up evaluation and to monitor therapeutic efficacy.1567
Immunocytochemistry using antibodies against proteins, which are not expressed in mesenchymal cells, but in epithelial cells, is commonly used for the detection of CTCs. In recent studies, reverse transcriptase polymerase chain reaction (RT-PCR) and quantitative real-time RT-PCR methods have shown higher diagnostic sensitivity.8910 The development of real-time RT-PCR technology has allowed an enormous progress in cancer diagnostics, providing significant and quantitative data about gene expression in an automated, fast, flexible, affordable, and cost-effective way. Real-time RT-PCR has recently been used to detect CTCs in the peripheral blood and lymph nodes in patients with metastatic breast cancer through the detection of differential expression of marker genes, which are achieved by multigene RT-PCR assays, respectively.1011
In this study, we report an adaptation and analytical validation of a real-time quantitative RT-PCR methodology for cytokeratin (CK)-19 mRNA using the LightCycler system.812 CK-19 is a typical epithelial marker, which is present in epithelial cells, but is not found in mesenchymal cells. It has been investigated extensively as a potential marker to detect CTCs in early and metastatic breast cancer.310131415161718192021222324 Among the numerous molecular markers currently being used to detect occult micrometastasis indirectly in patients with breast cancer, detecting CK-19 mRNA expression is considered one of the most promising methods to detect CTCs, due to its high sensitivity and specificity.11
The human breast cancer cell lines including MCF-7, SK-BR-3, and MDA-MB-231 (obtained from the Korean Cell Line Bank, Seoul, Korea), which contain the CK-19 gene, were used as positive controls. Each of the cell lines was cultured in Dulbecco's modified eagle medium containing 10% fetal bovine serum, 100 U/mL penicillin, and 100 µg/mL streptomycin (HyClone, Logan, UT, USA).
From October 2010 until February 2012, a total of 242 consecutive patients with breast cancer (median age 53 years, 26–83 years), including 69 patients with early breast cancer (stage I–II, 28.5%), 47 patients with locally advanced breast cancer (19.4%), and 126 patients with metastatic disease (stage IV, 52.1%), were enrolled to identify detection rates of CTCs according to tumor burden. To evaluate clinical relevance, blood samples were collected after surgery or before adjuvant therapy in early breast cancer patients, at initial diagnosis in locally advanced breast cancer patients and at implementation of therapy or the regimen change for disease progression in metastatic patients. All patients with locally advanced breast cancer received neoadjuvant chemotherapy before surgery.
To serve as controls, 30 healthy female donors were recruited (median age 47 years, 30–54 years) and blood samples were obtained. Peripheral blood (12.5 mL in ethylenediaminetetraacetic acid) was obtained from healthy volunteers and breast cancer patients. The blood samples were conditioned at 4℃ for different time intervals and processed within a maximum of 24 hours after the samples were drawn. To avoid epithelial contamination by the skin, the first 5 mL of each blood sample was discarded. Prior to participation in this study, patients and healthy donors provided informed consent, approved by the Ethics and Scientific Committees of our institution (IRB ID number=4-2011-0011).
Isolation of total RNA was processed using the Trizol LS reagent (Gibco-BRL, Carlsbad, CA, USA) according to the manufacturer's instructions. All RNA preparation and handling procedures were performed in a laminar flow hood under RNase-free conditions. Isolated RNA was dissolved in RNA storage buffer (Life Technologies, Carlsbad, CA, USA) and stored at -80℃ for further processing. The RNA concentration was evaluated by absorbance readings at 260 nm using a NanoDrop spectrophotometer (Thermo Scientific, Fremont, CA, USA). RNA integrity was tested by PCR amplification of the glyceraldehyde 3-phosphate dehydrogenase (GAPDH) housekeeping gene. Reverse transcription of RNA was carried out with the Omniscript Reverse Transcription RT kit (QIAGEN, Hilden, Germany). Total RNA prepared from the MCF-7 cell line was used as a positive control. cDNA was synthesized from 5 µg of total RNA isolated from peripheral blood mononuclear cells of healthy donors and breast cancer patients, according to the manufacturer's instructions.
Quantification is based on real-time monitoring during PCR of fluorescently labeled specific probes for CK-19. Real-time RT-PCR for CK-19 mRNA was performed using the LightCycler system 480 (Roche, Penzberg, Upper Bavaria, Germany). Real-time RT-PCR was conducted in a total volume of 20 µL, containing 2 µL of cDNA, 0.3 µg of the CK-19 forward primer (10 pM), 0.3 µL of the reverse primer CK-19 (10 pM), 0.3 µL of the probe CK-19 (10 pM), and 10 µL of LightCycler 480 Probes Master mix reagent (2X, Roche, Penzberg, Upper Bavaria, Germany). Finally, diethylpyrocarbonate (DEPC)-H2O was added to the reaction mix. All primers and hybridization probe sequences are listed in Table 1.
