Abstract
Purpose
Our previous high-performance liquid chromatography-quadrupole time-of-flight mass spectrometry study identified bladder cancer (BCA)-specific urine metabolites, including carnitine, acylcarnitines, and melatonin. The objective of the current study was to determine which metabolic pathways are perturbed in BCA, based on our previously identified urinary metabolome.
Materials and Methods
A total of 135 primary BCA samples and 26 control tissue samples from healthy volunteers were analyzed. The association between specific urinary metabolites and their related encoding genes was analyzed.
Results
Significant alterations in the carnitine-acylcarnitine and tryptophan metabolic pathways were detected in urine specimens from BCA patients compared to those of healthy controls. The expression of eight genes involved in the carnitine-acylcarnitine metabolic pathway (CPT1A, CPT1B, CPT1C, CPT2, SLC25A20, and CRAT) or tryptophan metabolism (TPH1 and IDO1) was assessed by RT-PCR in our BCA cohort (n=135). CPT1B, CPT1C, SLC25A20, CRAT, TPH1, and IOD1 were significantly downregulated in tumor tissues compared to normal bladder tissues (p<0.05 all) of patients with non-muscle invasive BCA, whereas CPT1B, CPT1C, CRAT, and TPH1 were downregulated in those with muscle invasive BCA (p<0.05), with no changes in IDO1 expression.
Among men, bladder cancer (BCA) is the seventh most common cancer worldwide and the fourth most common in developed countries.1 Although cystoscopy and cytology are the standard diagnostic tools for BCA, cystoscopy has low sensitivity for high-grade superficial tumors [i.e., carcinoma in situ (CIS)] and cytology has poor sensitivity for low-grade tumors.23 Therefore, there is a growing interest in the development of new, noninvasive, and accurate methods for the detection of BCA.
Metabolic dysfunction has been implicated in a wide variety of human diseases including BCA.4 Recent advances on technological and data analysis have enabled the characterization of metabolites in biofluids. Metabolomics has been applied increasingly in the field of toxicology and biomarker research,56 in particular for cancer diagnosis.78 Growth and division of tumor cells are associated with an increase in the activity of a variety of metabolic pathways. Genes linked to altered cancer metabolism contribute to production and secretion of the cancer-specific metabolites into biofluids.9 Recent studies reported that metabolites uniquely detected in urine samples from patients with BCA, not those in the matched non-cancer controls, could be a useful biomarker for BCA diagnosis and prognosis.4101112 Because the bladder serves as a temporary urine reservoir, analysis of urine metabolites could provide potential candidates of sensitive and specific biomarkers of BCA.4101112
In our previous study, we used a comprehensive mass spectrometry-based metabolomics approach to identify potential diagnostic biomarkers of BCA, employing 138 patients with BCA and 121 control subjects.13 This previous analysis revealed twelve metabolites as potential biomarkers for the detection of BCA. They were carnitine, ovalerylcarnitine, glutarylcarnitine, octenoylcarnitine, decanoylcarnitine, succinate, pyruvate, oxoglutarate, phosphoenolpyruvate, trimethyllysine, melatonin, and acetyl-CoA. Carnitine has two key functions: 1) the transport of long-chain fatty acids (FAs) into the mitochondria, where they are oxidized to produce energy, and 2) the removal of toxic compounds to prevent their accumulation.14 The carnitine-acylcarnitine metabolic pathway involves several enzymes, including carnitine palmitoyltransferase type 1 (CPT1) and carnitine acetyltransferase (CRAT).15 CPT1, which has several isoforms including CPT1A, CPT1B, and CPT1C, is a mitochondrial enzyme that plays a role in FA import into mitochondria for β-oxidation. Loss of CPT1 leads to the impaired FA oxidation and contributes to the cancer-associated cachexia, which is characterized by progressive weight loss resulting from a reduction in adipose tissue and skeletal muscle. CRAT catalyzes the reversible conversion of acetyl-CoA into acetyl-carnitine.15
Our earlier urinary metabolomics analysis showed the decreased urinary levels of melatonin in BCA patients compared to controls.13 Melatonin, a derivative of tryptophan, enters to cells via glucose transporters and has a broad range of biological functions, including regulation of circadian rhythms and detoxification of free radicals.16 Because of its antioxidant properties, melatonin is considered as a potential agent for the prevention and/or treatment of cancer.16
Due to the limited metabolome databases, there have been few studies analyzing the metabolomics databases in the context of the related genes/proteins and biological pathways/networks. The potential association between specific metabolites and their related tissue gene/protein expression in BCA remains unclear. An association between BCA-related metabolites and altered biochemical processes fundamental to BCA development and progression was proposed in a previous study, however, the findings were based on the limited experimental data of phase I/II metabolic enzymes (CYP1A1 and CYP1B1).4 In the present study, we assessed the relationship between the BCA-associated urinary metabolites and gene expression associated with relevant biological pathways using bladder tissues derived from BCA patients and controls.
The study protocol was approved by the Ethics Committee of Chungbuk National University, and written informed consent was obtained from each subject. The Institutional Review Board (IRB) of Chungbuk National University approved the protocols for the collection and analysis of samples (IRB approval number GR 2010-12-010).
