Abstract
Purpose
Oxidative stress during CO2 pneumoperitoneum is reported to be associated with decreased bioactivity of nitric oxide (NO). However, the changes in endothelial nitric oxide synthase (eNOS), inducible nitric oxide synthase (iNOS), and arginase during CO2 pneumoperitoneum have not been elucidated.
Materials and Methods
Thirty male Sprague-Dawley rats were randomized into three groups. After anesthesia induction, the abdominal cavities of the rats of groups intra-abdominal pressure (IAP)-10 and IAP-20 were insufflated with CO2 at pressures of 10 mm Hg and 20 mm Hg, respectively, for 2 hours. The rats of group IAP-0 were not insufflated. After deflation, plasma NO was measured, while protein expression levels and activity of eNOS, iNOS, arginase (Arg) I, and Arg II were analyzed with aorta and lung tissue samples.
Results
Plasma nitrite concentration and eNOS expression were significantly suppressed in groups IAP-10 and IAP-20 compared to IAP-0. While expression of iNOS and Arg I were comparable between the three groups, Arg II expression was significantly greater in group IAP-20 than in group IAP-0. Activity of eNOS was significantly lower in groups IAP-10 and IAP-20 than in group IAP-0, while iNOS activity was significantly greater in group IAP-20 than in groups IAP-0 and IAP-10. Arginase activity was significantly greater in group IAP-20 than in groups IAP-0 and IAP-10.
Conclusion
The activity of eNOS decreases during CO2 pneumoperitoneum, while iNOS activity is significantly increased, a change that contributes to increased oxidative stress and inflammation. Moreover, arginase expression and activity is increased during CO2 pneumoperitoneum, which seems to act inversely to the NO system.
Laparoscopic surgery requires intra-abdominal carbon dioxide (CO2) insufflation with pressures commonly maintained at 10-15 mm Hg. This state of CO2 pneumoperitoneum and ensuing reperfusion injury upon deflation is known to cause a decrease in splanchnic blood flow and possible organ ischemia due to oxidative stress.12345 Although well-tolerated by most patients for even an extended period of time, this form of ischemia-reperfusion injury and oxidative stress has been recognized as a potentially serious problem in the elderly and parti-cularly those with multiple comorbidities.1
In order to minimize such injury after laparoscopic surgery, many efforts have been made to uncover the underlying me-chanism of oxidative stress caused by pneumoperitoneum. Among the many factors that have been proposed to contribute to oxidative stress, the decreased bioactivity of nitric oxide (NO) has recently received much attention. NO is known to act as a vasodilator and to have antithrombotic, anti-inflammatory, anti-proliferative, and antioxidant properties, and it is produced by three isoforms of NO synthase (NOS): neuronal NOS (nNOS), inducible NOS (iNOS), and endothelial NOS (eNOS).6 Inclusion of an NO donor substance in the insufflation gas was found to be effective in reducing hepatic and renal ischemia,78 and pretreatment with nitroglycerine also ameliorated the adverse renal effects due to CO2 pneumoperitoneum.29 On the other hand, pretreatment with NG-nitro-L-arginine methyl ester (L-NAME), an inhibitor of NOS, was shown to aggravate renal ischemia.210
Another factor that may be involved in changes of NO synthesis during laparoscopic surgery is the enzyme arginase. Given that both NOS and arginase share L-arginine as a substrate, arginase is thought to inhibit NO synthesis by competing with NOS for L-arginine.1112 Understandably, the inhibition of arginase has been shown to stimulate NO synthesis, while overexpression of arginase suppresses NO generation in endothelial cells.11 Arginase exists as either arginase I (Arg I) or arginase II (Arg II), and both have been reported to be associated with endothelial dysfunction and decreased NO, likely by regulating substrate availability.131415 In this context, the decreased bioactivity of NO during pneumoperitoneum can be hypothesized to be related to the relative up-regulation of arginase.