PCR reaction was initiated after a 10-minute denaturation at 95℃, and then the cycling protocol, consisting of denaturation at 95℃ for 10 seconds, and annealing at 60℃ for 30 seconds, was repeated 50 times. Fluorescence detection was performed at the end of each annealing step for 0.25 second.
For quantification, an external standard curve was applied using cDNA from MCF-7 cells, which was described in previous studies.812 Total RNA was obtained from 1×106 MCF-7 cells through serial dilution.812 This cDNA was used as the CK-19-positive control. This standard curve was created by plotting the number of MCF-7 cells corresponding to each value of its Ct.812
The proposed real-time RT-PCR methodology was applied in a total of 272 peripheral blood samples collected from 30 healthy female donors, 69 patients with early breast cancer after surgery or before adjuvant therapy, 47 patients with locally advanced breast cancer, and 126 patients with verified metastasis before regimen change or first-line chemotherapy.
The amount of total RNA in each sample was used to normalize our real-time RT-PCR data. We adopted the previous detection limit method to set up the cutoff of CTC detection.8 The presence of ≥0.792 MCF-7 cell equivalents/5 µg RNA in the patient blood sample was considered a positive result on the basis of the analytical detection limit. According to this cutoff, the specificity of the proposed method was evaluated by analyzing peripheral blood samples from 30 female healthy donors.
Real-time RT-PCR data was quantified as Ct values that are inversely related to the amount of starting template: high Ct values were correlated with low levels of gene expression, whereas low Ct values were correlated with high levels of gene expression. Results were normalized to an internal control reference gene. If the mean Ct value for CK-19 mRNA was higher or equal to 36, the CK-19 expression was considered undetectable. To define the baseline levels of CK-19 expression and thresholds for CK-19 positivity, 30 specimens of peripheral blood mononuclear cells obtained from female healthy donors with no evidence of malignancy were analyzed. The molecular analysis was blinded to clinical outcome and patient clinicopathological data.
Tumor stage was based on the American Joint Committee on Cancer Staging manual 6th edition. Histological grade was assessed based on the modified Bloom-Richardson classification. Tumors with ≥1% nuclear-stained cells were considered to have estrogen receptor (ER) and progesterone receptor (PR) positivity. Human epidermal growth factor receptor (HER2) overexpression was considered positive in cases with an immunohistochemistry (IHC) score of 3+ or gene amplification by fluorescence in situ hybridization with an IHC score of 2+.25 Breast cancer subtypes, based on ER, PR, and HER2, were categorized into three subtypes: those that were ER- and/or PR-positive were classified as the luminal subtype; those that were ER- and PR-negative and HER2-positive were classified as the HER2 subtype; and those that were negative for all markers were classified as triple-negative breast cancer.
SPSS version 20.0 (IBM, Armonk, NY, USA) statistical software was used for the analysis of pathological and molecular outcome. Chi-square tests were conducted to explore the association between pre-defined baseline categorical covariates and real-time RT-PCR positivity/negativity status. t-test or ANOVA was used to compare continuous variables. Pre-defined baseline covariates were age, TNM stage, histological grade, ER status, PR status, and HER2 status. Statistical significance was defined as p values<0.05. All tests were two-sided.
The real-time RT-PCR method based on CK-19 mRNA showed 100% specificity, because none of the 30 blood samples from healthy donors had detectable CK-19 mRNA positive cells.
In 69 patients with early breast cancer, CK-19 mRNA positive cells were detected in 33 patients (47.8%). Among the 47 patients with locally advanced breast cancer, 22 (46.8%) patients were found to be positive prior to neoadjuvant chemotherapy. CK-19 mRNA positive cells were detected in 77 (61.1%) patients among the 126 patients with distant metastasis. The Ct values observed in patients with breast cancer who had detectable CK-19 mRNA, classified according to treatment groups, are illustrated in Fig. 1. There was no significant difference in terms of Ct values according to the treatment group (p=0.164) (Fig. 1).
The mean age of all patents was 52.1±10.4 years. Mean ages according to tumor burden were 53.1±9.7 years in patients with early breast cancer, 50.1±11.4 years in patients with locally advanced cancer, and 52.3±10.4 years in patients with distant metastasis. There were no significant differences in age according to tumor burden.