A total of 135 primary BCA samples and 26 control tissue samples from healthy volunteers were analyzed. 120 of 135 BCA samples were overlapped between our previous studies13 and the current one. The study included primary tumor samples from patients with histologically-verified transitional cell carcinoma treated at our institute. All tumors were macrodissected, typically within 15 minutes of surgical resection. Each BCA specimen was verified by pathological analysis of tissue samples from fresh frozen sections of transurethral resection or radical cystectomy specimens. Specimens were frozen in liquid nitrogen and stored at -80℃ until use. Tumors were staged according to the 2002 TNM classification,17 while they were graded according to the 1973 World Health Organization grading system.18
In this study, two pathways, the carnitine-acylcarnitine metabolic pathway and tryptophan metabolism pathway, were selected for further analysis. The metabolic pathways associated with the selected urinary metabolites were identified using KEGG pathways (http://www.genome.jp/kegg). The association between specific urinary metabolites and their related encoding genes was analyzed (Table 2). Differences between two groups were analyzed using the Mann-Whitney U test. All statistical analyses were performed using SPSS® 21.0 software (SPSS Inc., Chicago, IL, USA), with p<0.05 considered statistically significant.
The mean age of the 135 BCA patients was 65.9±12.62 years. Eighty-two (60.7%) patients had non-muscle invasive bladder cancer (NMIBC) and 53 (39.3%) had muscle invasive bladder cancer (MIBC). The baseline characteristics of the patients are shown in Table 3.
Our previous urinary metabolomics-based analysis13 showed that patients with BCA had the elevated levels of urinary carnitine and several acylcarnitines, such as isovalerylcarnitine and octenoylcarnitine, and the decreased levels of urinary melatonin, glutarylcarnitine, and decanolycarnitine, compared to the control group. Bioinformatics analysis suggested that the levels of these metabolites may be altered mainly in two metabolic pathways, the carnitine-acylcarnitine metabolic pathway and the tryptophan metabolism. In order to quantify the expression levels of key genes in these two pathways, we performed qRT-PCR analysis using samples from 135 BCA patients (82 NMIBC and 53 MIBC) and 26 controls. Several key enzymes of the carnitine-acylcarnitine metabolic pathway or tryptophan pathway were selected for further validation (Table 2).
CPT catalyzes the transfer of the acyl group of a FA to carnitine to allow its movement from the cytosol to mitochondria for oxidation.20 Carnitine-acylcarnitine translocase (CACT, gene symbol: SLC25A29) plays a role in transporting both carnitine-FA complexes and carnitine across the inner mitochondrial membrane for beta-oxidation.21 CRAT catalyzes the reaction of acetyl-CoA plus carnitine to CoA plus acetylcarnitine.22 Tryptophan hydroxylase (TPH) is involved in the synthesis of the neurotransmitters serotonin and melatonin in tryptophan metabolism.23 Indoleamine 2,3-dioxygenase (IDO) is involved in the degradation of tryptophan in the tryptophan metabolic pathway.24 In the carnitine-acylcarnitine metabolic pathway, the mRNA expression levels of CPT1B and CPT1C, SLC25A20, and CRAT, but not CPT1A or CPT2, were significantly lower in NIMBC patients than in controls (p<0.05 each) (Table 4, Fig. 1). In the tryptophan metabolic pathway, the mRNA expression of TPH1 and IDO1 was significantly lower in NIMBC patients than in controls (p<0.05).
Among the carnitine-acylcarnitine metabolic pathway-associated genes, the expression levels of CPT1B, CPT1C, and CRAT were significantly lower in MIBC tissues than those of controls (p<0.05) (Table 4, Fig. 1). No significant differences in the expression of CPT2 and SLC25A20 were observed between MIBC patients and controls. CPT1A expression was significantly higher in MIBC patients than in controls (p=0.008). Among tryptophan metabolism-associated genes, only TPH1, but not IDO1, was significantly downregulated in MIBC compared to controls (p=0.001).
Octenoylcarnitine, urinary metabolites, was positively correlated with CPT1A and SLC25A20 (r=0.250, p=0.006 and r=0.255, p=0.005, respectively), while decanolylcarnitine was negatively correlated with CPT2 (r=-0.226, p=0.013). Other candidate urinary metabolites did not show any significant correlation with tissue RNA expression in BCA patients (Table 5).
Our previous study identified twelve urinary metabolites, including carnitine, and their derivatives and melatonin, as potential diagnostic biomarkers for BCA.13 The following bioinformatics analysis showed that these metabolites were enriched in the carnitine-acylcarnitine or tryptophan metabolic pathways. Given that metabolic pathways and associated genes/proteins/metabolites were altered in BCA, we next tested whether the expression levels of key genes involved in the carnitine-acylcarnitine metabolic pathway and tryptophan metabolism are also perturbed in bladder tissues obtained from patients with BCA.
A few studies have analyzed carnitine or carnitine metabolism-associated genes in BCA. Huang, et al.11 performed a liquid chromatography (LC) mass spectrometry-based metabolomics analysis and showed that the level of urinary carnitine C9:1 was significantly decreased in patients with BCA, particularly in those with early BCA. However, the contribution and regulatory mechanism of the carnitine metabolic pathway to BCA remains unclear.
