Abstract
Purpose
We aimed to establish the propofol effect-site concentration (Ce) for appropriate sedation by pharmacodynamic analysis and to determine the propofol Ce during occurrence of sedation-related side effects in pediatric patients undergoing brain magnetic resonance imaging (MRI).
Materials and Methods
In 50 pediatric patients scheduled for brain MRI, sedation was induced with 2.0 mg/kg propofol; additional propofol doses were 0.5–1 mg/kg. Propofol Ce was simulated by inputting the propofol administration profiles of patients into a pediatric compartmental model (Choi model). The relationship between propofol Ce and probabilities of sedation and recovery were analyzed using a sigmoidal Emax model. The simulated propofol Ce for sedation-related side effects was investigated. Population model parameters were estimated using the Nonlinear Mixed-Effects Modelling software.
Results
The mean values of propofol Ce50 for sedation during the preparation, scanning, and recovery phases were 1.23, 0.43, and 0.39 µg/mL. The simulated propofol Ce values during oxygen desaturation (SpO2 <90%) (3 patients; 6%), hypotension (16 patients; 32%), and bradycardia (12 patients; 24%) were 3.01±0.04, 2.05±0.63, and 2.41±0.89 µg/mL, respectively.
Conclusion
The required propofol Ce50 for applying monitors during the preparation phase before the start of MRI was higher than the propofol Ce50 required during the scanning phase. During low-intensity stimulation phases, such as scanning, propofol bolus dose should be strictly titrated not to exceed the propofol Ce that can lead to oxygen desaturation because of the relatively low propofol Ce (Ce95, 1.43 µg/mL) required for sedation in most patients.
Acquisition of accurate magnetic resonance (MR) images in pediatric patients requires sedation because MR imaging (MRI) is very sensitive to motion artefacts.1 Administration of hypnotic agents prior to the start of MRI is mandatory, because most children are not reassured by verbal explanation. Additional administration of sedatives might also be required to prevent patient movement during MRI. Propofol is a popular intravenous anesthetic for pediatric sedation because of its properties of rapid onset and high efficacy of sedation, rapid and complete recovery, and prevention of nausea and vomiting.2345 However, at high concentrations, propofol is associated with a high incidence of side effects, including hypotension, bradycardia, respiratory depression, and apnea.67 Therefore, anesthesiologists should administer appropriate dosages of propofol to ach-ieve adequate sedation without any adverse effects. However, because of the lack of MRI-compatible assessment monitors for the level of sedation (e.g., bispectral index) and target-controlled infusion systems, it is challenging to determine the appropriate dosage and time interval of additional propofol administration. Furthermore, few studies to date have evaluated optimal propofol dosing strategies for achieving adequate sedation with minimal adverse effects.8910 The ability to predict the appropriate propofol effect-site concentration (Ce) for sedation, as well as its side effects, will be clinically advantageous, because the Ce of a drug is considered a surrogate for estimating its effects without time delay. Simulation and population pharmacodynamic analysis can help determine the probability of sedation and adverse effects of sedation at specific propofol Ce values simulated on the basis of the pharmacokinetic parameters of the compartmental model.
This study aimed to establish the propofol Ce for appropriate sedation by pharmacodynamic analysis and to investigate the Ce of propofol during occurrence of sedation-related side effects in pediatric patients undergoing brain MRI.
This retrospective study was approved by the Institutional Review Board of Gangnam Severance Hospital (#3-2013-0075). The medical data of fifty patients between the ages of 4 and 12 years scheduled for elective brain MRI (with and without contrast) under sedation were reviewed retrospectively. Patients with heart and lung diseases, airway abnormalities, uncontrolled movement, and recent history of use of anticonvulsants or psychotropic medication were excluded. The pediatric sedation protocol for MRI scanning was used in our hospital as follows: Preprocedural fasting of 6 h for solid food and 2 h for clear fluids were ensured for MRI. Baseline values of mean arterial pressure (MAP) and heart rate (HR) were recorded, and an intravenous cannula was placed in the general ward before the patients were brought into the preparation room for MRI. Sedation was induced with a combination of 2 mg/kg propofol and 2 mg/mL lidocaine administered over 