Although the relationship between decreased NO and spla-nchnic ischemia during CO2 pneumoperitoneum has been studied and supported by several recent studies, the changes in specific NOS isoforms have not been elucidated. While eNOS is the predominant isoform in the vasculature and is responsible for most of the NO produced in this tissue,16 iNOS is up-regulated in macrophages and virtually any cell or tissue during various inflammatory diseases.6 Furthermore, the role of arginase and its reciprocal interactions with NOS during pneumoperitoneum is a new area of interest, and arginase may serve as a novel target for reducing oxidative stress due to laparoscopic surgery. This study was performed to investigate the expression and activity of the different isoforms of NOS and arginase as well as the changes in NO and degree of oxidative stress during CO2 pneumoperitoneum in rats. The concentration of proinflammatory cytokines interleukin (IL)-1β, IL-6, and tumor necrosis factor (TNF)-α in the lungs were analyzed to assess the degree of stress response and tissue trauma due to CO2 pneumoperitoneum, while local oxidative stress in the lung tissue was evaluated by examining histopathological changes.
All procedures of this study were approved by the Yonsei University Institutional Animal Care and Use Committee. Experiments were performed on 30 male Sprague-Dawley rats that were 3-4 months old and weighing 250-320 g. The rats were allowed to adjust to the controlled laboratory environment of 22-24℃ for at least 7 days with free access to standard laboratory chow and tap water prior to experiments. After the rats were anesthetized with 2.0% sevoflurane inhalation in the supine position, they were safely secured on a thermoregulated pad (37℃) and mechanically ventilated with a volume-driven small-animal ventilator (model 665A, Harvard Apparatus, Holliston, MA, USA) after tracheotomy. Tidal volume was set between 0.5 and 1 mL/100 g of body weight, and the respiratory rate was adjusted to 55-60 breaths/min to maintain end-tidal CO2 at 30-35 mm Hg. Anesthesia was maintained with 1.5-2.0% sevoflurane with 50% oxygen in air throughout the study. The abdominal cavities of the rats were then punctured with a Veress needle that was connected to an electronic laparoflator for CO2 insufflation, and the rats were randomized to three groups of 10 animals each. The intra-abdominal pressures (IAPs) of the rats in groups IAP-10 and IAP-20 were maintained at 10 mm Hg and 20 mm Hg, respectively, for 2 hours, while the rats of group IAP-0 were not insufflated with CO2. Blood samples were collected 1 hour after abdominal deflation. The rats were then euthanized by exsanguination and the aorta and lung tissue were harvested and dissected from connective tissue in Kreb's solution.
Nitrite (NO2-) is a stable metabolite of NO and the most suitable and practical method to assess NO synthesis in vivo. Blood was collected in tubes using citrate and ethylenediaminetetraacetic acid (EDTA) as an anticoagulant and was centrifuged for 15 minutes at 1000 g. To measure nitrite, plasma was incubated with 100 µL of Griess reagent [1% sulfanilamide in 0.1 mol/L HCl and 0.1% N-(1-naphthyl) ethylenediamine dihydrochloride, Sigma-Aldrich, Saint Louis, MO, USA] at room temperature for 10 minutes. Absorbance was then measured at 540 nm using a microplate reader. The nitrite content was calculated based on a standard curve constructed with NaNO2.
Malondialdehyde (MDA) is a stable product of lipid peroxidation, which is regarded as an index of oxygen free-radical production. Aorta tissue MDA was analyzed using an OxiSelect™ MDA Adduct enzyme-linked immunosorbent assay (ELISA) kit (Cell Biolabs, Inc., San Diego, CA, USA). Absorbance was read by a microplate reader using 450 nm as the primary wave length after adding stop solution to stop the enzyme reaction.