Increased urinary levels of tryptophan and its metabolites have been reported in BCA.1012 Alberice, et al.10 showed that the levels of tryptophan and its related metabolite N-acetyltryptophan were significantly increased in the urine of patients with early BCA, and Pasikanti, et al.12 showed elevated concentrations of tryptophan metabolites in the urine of BCA patients. Despite accumulating evidence supporting the importance of tryptophan and its urinary metabolites in bladder carcinogenesis,25 the role of THP1, a key enzyme in tryptophan metabolism that catalyzes the conversion of tryptophan to serotonin and the depletion of tryptophan, in BCA has not been investigated to date. Our present data indicate that TPH1 levels are significantly decreased in patients with NMIBC and MIBC compared to controls. A tryptophan metabolite, melatonin, was also decreased in the urine of patients with BCA.
Metabolic profiling included high resolution spectroscopy, mainly nuclear magnetic resonance, and mass spectro-metry with LC or gas-chromatography. Because the final products of the metabolism of DNA, RNA, and protein are metabolites, the levels of metabolites may be a more accurate reflection of changes in metabolic activity than the levels of DNA, RNA, and protein. Therefore, metabolites could be important diagnostic markers because their levels may vary without significant changes in DNA, RNA, and protein levels, and metabolomics studies of BCA have been performed using urine, serum, and cancer tissues.41011122627 Urinary biomarkers for the detection of BCA are attractive because of their direct contact to cancer and the noninvasive nature of urine collection. Consequently, there has been growing interest in urinary metabolomics for BCA detection. However, validating urinary metabolomics profiles in independent cohorts or using different methods, and determining their association with biological properties and specific disorders have been challenges that need to be resolved.
In this study, we proposed the possibility of the association between urinary metabolites and tissue mRNA expression in BCA in Fig. 2. In the β-oxidation, carnitine and their carnitine derivatives are excreted in the urine from BCA tissue, because CPT1, CPT2, CACT mRNA expression associated with acyl-carnitine and carnitine metabolism were decreased. p53 is well known for its tumor suppressive functions and found to mutate in more than 50% MIBC.28 Mutant p53 resulted in TGF-beta driven invasion and metastasis in cancer cells.29 In this regard, Shariat, et al.30 reported that TGF-beta level in patients with MIBC was significantly higher than that in patients with NMIBC and normal controls. Interestingly, inhibition of p53 increased carnitine palmitoyltransferase 1 activity and p53 regulates mitochondrial respiration.3132 Therefore, we suggest that the gene expression involved in carnitine-acylcarnitine pathway in NMIBC might be downregulated compared to MIBC. Similarly, CPT1A gene expression might be upregulated in MIBC compared to NMIBC. Additionally, the decreased TPH1 might lead to the inhibition of metabolizing the tryptophan pathway to target metabolite, melatonin, although the decreased TPH1 level might be associated with the reduced metabolite such as melatonin in tryptophan metabolism. A metabolite in the tryptophan metabolic pathway, acetyl CoA, is the last metabolite in the FA metabolism, the glycolysis metabolism and the tryptophan metabolism. In our present study, we found the increase of acetyl-CoA level, which might be caused by the activated FA and glycolysis metabolism. IDO1 encode the enzyme IDO in human. This enzyme is produced by macrophage and other immunoregulatory cells.33 Elevation of IDO1 levels in bladder tumor cells might be associated with immune escape of tumor cells. In particular, in early tumorigenesis periods like NMIBC, the human immune systems may downregulate IDO1 and lead to the protection of cancer cells from immune response.
The present data generated by the use of metabolomics technologies may broaden our understanding of BCA. However, further data interpretation requires a rich integrated database of metabolome, transcriptome, and proteome, as well as systematic bioinformatics approach to confirm the biological function of metabolite candidates.
In conclusion, we showed that the expressions of genes involved in the carnitine-acylcarnitine and tryptophan pathways are associated with alterations in the levels of urinary metabolites in BCA patients. The present results may advance our understanding of the association between urinary metabolites and their related tissue gene expression in BCA.
Figures and Tables
![]() | Fig. 1Jitter plots showing mRNA levels in normal controls and patients with NMIBC or MIBC. RT-PCR analysis of the mRNA expression of CPT1A, CPT1B, CPT1C, CPT2, SLC25A20, CRAT, TPH1, and IDO1 was performed as described in the Materials and Methods section. p values were calculated using the Mann-Whitney U test. NMIBC, non-muscle invasive bladder cancer; MIBC, muscle invasive bladder cancer; IDO, indoleamine 2,3-dioxygenase; CPT, carnitine palmitoyltransferase; CRAT, carnitine O-acetyltransferase; TPH, tryptophan hydroxylase; SLC25A20, solute carrier family 25 [carnitine/acylcarnitine translocase (CACT)], member 20. |
![]() | Fig. 2Schematic pathway of bladder cancer-associated metabolic signatures in bladder cancer patients. CPT, carnitine palmitoyltransferase; CACT, carnitine/acylcarnitine translocase; CRAT, carnitine O-acetyltransferase; TPH, tryptophan hydroxylase; IDO, indoleamine 2,3-dioxygenase. |
Table 1
Sequences of the Primers Used in This Study
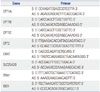
Table 2
Selected Urinary Metabolites in Bladder Cancer Patients and Their Related Pathways, Enzymes, and Encoding Genes