30 s before application of monitors for recording electrocardiography (ECG), non-invasive blood pressure (NIBP), oxygen saturation (SpO2), and end-tidal CO2 level. Supplemental oxygen was supplied at 3–4 L/min, and nasal capnography was performed. Patients were provided earplugs for protection against the operating noise of the scanner and were appropriately positioned on the scanning table using a soft neck roll. During the preparatory period for application of monitors, patients exhibiting University of Michigan Sedation Scale (UMSS) (Table 1) scores ≥3 and no movement after 1.5 min of propofol administration were considered adequately “sedated” for the preparation phase, while those exhibiting movement or UMSS scores <3 were considered to be “not sedated,” after which 0.5–1 mg/kg propofol was additionally administered. Following the preparation phase, when patients were judged to be adequately sedated, scanning was started after ensuring airway patency and adequacy of spontaneous respiration. During the MR scanning period, if patients were considered to be “not sedated,” 0.5–1 mg/kg propofol was additionally administered. In case of suspected airway obstruction or oxygen desaturation, scanning was stopped, the patient was brought out from the imaging tunnel, and the neck was extended with a chin lift. Upon completion of the scanning procedure, patients were transported to the adjacent recovery room, where HR, MAP, respiratory rate, and SpO2 were monitored by a nurse. “Recovery” from sedation was defined by spontaneous eye opening and vocalization by the patients. Parents were allowed to stay with the patients in the recovery room. Upon fulfilment of the discharge criteria,11 patients were discharged from the recovery room.12
The dosage and time of administration of propofol, appropriateness of sedation, and incidence of hypotension (MAP 20% below the baseline value), bradycardia (HR 20% below the baseline value), oxygen desaturation (SpO2 <90%), and the managements for sedation-related events were collected from electronic medical records. On the assumption that stimuli presented to the patients would vary during preparation, scanning, and recovery, those periods were divided into three phases: 1) preparation phase, period starting from application of monitors for recording ECG, NIBP, and SpO2 before the start of MRI; 2) scanning phase, period of MR image acquisition; and 3) recovery phase, period from the end of MRI to recovery of the patient. Recovery time was defined as the duration of the recovery phase, while scanning time was defined as the period from the start of MR image acquisition to its completion.
Propofol Ce was simulated by inputting the propofol administration profile of each patient into the pediatric compartmental model proposed by Choi, et al.,13 using a simulation program (http://www.pkpdtools.com). The pharmacodynamic model for assessment of adequate sedation and recovery was developed using the population approach. The relationships between propofol Ce and the probabilities of sedation for the preparation and scanning phases (not sedated=0; sedated=1, equation 1) and recovery for the recovery phase (sedated=0; recovered=1, equation 2) were analyzed using the following sigmoidal Emax models: where P is the probability of the patients being “sedated”; Ce50 is the value of Ce associated with a 50% probability of the patients being “sedated”; and λ is the steepness of the concentration-versus-response curve.
where P is the probability of the patients having “recovered”; Ce50 is the value of Ce associated with a 50% probability of the patients having “recovered”; and λ is the steepness of the concentration-versus-response curve. The likelihood of the observed response, R (sedation in equation 1 and recovery in equation 2), was described by the following equation:
Model parameters were estimated using the option “Likelihood Laplace Method=conditional” in the Nonlinear Mixed-Effects Modelling (NONMEM) software (version VII; Globo-Max, Hanover, MD, USA). Random inter-individual variabilities of Ce50 and λ were modelled using a log-normal model or, if necessary, fixed to zero. Propofol Ce associated with a 95% probability of sedation or recovery (Ce95) was estimated from the parameters of our pharmacodynamics model for the relationship between propofol Ce and the probabilities of sedation and recovery. For each analysis, NONMEM computes the minimum value of the objective function, a statistic proportional to twice the negative log likelihood of the data. A nonparametric bootstrap procedure was performed for internal validation using the Perl-speaks-NONMEM (PsN) software (version 3.6.2; https://uupharmacometrics.github.io/PsN), and the original data set was randomly sampled to generate 2000 bootstrap replicates. The 95% confidence intervals (CIs) of the nonparametric bootstrap replicates were determined and compared with the final values of the model parameters. Descriptive statistical analyses were performed with SPSS (IBM SPSS Statistics, version 23; IBM Corp., Armonk, NY, USA).