The distribution of patient demographic and clinicopathological characteristics is shown in Table 2. The rates of CTC-positive cells were not different between patients younger than 50 years and those aged 50 years and older. Patients with distant metastasis had a CTC detection rate of 61.1% in their blood samples; meanwhile, patients with early and locally advanced breast cancer had detection rates of 47.8% and 46.8%, respectively. CTCs were more often observed in ER-negative tumors than in ER-positive tumors (60.8% vs. 51.0%, respectively). In terms of breast cancer subtypes, CTCs were more commonly detected in patients with TNBC than other subtypes (63.3% vs. 47.5–55.0%). All clinicopathological features were not significantly associated with the detection of CTCs, as defined by CK-19 mRNA positive cells (Table 2).
Table 3 shows the detection rates of CK-19 mRNA-positive cells according to metastatic sites in patients with distant metastasis. CTCs were more frequently identified in patients with visceral metastasis than in those with non-visceral metastasis (61.5% vs. 58.6%); however, this difference was not significant (p=0.784). Patients with pleural metastasis demonstrated significantly higher CTC detection than those without pleural metastasis (p=0.04) All other metastatic sites were not associated with CK-19 mRNA-positive CTCs.
Overall survival curves according to CK-19 mRNA-positive cells are shown in Fig. 2. With a median follow-up period of 29 months (range, 0–44 months), survival was poorer in patients positive for CTCs than in those without detectable CTCs, and the difference was significant (p=0.014). In multivariate analysis adjusting for age, CTC detection, and tumor burden, only metastatic tumor burden was significantly associated with overall survival [p<0.001, hazard ratio (HR)=27.014, 95% confidence interval (CI)=8.524–85.617]. Binary CTC detection (yes or no) was not a significant prognostic factor for overall survival in multivariate analysis (p=0.097, HR=0.700, 95% CI=0.459–1.067) (Table 4).
CTCs are epithelial tumor cells detected in the peripheral blood of patients with solid tumors using mainly immunocytochemical approaches including CellSearch (Veridex LLC, Raritan, NJ, USA) and AdnaTest (AdnaGen AG, Langenhagen, Germany).11026 CellSearch is the only technology for CTC detection that has been admitted by the Food and Drug Administration as an aid in monitoring patients with metastatic breast, colorectal, and prostate cancers. However, CellSearch has a limitation that is inherent in its immunomagnetic enrichment system. EpCAM-negative CTCs can be excluded during the test using CellSearch, because CellSearch is based on the magnetic detection of EpCAM-positive cells. Some investigators have pointed out heterogeneous expression of EpCAM in breast cancer and down-regulation of EpCAM after chemotherapy.27 Thus, detection systems that use RT-PCR for CK or multiple markers that can identify the presence of CTCs, regardless of EpCAM expression, have been investigated in several studies.81213142224 Compared to the CellSearch immunomagnetic enrichment system, RT-PCR for CK or other multimarkers exhibits superior sensitivity.10 In this study, CK-19 mRNA expression was detected in more than half of patients with metastatic disease and about half of patients with early breast cancer, and these results were similar to those of a previous study.14 The detection rate of CTCs using the Cellsearch system was about 20–30% in patients with breast cancer.1028 The detection rate of RT-PCR for CK-19 mRNA expression in the current study was almost two-fold higher than that of CellSearch. However, there was no standardized technique for detecting CK-19 mRNA-positive CTCs among previous studies that used CK-19 mRNA expression to report on the presence of CTCs, and considerable heterogeneity of detection methods existed in previous studies.81112 Therefore, caution is required in the interpretation and application of RT-PCR for the detection of CK-19 mRNA expression.
The relationships observed between the presence of CTCs and clinicopathological parameters were different in different studies due to heterogeneity of the study design.11131420222326 The meta-analysis conducted by Zhao, et al.11 revealed that CTC-positive tumors were associated with biologically aggressive phenotypes; on the other hand, CK-19 mRNA-positive breast cancers were not. Several studies also reported no relation between the presence of CK-19 mRNA-positive CTCs and clinicopathological parameters, including age, tumor size, ER, PR, HER2, and grade,1314202223 and only the tumor burden was related to presence of CTCs.1322 Previous studies reported that more CTCs were detected in metastatic disease than in early disease.1322 Our study showed no relationship between the presence of CK-19 mRNA-positive CTCs and clinicopathological parameters with the exception of pleural metastasis, and this is partially concordant with a previous study.29 The lack of agreement between the current study and previous studies in the literature may be due to the difference in defining CTCs and the lack of standardized techniques for RT-PCR of CK-19 mRNA.