Aorta and lung tissue were homogenized in ice-cold Proprep (Intron Biotechnology, Seongnam, Korea). The expressions of eNOS, Arg I, and Arg II were analyzed with aorta tissue, and iNOS expression was analyzed with lung tissue. Tissue extracts were loaded on 8-10% sodium dodecyl sulfate (SDS)-polyacrylamide gel, and the proteins were transferred on polyvinyl-difluoride membranes (0.2 µm: Immun-Blot, Bio-Rad, Hercules, CA, USA). Membranes were blocked using 5% (wt/vol) nonfat milk in Tris-buffered saline with 0.1% Tween-20. The blots were incubated overnight at 4℃ with primary antibodies, eNOS (Santa Cruz Biotechnology, Santa Cruz, CA, USA), iNOS (Santa Cruz Biotechnology, Santa Cruz, CA, USA), Arg I (R&D Systems, Minneapolis, MN, USA), and Arg II (Santa Cruz Biotechnology, Santa Cruz, CA, USA) at 1:1000 dilution. After incubation, the membranes were incubated with anti-rabbit, anti-sheep, or anti-mouse secondary antibodies at 1:5000 dilution. Protein expression was normalized to β-actin (1:1000 dilution). The bands were detected using a chemiluminescence assay (ECL Plus, Amersham Biosciences, Sunnyvale, CA, USA).
The activities of eNOS and iNOS were assayed by rat ELISA kits (Wuhan EIAab Science Co., Ltd., Wuhan, China) with aorta and lung tissue, respectively. In brief, the supernatants of aorta and lung tissue were homogenized in ice-cold Proprep, and then incubated in a 96-well plate containing the co-factors and substrate L-arginine for 1 hour at 37℃. After the incubation period, the enzyme-substrate reaction was terminated by the addition of stop solution, and the color change was measured spectrophotometrically at wave lengths of 530 nm and 450 nm for eNOS and iNOS, respectively.
Activity of intracellular arginase was determined using aorta digest cell lysates with the QuantiChrom™ Arginase Assay Kit (BioAssay Systems, Hayward, CA, USA), which measured the conversion of arginine to urea by arginase. After total protein quantification, the sample was incubated with arginine buffer at 37℃ for 2 hours. Optical density was read using a 430-nm filter.
IL-1β, IL-6, and TNF-α concentrations in the rat lungs were measured by ELISA, using kits for the measurement of rat IL-1β (eBioscience, San Diego, CA, USA), IL-6 (Young In Frontier Co., Ltd., Seoul, Korea), and TNF-α (BD Biosciences, San Jose, CA, USA). In brief, supernatants of lung tissue were added to a 96-well plate coated with corresponding antibodies to each cytokine and incubated. Streptavidin-horseradish peroxidase conjugate was added as an antibody to a second epitope on each cytokine. Absorbance was read using a microplate reader with 450 nm as the primary wave length after adding the stop solution.
The lungs of the rats were removed by median sternotomy, fixed with 10% paraformaldehyde through trachea infusion, and embedded with paraffin. Hematoxylin and eosin (H&E) staining was conducted using 4-µm tissue slides. Lung injury was assessed according to the modified scoring system described by Kristof, et al.17 In brief, two pulmonologists randomly selected ten fields of lung sections from three lobes of lung tissue for each rat and used a microscope at 200× magnification to read and score the damaged levels in these sections according to the extent of interstitial cellular infiltration, alveolar protein exudation, and tissue hemorrhage. A separate grade from 0 to 3 was calculated for each field, depending on its presence or absence. The sum of each category from 10 different microscopic fields was recorded as the final damaged score for each rat. The total lung injury score for each rat was determined as the sum of three individual scores for alveolar cellularity, protein exudation, and tissue hemorrhage. If the interpretations of the two physicians differed significantly, the slides were checked by a pathologist.
Data are described as mean±SD or numbers of subjects. Nonparametric data such as proportion of muscular pulmonary arteries were analyzed using the chi-square test. An ANOVA with Tukey's multiple comparison was performed to compare parametric variables among the three groups. p values less than 0.05 were considered statistically significant.
Plasma nitrite levels were significantly lower in rats of groups IAP-10 and IAP-20 than in group IAP-0 (8.43±2.09 µmol/mL and 8.70±2.90 µmol/mL vs. 13.70±3.20 µmol/mL, respectively; p<0.01 for both). Plasma nitrite was highest in group IAP-0 among all groups, while there was no difference between groups IAP-10 and IAP-20 (Fig. 1A).