All 50 of the enrolled patients completed the study without dropping out. The most common indications for MRI included developmental delay, myopathy, and headache. The demographic and clinical characteristics of the enrolled patients are presented in Table 2. While 13 patients (26%) underwent MRI with a single dose of propofol, 37 patients (74%) required additional administration after the first bolus. Table 3 lists the values of the parameters and bootstrap estimates of the pharmacodynamic models for sedation and recovery. As indicated by the narrow 95% CIs and <50% relative standard errors, all parameters were estimated with adequate precision, and the probabilities of sedation and recovery were adequately described with a sigmoidal Emax model using the propofol Ce. There was no covariate, such as sex, age, body weight or developmental delay, included to improve the performance of the model for each phase based on the likelihood ratio test. The simulated Ce95 values for sedation during preparation and scanning phase and recovery during recovery phase were 2.77, 1.43, and 0.31 µg/mL, respectively (Fig. 1).
Three patients (6%) experienced oxygen desaturation (SpO2, 74–90%) during the scanning phase, which was resolved immediately by administering jaw thrust and chin lift maneuvers. The estimated Ce at which oxygen desaturation occurred in these patients was 2.91–3.09 µg/mL. Hypotension and bradycardia were transiently observed in 16 (32%) and 12 (24%) patients and were resolved without special treatment. The estimated Ce values at which hypotension and bradycardia occurred were 2.05±0.63 and 2.41±0.89 µg/mL, respectively. Fig. 2 and Supplementary Table 1 (only online) present an example of the simulated dosing strategy for achieving Ce95 values of 2.77 µg/mL (Ce95 required for sedation during the preparation phase) and 1.43 µg/mL (Ce95 required for sedation during the scanning phase) in a patient (body weight: 10, 15, 20, 25, and 30 kg) on the basis of the parameters of our pharmacodynamic model.
In the present study, we simulated the propofol Ce using the propofol administration profile of each patient and established the pharmacodynamic relationships between propofol Ce and the probabilities of sedation and recovery for MRI in pediatric patients. We used a non-linear sigmoidal Emax model for analysis14 and estimated not only the Ce50 value but also the steepness of the concentration-effect relationship (γ). We also simulated the propofol Ce values at which sedation-related side effects were observed.
Propofol is considered to be the best intravenous drug for pediatric sedation.341516 It offers several advantages over pentobarbital, midazolam, and fentanyl,78 including faster induction, shorter emergence, shorter duration of stay in the postanesthesia care unit (PACU), and fewer interruptions during MRI.1718 However, its narrow therapeutic window and the vulnerability of patients to its sedative effects might quickly lead to unintended deep anesthesia with loss of protective reflexes upon even small increases in dosage.1920 An appropriate low dosage of propofol that ensures an adequate depth of sleep for the successful completion MRI would probably help minimize these adverse events. When the Ce of propofol for appropriate sedation is unknown, there is no alternative for the dosing strategy, other than depending entirely on the experience of the clinician. This means that, with the aid of estimated Ce values of a drug for response, it is possible to predict the responsiveness of patients and the side effects of the drug in advance by simulating Ce values with the drug administration profiles, regardless of whether the drug is administered through bolus or continuous infusion.