The current study demonstrated that patients with CK-19 mRNA CTC detection showed poorer survival than those without detection in univariate analyses; however, this did not hold up on multivariate analyses. Stathopoulou, et al.14 suggested that CTC detection by CK-19 mRNA expression is related to poor survival. Benoy, et al.22 also reported that elevated CK-19 in bone marrow is prognostic; however, the peripheral CK-19 level is not. Although there are some similarities in detection techniques between previous and current studies, the prognostic values of CTC detection using CK-19 mRNA expression varies among studies. This discordance among studies may be due to heterogeneity in the study design: the Greek study mainly included metastatic breast cancer,14 whereas the Belgian study enrolled more patients with early breast cancer than with metastatic breast cancer.26 We enrolled 129 patients with metastatic breast cancer; this constituted about half (52.1%) of all patients. These discrepancies among the studies may have caused the difference in outcomes. Recently, a large prospective multicenter study confirmed that CTC detection by the CellSearch system in patients with early breast cancer is also clinically relevant and prognostic.28 In the current study, it was not possible to analyze patients with early and advanced breast cancer using subgroup analyses, due to the short follow-up duration. Therefore, further studies with longer follow-up periods are needed for the patients with early breast cancer in this cohort.
There are some limitations of this study. First, due to the retrospective nature of the study and its sample size, selection bias cannot be ruled out. Pleural metastases were frequently found in the CTC-positive diseases in the current study. However, the cases of pleural metastases were too few to make solid conclusions, and thus, further studies are needed. Second, the absence of a consensus for CTC definition or threshold using RT-PCR methods makes it difficult to apply the results of the study to clinical settings. Different definitions and thresholds among studies can have an influence on reproducibility of the clinical role of CTCs in breast cancer. Third, the sole use of CK-19 mRNA expression for the detection of CTCs in the current study could be a limitation of the study, because the use of a single marker for the detection of CTCs may not be solely representative of various types of CTCs that may be defined by the presence of other CK family proteins, such as CK-8 and -18, and because the use of CK-family as CTC markers can enhance the detection rate of CTCs.3031 A previous study indicates that RT-PCR for multiple markers, including HER2 and MUC1, which are closely related to the progression or development of breast cancer, can provide more prognostic or predictive information.32 Furthermore, epithelial-messenchymal transition markers and stem cell markers can increase in CTCs.33 Therefore, further study using RT-PCR for multiple markers is needed to extend our knowledge of CTCs. Finally, recent genomic, morphological, and cultural analyses including single-cell sequencing, CTC clusters, and conditionally reprogrammed cells using CTCs cannot be accessed using RT-PCR for CK-19 mRNA-positive CTCs.343536 Thus, new technology that can isolate morphological CTCs has been introduced to overcome these limitations.37 Further studies using the enhanced technology may shed light on the role of complex behaviors of CTCs in tumor biology.
In conclusion, we adapted and validated a detection method for CTCs using RT-PCR for CK-19 mRNA expression. The current study suggested that CTC detection using RT-PCR for CK-19 mRNA expression may have a clinical impact on overall survival in patients with breast cancer. Even though clinicopathological parameters, including age, tumor size, nodal status, ER, PR, HER2, and the grade of the primary tumor, which are all known prognostic factors of breast cancer, were not related to CTC detection using RT-PCR for CK-19 mRNA expression, with the exception of pleural metastasis, breast cancer patients in which CTCs were detected showed poor overall survival in univariate analysis. Further studies are needed to clarify the clinical role of the RT-PCR approach for CTC detection in the peripheral blood of patients with breast cancer.
Figures and Tables
Fig. 1
Ct values according to treatment setting in patients with CTCs. High Ct values correlated with low levels of gene expression, whereas low Ct values correlate with high levels of gene expression. The box plots represent the median, maximal, and minimal number of Ct values. There was no significant difference in terms of Ct values among three groups (p=0.16). CTC, circulating tumor cell; EBC, early breast cancer; LBC, locally advanced breast cancer; MBC, metastatic breast cancer.
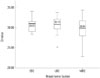
Fig. 2
Overall survival according to the detection of CTCs defined by CK-19 mRNA-positive cells. CTC, circulating tumor cell; CK, cytokeratin.