MDA levels were significantly higher in group IAP-20 than in groups IAP-0 and IAP-10 (1.41±0.17 pmol/mg vs. 1.11±0.09 pmol/mg and 1.21±0.08 pmol/mg, respectively; p<0.001 and p<0.01, respectively). However, there was no difference in MDA levels between groups IAP-0 and IAP-10 (Fig. 1B).
Representative results of Western blot analysis are shown in Fig. 2A. The expression of eNOS was significantly more suppressed in groups IAP-10 and IAP-20 than in group IAP-0 (1.30±0.10 and 0.90±0.27 vs. 1.83±0.21, respectively; p<0.05 and p<0.01, respectively); however, there was no difference between groups IAP-10 and IAP-20 (Fig. 2B). The expression of iNOS was found to be comparable between the three groups (3.05±0.88, 2.21±1.10, and 1.96±0.93 in groups IAP-0, IAP-10, and IAP-20, respectively) (Fig. 2C). There were no significant differences in Arg I expression among the three groups (2.02±0.80, 1.34±0.50, and 1.25±0.23 in groups IAP-0, IAP-10, and IAP-20, respectively) (Fig. 2D). Arg II expression was significantly higher in group IAP-20 than in group IAP-0 (5.03±1.53 vs. 1.30±0.61; p<0.05); however, there was no difference between groups IAP-0 and IAP-10 or IAP-10 and IAP-20 (Fig. 2E).
The activity of eNOS was significantly lower in groups IAP-10 and IAP-20 than in group IAP-0 (7.18±1.78 U/L and 5.06±1.49 U/L vs. 11.88±3.69 U/L, respectively; p<0.05 and p<0.001, respectively), with no difference between groups IAP-10 and IAP-20 (Fig. 3A). The activity of iNOS was significantly higher in group IAP-20 than in groups IAP-0 and IAP-10 (20.91±4.97 vs. 6.85±2.16 and 9.68±2.84, respectively; p<0.001 in both) (Fig. 3B). Arginase activity was significantly higher in group IAP-20 than in groups IAP-0 and IAP-10 (0.54±0.67 U/L vs. 0.09±0.05 U/L and 0.09±0.04 U/L, respectively; p<0.05 for both). However, there was no significant difference in arginase activity between groups IAP-0 and IAP-10 (Fig. 3C).
IL-1β concentration was significantly higher in group IAP-20 than in group IAP-0 (11.03±2.40 vs. 4.57±1.26; p<0.01) (Fig. 4A). IL-6 concentrations were significantly higher in group IAP-20 than in groups IAP-0 and IAP-10 (55.16±15.02 vs. 21.20±5.86 and 34.54±10.38, respectively; p<0.01 and p<0.05, respectively); however, there was no difference between groups IAP-0 and IAP-10 (Fig. 4B). TNF-α was significantly higher in groups IAP-10 and IAP-20 than in group IAP-0 (19.22±6.01 and 22.24±5.89 vs. 7.89±3.34, respectively; p<0.05 and p<0.01, respectively), while there was no difference between groups IAP-10 and IAP-20 (Fig. 4C).
An analysis of the histopathological scores for rat lung tissue from the three groups is shown in Table 1. Total scores of lung injury were significantly higher in group IAP-20 than in groups IAP-0 and IAP-10 (p<0.001 in both), while there was no difference between groups IAP-0 and IAP-10 (Fig. 5A). Lung sections stained with H&E revealed normal structures in the IAP-0 group (Fig. 5B), while inflammatory cell infiltration with protein exudation and alveolus collapse were obvious in the IAP-20 group (Fig. 5D). The lung tissue of group IAP-10 did not show any significant histopathological changes, with only a subtle increase in cellularity (Fig. 5C).
The results of this study support the well known increase in oxidative stress and ischemia-reperfusion injury caused by laparoscopic surgery and show that the level of plasma NO is decreased after 2 hours of CO2 pneumoperitoneum. This drop in plasma NO levels seems to be mainly due to the decreased expression and activity of eNOS, while the increase in iNOS activity may be related to increased inflammation and tissue injury. Most importantly, our findings provide novel information regarding the increased expression and activity of arginase as an underlying mechanism of decreased NO availability during laparoscopic surgery.