In the present study, anesthesia was induced with 2.0 mg/kg propofol, which has been considered as the conventional dosage in previous studies.1521 There were no instances of airway compromise or respiratory depression during the preparation phase, although nine patients (18%) required additional administration of 1.0 mg/kg propofol because of inadequate sedation. In a previous study, an induction dose of 2.69 mg/kg propofol was found to be adequate for MRI in patients with cerebral palsy; however, 25% of the patients in that study experienced oxygen desaturation with partial airway obstruction immediately after the bolus dose.22 According to our findings, the simulated mean Ce95 of propofol for sedation during the preparation phase prior to the start of MRI was 2.77 µg/mL. Considering these results, an initial dosage of 2.4–2.5 mg/kg propofol would be appropriate for achieving a Ce of approximately 2.77 µg/mL, which could induce sedation with 95% probability while minimizing the risk of oxygen desaturation. During the scanning phase, patients require deep sedation for the successful completion of MRI without any undesirable patient movement, discomfort, pain, or anxiety.2324 Therefore, in the present study, the state of adequate sedation was defined when patients exhibited UMSS scores ≥3, indicating deep sedation. However, the concentrations of propofol required for scanning phase (Ce50, 0.43 µg/mL; Ce95, 1.43 µg/mL) were lower than those required for the preparation phase, and those were lower than we had expected. We had the patients wear earplugs for protection against the operating noise of the MR scanner while applying other monitors. Although it has not been proven whether earplugs shut out noise effectively, they might help reduce the required propofol concentration during MRI.
It might be presumed that the propofol concentration required for recovery would be much lower than that required for sedation during the scanning phase. However, according to our results, the Ce50 for recovery (0.39 µg/mL) was a little lower than that for sedation during scanning (0.43 µg/mL). This might be attributable to the stimuli encountered after the scanning procedure: stimuli provided by the removal of earplugs and detachment of monitors after the completion of MRI would be more intense than initially expected. The value of λ, which represents the steepness of the dose-response curve, in the recovery phase was greater than that in the scanning phase. This finding suggests abrupt recovery of patients during the recovery phase, which might have also been caused by the aforementioned physical stimuli encountered at the end of scanning.
Hypotension, bradycardia, and arterial oxygen desaturation occur more commonly with propofol than with other sedatives.7 Oxygen desaturation, one of the most disastrous side effects of sedation with propofol, was observed in three patients at 2–5 min after additional administration of propofol during the scanning phase. Given that these patients readily recovered upon administration of the jaw thrust and chin lift maneuvers, the oxygen desaturation was probably caused by airway obstruction rather than hypoventilation. Propofol induces hypoxemia more readily in pediatric patients than in adults because of their narrower airways and lower functional residual capacity.25 In the present study, the simulated propofol Ce that caused oxygen desaturation, which estimated from pharmacokinetic parameters and the blood-brain equilibration rate constant (ke0) of the Choi model13 was 2.9–3.1 µg/mL. The dosage (0.5–1 mg/kg) mentioned in our study should be titrated strictly, especially for additional administration of propofol during low-intensity stimulation phases, such as scanning, in order to ensure a low probability of oxygen desaturation. Additionally, airway patency should be monitored more vigorously immediately after the peak time of propofol Ce after additional administration. Otherwise, continuous infusion of low dose propofol based on simulation with our pharmacodynamic model could be more advantageous than intermittent bolus administration of propofol as shown in Supplementary Table 1 (only online), because bolus administration of propofol might often be unnecessarily higher than the dosage required for sedation in patients during scanning phase. In the present study, hypotension and bradycardia were observed at propofol Ce values of 2.05±0.63 and 2.41±0.89 µg/mL in 32% and 24% of the patients, respectively, a few minutes after additional administration of propofol. Hypotension and bradycardia occurred at lower values of propofol Ce than did oxygen desaturation; however, these effects were transient and resolved spontaneously. In our experience, these changes were benign and not associated with any adverse events.