Table 1
Sequences of Primers and Hybridization Probes Used in This Study

Table 2
Patient Demographics according to the Detection of CTCs, Defined as CK-19 mRNA-Positive Cells

Table 3
Metastatic Sites according to the Detection of CTCs, Defined as CK-19 mRNA-Positive Cells
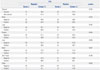
Table 4
Multivariate Analysis according to the Detection of CTC, Defined as CK-19 mRNA-Positive Cells
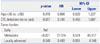
ACKNOWLEDGEMENTS
This research was supported by Basic Science Research Program through the National Research Foundation of Korea (NRF) funded by the Ministry of Education, Science and Technology (NRF-2013R1A1A2011600). This research was supported by a grant of the Korea Health Technology R&D Project through the Korea Health Industry Development Institute (KHIDI), funded by the Ministry of Health & Welfare, Republic of Korea (grant number: HI14C1234). This research was supported by a grant of the Korea Health Technology R&D Project through the Korea Health Industry Development Institute (KHIDI), funded by the Ministry of Health & Welfare, Republic of Korea (HI3C2162). This work was supported by the National Research Foundation of Korea (NRF) grant funded by the Korea government (MSIP) (No. 2015R1A2A2A04006450). This work was supported by the Industrial Core Technology Development Program (10054699, Phase Ib, II Study for Chemotherapy Cure Rate Improvement in Breast Cancer Patients by Activation of RIP3 Expression) funded by the Ministry of Trade, Industry & Energy(MOTIE, Korea). This study was supported by a faculty research grant of Yonsei University College of Medicine for 2011(6-2011-0108).
References
1. Fehm T, Müller V, Alix-Panabières C, Pantel K. Micrometastatic spread in breast cancer: detection, molecular characterization and clinical relevance. Breast Cancer Res. 2008; 10:Suppl 1. S1.


2. Cristofanilli M, Budd GT, Ellis MJ, Stopeck A, Matera J, Miller MC, et al. Circulating tumor cells, disease progression, and survival in metastatic breast cancer. N Engl J Med. 2004; 351:781–791.


3. Xenidis N, Ignatiadis M, Apostolaki S, Perraki M, Kalbakis K, Agelaki S, et al. Cytokeratin-19 mRNA-positive circulating tumor cells after adjuvant chemotherapy in patients with early breast cancer. J Clin Oncol. 2009; 27:2177–2184.


4. Cristofanilli M, Hayes DF, Budd GT, Ellis MJ, Stopeck A, Reuben JM, et al. Circulating tumor cells: a novel prognostic factor for newly diagnosed metastatic breast cancer. J Clin Oncol. 2005; 23:1420–1430.


5. Dawood S, Broglio K, Valero V, Reuben J, Handy B, Islam R, et al. Circulating tumor cells in metastatic breast cancer: from prognostic stratification to modification of the staging system? Cancer. 2008; 113:2422–2430.


6. Pantel K, Alix-Panabières C, Riethdorf S. Cancer micrometastases. Nat Rev Clin Oncol. 2009; 6:339–351.


7. Paterlini-Brechot P, Benali NL. Circulating tumor cells (CTC) detection: clinical impact and future directions. Cancer Lett. 2007; 253:180–204.