Table 3
Baseline Characteristics of Primary Bladder Cancer Patients
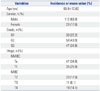
Table 4
Comparison of Tissue mRNA Expression Levels in Control vs. NMIBC or MIBC
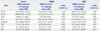
Table 5
Correlations between Urinary Metabolites and Tissue mRNA Expression in 120 Bladder Cancer Patients
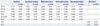
ACKNOWLEDGEMENTS
The authors thank Mr. T. Dan Park of the University of California, Berkerly for editing assistance. This study was supported by the National Research Foundation of Korea (NRF) grant funded by the Korea government (MSIP) (no. NRF-2014R1A2A1A09006983 and no. 2014R1A2A2A04007036) (to W.K.), and by NIH grants R01DK100974 (to J.K.). The biospecimens for this study were provided by the Chungbuk National University Hospital, a member of the National Biobank of Korea, which is supported by the Ministry of Health, Welfare, and Family Affairs. All samples provided by the National Biobank of Korea for this study were obtained with informed consent under institutional review board-approved protocols. The funders had no role in study design, data collection and analysis, decision to publish, or preparation of the manuscript.
References
1. Burger M, Catto JW, Dalbagni G, Grossman HB, Herr H, Karakiewicz P, et al. Epidemiology and risk factors of urothelial bladder cancer. Eur Urol. 2013; 63:234–241.