There are a few limitations to the present study. We did not evaluate the plasma propofol concentrations. We also presumed the simulated propofol Ce values derived from the Choi model to be applicable to the enrolled study population. Although a perfect pharmacokinetics model for pediatrics has yet to be established, the Choi model, which has been recently developed and externally validated in children, is considered reliable and appropriate for pharmacodynamic modelling. This unique model includes pharmacokinetic parameters as well as the ke0 of propofol obtained in a single population of children.132627 Another limitation of the present study is that our pharmacodynamic model was developed using data acquired without premedication or analgesics. Therefore, in order to avoid oversedation, propofol Ce should be reduced when other drugs with potentially synergistic effects on sedation are concurrently administered.2829 However, propofol alone is close to an ideal sedative for non-painful procedures, such as MRI or nuclear imaging. Moreover, the use of multidrug sedation regimens is not clinically recommended because of their increased risk of adverse respiratory events.30
In conclusion, this clinical investigation is the first to report a pharmacodynamic model that can help establish the guidelines for optimal propofol sedation with minimal risk of oxygen desaturation during MRI. The required propofol Ce50 for applying monitors during the preparation phase before the start of MRI is higher than the propofol Ce50 required during the scanning phase. During low-intensity stimulation phases, such as scanning, propofol bolus dose should be strictly titrated not to exceed the propofol Ce that lead to oxygen desaturation because of relatively low propofol Ce (Ce95, 1.43 µg/mL) required for sedation in most patients. Also, despite the low probability of oxygen desaturation, airway patency should be monitored vigorously immediately after additional administration of propofol during the scanning phase. Our pharmacodynamic model representing the relationships between propofol Ce and the probabilities of sedation and recovery could help determine the dosages and infusion rates of propofol for all methods of delivery.
Figures and Tables
Fig. 1
Probability curves for propofol Ce versus sedation (preparation and scanning phase) and recovery (recovery phase). The simulated Ce95 values for sedation in preparation and scanning phase and recovery in recovery phase are 2.77, 1.43, and 0.31 µg/mL, respectively. Preparation phase, period starting from application of monitors for recoding electrocardiography readings, non-invasive blood pressure, and arterial oxygen saturation prior to the start of MRI; scanning phase, period of MR image acquisition; recovery phase, period from the end of MRI to the recovery of the patient. Ce, effect-site concentration; MRI, magnetic resonance imaging.
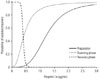
Fig. 2
Propofol Ce required for 95% probability of sedation during the preparation (2.77 µg/mL) and scanning phase (1.43 µg/mL), respectively. Blue line represents target propofol Ce for sedation and red line represents actual propofol Ce achieving target propofol Ce. A: preparation phase, period starting from application of monitors for recording electrocardiography readings, non-invasive blood pressure, and arterial oxygen saturation prior to the start of MRI. B: scanning phase, period of MR image acquisition. C: recovery phase, period from the end of MRI to the recovery of the patient. Ce, effect-site concentration; MRI, magnetic resonance imaging.
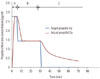
Table 1
The University of Michigan Sedation Scale
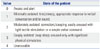
Table 2
Demographic and Clinical Characteristics of the 50 Pediatric Patients Scheduled for Elective Brain MRI under Sedation
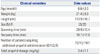
Table 3
Findings of the Population Pharmacodynamic Models for Sedation and Recovery with Propofol
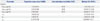
Ce50(m), propofol Ce associated with a 50% probability of being at phase “m”; λ(m), steepness of the concentration-versus-response curve at phase “m”; phase 1 (preparation), period starting from the application of monitors including electrocardiography, non-invasive blood pressure, and SpO2 prior to the start of MRI; phase 2 (scanning), period of MR image acquisition; phase 3 (recovery), period from the end of MRI to recovery of the patient. Ce, effect-site concentration; RSE, relative standard error; CV, coefficient of variation; MRI, magnetic resonance imaging.
ACKNOWLEDGEMENTS
This work was performed in the Department of Anesthesia and Pain Medicine, Gangnam Severance Hospital, Seoul, Korea.
References
1. Mark A, Brown RCS. Magnetic resonance imaging principles and applications. In : Joseph KTL, Stuart SS, Robert JS, Jay PH, editors. Computed body tomography with MRI correlation. 4th ed. Philadelphia: Lippincott Williams & Wilkins;2006. p. 29–93.
2. Hasan RA, Shayevitz JR, Patel V. Deep sedation with propofol for children undergoing ambulatory magnetic resonance imaging of the brain: experience from a pediatric intensive care unit. Pediatr Crit Care Med. 2003; 4:454–458.