8. Stathopoulou A, Gizi A, Perraki M, Apostolaki S, Malamos N, Mavroudis D, et al. Real-time quantification of CK-19 mRNA-positive cells in peripheral blood of breast cancer patients using the lightcycler system. Clin Cancer Res. 2003; 9:5145–5151.
9. Ring AE, Zabaglo L, Ormerod MG, Smith IE, Dowsett M. Detection of circulating epithelial cells in the blood of patients with breast cancer: comparison of three techniques. Br J Cancer. 2005; 92:906–912.


10. Van der Auwera I, Peeters D, Benoy IH, Elst HJ, Van Laere SJ, Prové A, et al. Circulating tumour cell detection: a direct comparison between the CellSearch System, the AdnaTest and CK-19/mammaglobin RT-PCR in patients with metastatic breast cancer. Br J Cancer. 2010; 102:276–284.


11. Zhao S, Liu Y, Zhang Q, Li H, Zhang M, Ma W, et al. The prognostic role of circulating tumor cells (CTCs) detected by RT-PCR in breast cancer: a meta-analysis of published literature. Breast Cancer Res Treat. 2011; 130:809–816.


12. Stathopoulou A, Ntoulia M, Perraki M, Apostolaki S, Mavroudis D, Malamos N, et al. A highly specific real-time RT-PCR method for the quantitative determination of CK-19 mRNA positive cells in peripheral blood of patients with operable breast cancer. Int J Cancer. 2006; 119:1654–1659.


13. Kahn HJ, Yang LY, Blondal J, Lickley L, Holloway C, Hanna W, et al. RT-PCR amplification of CK19 mRNA in the blood of breast cancer patients: correlation with established prognostic parameters. Breast Cancer Res Treat. 2000; 60:143–151.


14. Stathopoulou A, Vlachonikolis I, Mavroudis D, Perraki M, Kouroussis Ch, Apostolaki S, et al. Molecular detection of cytokeratin-19-positive cells in the peripheral blood of patients with operable breast cancer: evaluation of their prognostic significance. J Clin Oncol. 2002; 20:3404–3412.


15. Xenidis N, Vlachonikolis I, Mavroudis D, Perraki M, Stathopoulou A, Malamos N, et al. Peripheral blood circulating cytokeratin-19 mRNA-positive cells after the completion of adjuvant chemotherapy in patients with operable breast cancer. Ann Oncol. 2003; 14:849–855.


16. Xenidis N, Perraki M, Kafousi M, Apostolaki S, Bolonaki I, Stathopoulou A, et al. Predictive and prognostic value of peripheral blood cytokeratin-19 mRNA-positive cells detected by real-time polymerase chain reaction in node-negative breast cancer patients. J Clin Oncol. 2006; 24:3756–3762.


17. Xenidis N, Markos V, Apostolaki S, Perraki M, Pallis A, Sfakiotaki G, et al. Clinical relevance of circulating CK-19 mRNA-positive cells detected during the adjuvant tamoxifen treatment in patients with early breast cancer. Ann Oncol. 2007; 18:1623–1631.


18. Ignatiadis M, Perraki M, Apostolaki S, Politaki E, Xenidis N, Kafousi M, et al. Molecular detection and prognostic value of circulating cytokeratin-19 messenger RNA-positive and HER2 messenger RNA-positive cells in the peripheral blood of women with early-stage breast cancer. Clin Breast Cancer. 2007; 7:883–889.


19. Ignatiadis M, Xenidis N, Perraki M, Apostolaki S, Politaki E, Kafousi M, et al. Different prognostic value of cytokeratin-19 mRNA positive circulating tumor cells according to estrogen receptor and HER2 status in early-stage breast cancer. J Clin Oncol. 2007; 25:5194–5202.


20. Ignatiadis M, Kallergi G, Ntoulia M, Perraki M, Apostolaki S, Kafousi M, et al. Prognostic value of the molecular detection of circulating tumor cells using a multimarker reverse transcription-PCR assay for cytokeratin 19, mammaglobin A, and HER2 in early breast cancer. Clin Cancer Res. 2008; 14:2593–2600.