2. van der Aa MN, Steyerberg EW, Sen EF, Zwarthoff EC, Kirkels WJ, van der Kwast TH, et al. Patients' perceived burden of cystoscopic and urinary surveillance of bladder cancer: a randomized comparison. BJU Int. 2008; 101:1106–1110.


3. Lotan Y, Roehrborn CG. Sensitivity and specificity of commonly available bladder tumor markers versus cytology: results of a comprehensive literature review and meta-analyses. Urology. 2003; 61:109–118.


4. Putluri N, Shojaie A, Vasu VT, Vareed SK, Nalluri S, Putluri V, et al. Metabolomic profiling reveals potential markers and bioprocesses altered in bladder cancer progression. Cancer Res. 2011; 71:7376–7386.


5. Liu X, Zhang L, You L, Yu J, Zhao J, Li L, et al. Differential toxicological effects induced by mercury in gills from three pedigrees of Manila clam Ruditapes philippinarum by NMR-based metabolomics. Ecotoxicology. 2011; 20:177–186.


6. Xu XJ, Zheng P, Ren GP, Liu ML, Mu J, Guo J, et al. 2,4-Dihydroxypyrimidine is a potential urinary metabolite biomarker for diagnosing bipolar disorder. Mol Biosyst. 2014; 10:813–819.


7. Garcia E, Andrews C, Hua J, Kim HL, Sukumaran DK, Szyperski T, et al. Diagnosis of early stage ovarian cancer by 1H NMR metabonomics of serum explored by use of a microflow NMR probe. J Proteome Res. 2011; 10:1765–1771.


8. OuYang D, Xu J, Huang H, Chen Z. Metabolomic profiling of serum from human pancreatic cancer patients using 1H NMR spectroscopy and principal component analysis. Appl Biochem Biotechnol. 2011; 165:148–154.


9. Mitra AP, Bartsch CC, Cote RJ. Strategies for molecular expression profiling in bladder cancer. Cancer Metastasis Rev. 2009; 28:317–326.


10. Alberice JV, Amaral AF, Armitage EG, Lorente JA, Algaba F, Carrilho E, et al. Searching for urine biomarkers of bladder cancer recurrence using a liquid chromatography-mass spectrometry and capillary electrophoresis-mass spectrometry metabolomics approach. J Chromatogr A. 2013; 1318:163–170.


11. Huang Z, Lin L, Gao Y, Chen Y, Yan X, Xing J, et al. Bladder cancer determination via two urinary metabolites: a biomarker pattern approach. Mol Cell Proteomics. 2011; 10:M111.007922.


12. Pasikanti KK, Esuvaranathan K, Hong Y, Ho PC, Mahendran R, Raman Nee Mani L, et al. Urinary metabotyping of bladder cancer using two-dimensional gas chromatography time-of-flight mass spectrometry. J Proteome Res. 2013; 12:3865–3873.