3. Serafini G, Zadra N. Anaesthesia for MRI in the paediatric patient. Curr Opin Anaesthesiol. 2008; 21:499–503.


5. Vangerven M, Van Hemelrijck J, Wouters P, Vandermeersch E, Van Aken H. Light anaesthesia with propofol for paediatric MRI. Anaesthesia. 1992; 47:706–707.


6. Cravero JP, Beach ML, Blike GT, Gallagher SM, Hertzog JH. Pediatric Sedation Research Consortium. The incidence and nature of adverse events during pediatric sedation/anesthesia with propofol for procedures outside the operating room: a report from the Pediatric Sedation Research Consortium. Anesth Analg. 2009; 108:795–804.


7. Mallory MD, Baxter AL, Kost SI. Pediatric Sedation Research Consortium. Propofol vs pentobarbital for sedation of children undergoing magnetic resonance imaging: results from the Pediatric Sedation Research Consortium. Paediatr Anaesth. 2009; 19:601–611.


8. Pershad J, Wan J, Anghelescu DL. Comparison of propofol with pentobarbital/midazolam/fentanyl sedation for magnetic resonance imaging of the brain in children. Pediatrics. 2007; 120:e629–e636.


9. Machata AM, Willschke H, Kabon B, Kettner SC, Marhofer P. Propofol-based sedation regimen for infants and children undergoing ambulatory magnetic resonance imaging. Br J Anaesth. 2008; 101:239–243.


10. Kitt E, Friderici J, Kleppel R, Canarie M. Procedural sedation for MRI in children with ADHD. Paediatr Anaesth. 2015; 25:1026–1032.


11. American Society of Anesthesiologists Task Force on Sedation and Analgesia by Non-Anesthesiologists. Practice guidelines for sedation and analgesia by non-anesthesiologists. Anesthesiology. 2002; 96:1004–1017.
13. Choi BM, Lee HG, Byon HJ, Lee SH, Lee EK, Kim HS, et al. Population pharmacokinetic and pharmacodynamic model of propofol externally validated in children. J Pharmacokinet Pharmacodyn. 2015; 42:163–177.


14. Lu W, Ramsay JG, Bailey JM. Reliability of pharmacodynamic analysis by logistic regression: mixed-effects modeling. Anesthesiology. 2003; 99:1255–1262.
15. Srinivasan M, Turmelle M, Depalma LM, Mao J, Carlson DW. Procedural sedation for diagnostic imaging in children by pediatric hospitalists using propofol: analysis of the nature, frequency, and predictors of adverse events and interventions. J Pediatr. 2012; 160:801–806.e1.


16. Schulte-Uentrop L, Goepfert MS. Anaesthesia or sedation for MRI in children. Curr Opin Anaesthesiol. 2010; 23:513–517.


17. Koroglu A, Teksan H, Sagir O, Yucel A, Toprak HI, Ersoy OM. A comparison of the sedative, hemodynamic, and respiratory effects of dexmedetomidine and propofol in children undergoing magnetic resonance imaging. Anesth Analg. 2006; 103:63–67.


18. Gutmann A, Pessenbacher K, Gschanes A, Eggenreich U, Wargenau M, Toller W. Propofol anesthesia in spontaneously breathing children undergoing magnetic resonance imaging: comparison of two propofol emulsions. Paediatr Anaesth. 2006; 16:266–274.


19. Krauss B, Green SM. Sedation and analgesia for procedures in children. N Engl J Med. 2000; 342:938–945.


20. Koo BN, Shin S, Kim SY, Kang YR, Jeong KH, Han DW. Pharmacodynamic estimate of propofol-induced sedation and airway obstruction effects in obstructive sleep apnea-hypopnea syndrome. Yonsei Med J. 2015; 56:1408–1414.


21. Milius EM, Papademetrious TR, Heitlinger LA. Retrospective review of propofol dosing for procedural sedation in pediatric patients. J Pediatr Pharmacol Ther. 2012; 17:246–251.


22. Kim EJ, Jo YY, Kil HK. Optimal sedative dose of propofol to start MRI in children with cerebral palsy. Korean J Anesthesiol. 2011; 61:216–219.


23. Arthurs OJ, Sury M. Anaesthesia or sedation for paediatric MRI: advantages and disadvantages. Curr Opin Anaesthesiol. 2013; 26:489–494.
24. Osborn IP. Magnetic resonance imaging anesthesia: new challenges and techniques. Curr Opin Anaesthesiol. 2002; 15:443–448.


26. Rigouzzo A, Girault L, Louvet N, Servin F, De-Smet T, Piat V, et al. The relationship between bispectral index and propofol during target-controlled infusion anesthesia: a comparative study between children and young adults. Anesth Analg. 2008; 106:1109–1116.


27. Constant I, Rigouzzo A. Which model for propofol TCI in children. Paediatr Anaesth. 2010; 20:233–239.


28. McClune S, McKay AC, Wright PM, Patterson CC, Clarke RS. Synergistic interaction between midazolam and propofol. Br J Anaesth. 1992; 69:240–245.


SUPPLEMENTARY MATERIAL
Supplementary Table 1
An Example of the Simulated Dosing Strategy for Achieving Propofol Effect-Site Concentrations (Target Propofol Ce) of 2.77 and 1.43 µg/mL (Ce95 for Sedation) during the Preparation and MRI Scanning Phases, Respectively, in a Patient (Body Weight; 10, 15, 20, 25 and 30 kg), Assuming 0–5 min of Preparation for Applying Monitors, 5–30 min for MRI Scanning, and 30 min for Recovery