21. Chen Y, Zou TN, Wu ZP, Zhou YC, Gu YL, Liu X, et al. Detection of cytokeratin 19, human mammaglobin, and carcinoembryonic antigen-positive circulating tumor cells by three-marker reverse transcription-PCR assay and its relation to clinical outcome in early breast cancer. Int J Biol Markers. 2010; 25:59–68.


22. Benoy IH, Elst H, Philips M, Wuyts H, Van Dam P, Scharpé S, et al. Real-time RT-PCR detection of disseminated tumour cells in bone marrow has superior prognostic significance in comparison with circulating tumour cells in patients with breast cancer. Br J Cancer. 2006; 94:672–680.


23. Daskalaki A, Agelaki S, Perraki M, Apostolaki S, Xenidis N, Stathopoulos E, et al. Detection of cytokeratin-19 mRNA-positive cells in the peripheral blood and bone marrow of patients with operable breast cancer. Br J Cancer. 2009; 101:589–597.


24. Grünewald K, Haun M, Urbanek M, Fiegl M, Müller-Holzner E, Gunsilius E, et al. Mammaglobin gene expression: a superior marker of breast cancer cells in peripheral blood in comparison to epidermal-growth-factor receptor and cytokeratin-19. Lab Invest. 2000; 80:1071–1077.


25. Cha YJ, Jung WH, Cho NH, Koo JS. Expression of sarcosine metabolism-related proteins in invasive lobular carcinoma: comparison to invasive ductal carcinoma. Yonsei Med J. 2015; 56:598–607.


26. Criscitiello C, Sotiriou C, Ignatiadis M. Circulating tumor cells and emerging blood biomarkers in breast cancer. Curr Opin Oncol. 2010; 22:552–558.


27. Thurm H, Ebel S, Kentenich C, Hemsen A, Riethdorf S, Coith C, et al. Rare expression of epithelial cell adhesion molecule on residual micrometastatic breast cancer cells after adjuvant chemotherapy. Clin Cancer Res. 2003; 9:2598–2604.
28. Rack B, Schindlbeck C, Jückstock J, Andergassen U, Hepp P, Zwingers T, et al. Circulating tumor cells predict survival in early average-to-high risk breast cancer patients. J Natl Cancer Inst. 2014; 05. 15. [Epub]. DOI: 10.1093/jnci/dju066.


29. Soltani S, Mokarian F, Panjehpour M. The expression of CK-19 gene in circulating tumor cells of blood samples of metastatic breast cancer women. Res Pharm Sci. 2015; 10:485–496.
30. Hayes DF, Cristofanilli M, Budd GT, Ellis MJ, Stopeck A, Miller MC, et al. Circulating tumor cells at each follow-up time point during therapy of metastatic breast cancer patients predict progression-free and overall survival. Clin Cancer Res. 2006; 12(14 Pt 1):4218–4224.


31. de Bono JS, Scher HI, Montgomery RB, Parker C, Miller MC, Tissing H, et al. Circulating tumor cells predict survival benefit from treatment in metastatic castration-resistant prostate cancer. Clin Cancer Res. 2008; 14:6302–6309.


32. Tewes M, Aktas B, Welt A, Mueller S, Hauch S, Kimmig R, et al. Molecular profiling and predictive value of circulating tumor cells in patients with metastatic breast cancer: an option for monitoring response to breast cancer related therapies. Breast Cancer Res Treat. 2009; 115:581–590.


33. Hyun KA, Koo GB, Han H, Sohn J, Choi W, Kim SI, et al. Epithelial-to-mesenchymal transition leads to loss of EpCAM and different physical properties in circulating tumor cells from metastatic breast cancer. Oncotarget. 2016; 7:24677–24687.


34. Lohr JG, Adalsteinsson VA, Cibulskis K, Choudhury AD, Rosenberg M, Cruz-Gordillo P, et al. Whole-exome sequencing of circulating tumor cells provides a window into metastatic prostate cancer. Nat Biotechnol. 2014; 32:479–484.


35. Aceto N, Bardia A, Miyamoto DT, Donaldson MC, Wittner BS, Spencer JA, et al. Circulating tumor cell clusters are oligoclonal precursors of breast cancer metastasis. Cell. 2014; 158:1110–1122.