13. Jin X, Yun SJ, Jeong P, Kim IY, Kim WJ, Park S. Diagnosis of bladder cancer and prediction of survival by urinary metabolomics. Oncotarget. 2014; 5:1635–1645.


14. Rebouche CJ. Carnitine. In : Shils ME, Olson JA, Shike M, Ross AC, editors. Modern Nutrition in Health and Disease. 9th ed. New York: Lippincott Williams and Wilkins;1999. p. 505–512.
15. Jogl G, Tong L. Crystal structure of carnitine acetyltransferase and implications for the catalytic mechanism and fatty acid transport. Cell. 2003; 112:113–122.


16. Reiter RJ, Tan DX, Terron MP, Flores LJ, Czarnocki Z. Melatonin and its metabolites: new findings regarding their production and their radical scavenging actions. Acta Biochim Pol. 2007; 54:1–9.


17. Babjuk M, Oosterlinck W, Sylvester R, Kaasinen E, Böhle A, Palou-Redorta J. European Association of Urology (EAU). AU guidelines on non-muscle-invasive urothelial carcinoma of the bladder. Eur Urol. 2008; 54:303–314.
18. Greene FL. The American Joint Committee on Cancer: updating the strategies in cancer staging. Bull Am Coll Surg. 2002; 87:13–15.
19. Kim WT, Yun SJ, Park C, Kim IY, Moon SK, Kwon TG, et al. Identification of C16orf74 as a marker of progression in primary non-muscle invasive bladder cancer. PLoS One. 2010; 5:e15260.


20. van der Leij FR, Huijkman NC, Boomsma C, Kuipers JR, Bartelds B. Genomics of the human carnitine acyltransferase genes. Mol Genet Metab. 2000; 71:139–153.


21. Murthy MS, Pande SV. Mechanism of carnitine acylcarnitine translocase-catalyzed import of acylcarnitines into mitochondria. J Biol Chem. 1984; 259:9082–9089.


23. McKinney J, Teigen K, Frøystein NA, Salaün C, Knappskog PM, Haavik J, et al. Conformation of the substrate and pterin cofactor bound to human tryptophan hydroxylase. Important role of Phe313 in substrate specificity. Biochemistry. 2001; 40:15591–15601.


24. Najfeld V, Menninger J, Muhleman D, Comings DE, Gupta SL. Localization of indoleamine 2,3-dioxygenase gene (INDO) to chromosome 8p12-->p11 by fluorescent in situ hybridization. Cytogenet Cell Genet. 1993; 64:231–232.


25. Bryan GT. The role of urinary tryptophan metabolites in the etiology of bladder cancer. Am J Clin Nutr. 1971; 24:841–847.


26. Bansal N, Gupta A, Mitash N, Shakya PS, Mandhani A, Mahdi AA, et al. Low- and high-grade bladder cancer determination via human serum-based metabolomics approach. J Proteome Res. 2013; 12:5839–5850.


27. Tripathi P, Somashekar BS, Ponnusamy M, Gursky A, Dailey S, Kunju P, et al. HR-MAS NMR tissue metabolomic signatures cross-validated by mass spectrometry distinguish bladder cancer from benign disease. J Proteome Res. 2013; 12:3519–3528.


28. Wu XR. Biology of urothelial tumorigenesis: insights from genetically engineered mice. Cancer Metastasis Rev. 2009; 28:281–290.


29. Adorno M, Cordenonsi M, Montagner M, Dupont S, Wong C, Hann B, et al. A Mutant-p53/Smad complex opposes p63 to empower TGFbeta-induced metastasis. Cell. 2009; 137:87–98.


30. Shariat SF, Kim JH, Andrews B, Kattan MW, Wheeler TM, Kim IY, et al. Preoperative plasma levels of transforming growth factor beta(1) strongly predict clinical outcome in patients with bladder carcinoma. Cancer. 2001; 92:2985–2992.


31. Derdak Z, Villegas KA, Harb R, Wu AM, Sousa A, Wands JR. Inhibition of p53 attenuates steatosis and liver injury in a mouse model of non-alcoholic fatty liver disease. J Hepatol. 2013; 58:785–791.