The involvement of the NO system and the role of endothelium-derived NO in the changes in renal function after CO2 pneumoperitoneum were suggested by several recent studies. Essentially, blockade of NOS with L-NAME was found to aggravate pneumoperitoneum-induced renal hypoperfusion and oliguria, while pretreatment with nitroglycerine attenuated this effect.29 Bishara, et al.18 reported the importance of IAP by showing that blockade of the NO system with L-NAME had adverse renal effects at high yet not moderate IAPs. Moreover, they found in another study that rats with decompensated congestive heart failure were more susceptible to the adverse renal effects of pneumoperitoneum when pretreated with L-NAME, implying a higher susceptibility to kidney function deterioration in patients with cardiovascular diseases.10 The results of our study support these previous studies by showing actual decreases in the expression and activity of eNOS with increasing IAP. This would have resulted in a diminished amount of endothelium-derived NO and thus a decrease in its anti-inflammatory and antioxidant actions. However, plasma nitrite levels were not found to decrease proportionately with IAP but were comparable between groups IAP-10 and IAP-20. This seems to reflect the induction of iNOS by an increase in proinflammatory cytokines IL-1β, IL-6, and TNF-α and a compensatory increase in NO secretion in response to worsening hypoperfusion.19
While the results of the present study show that eNOS and iNOS activity changes in opposite directions during CO2 pneumoperitoneum, existing evidence related to iNOS has indicated controversial results. Although Romeo, et al.20 found increased iNOS activity and overexpression of iNOS mRNA after 1 hour of CO2 pneumoperitoneum, Hajri, et al.21 reported that 2 hours of CO2 pneumoperitoneum led to the depression of iNOS mRNA production in rats. Romeo, et al. suggested a time-dependent effect, due to which the duration of CO2 pneumoperitoneum for 2 hours in the former study may have exhausted macrophage function and therefore iNOS activity. Further studies performed using various lengths of CO2 pneumoperitoneum are needed to shed more light on the different roles of eNOS and iNOS in oxidative stress caused by laparoscopic surgery.
Arginase has received widespread interest as a promising therapeutic target in a wide range of physiological and pathophysiological conditions, such as vascular disease, pulmonary disease, infectious disease, and cancer.22 Moreover, the functional significance of arginase and its relevance with the activity of NOS was emphasized due to the fact that arginase and NOS share L-arginine as a substrate.11 However, while oxidative stress, tissue injury, and reduction in kidney function during laparoscopic surgery have been suggested to be closely related to the NO system in several recent studies,1918 the role of arginase during CO2 pneumoperitoneum has remained unknown. Considering the fact that competition for L-arginine supply is intensified under acute and chronic stress conditions,23 arginase may contribute to the shortage of NO bioactivity during laparoscopic surgery. As expected, the results of this study showed a significant increase in arginase activity at the high insufflation pressure of 20 mm Hg. Arginase exists in two isoforms in mammals as Arg I and Arg II, which seem to be expressed at different sites to different degrees under various pathophysiological conditions. Although there is evidence suggesting that the predominant isoform detected in human endothelium is Arg II,22 both types are reported to be involved in endothelial dysfunction and the downregulation of NO synthesis to some extent.131415 Interestingly, the expression of Arg I did not differ among the three groups, while Arg II showed a trend of higher expression levels with increasing IAPs in the present study. This may implicate a stronger association of Arg II than of Arg I with increased arginase activity and impaired endothelial NO production during CO2 pneumoperitoneum, suggesting that Arg II may be a more effective therapeutic target for the attenuation of adverse effects of laparo-scopic surgery.
CO2 pneumoperitoneum has been reported to induce oxidative stress not only in the intra-abdominal organs but also in the lungs. An increase in MDA and oxidatively modified proteins in the lung tissue of rats that underwent laparoscopy with CO2 pneumoperitoneum was demonstrated in previous studies.2425 Also, CO2 pneumoperitoneum maintained at 15 mm Hg for only 30 minutes was found to cause diffuse intra-alveolar hemorrhage, dense congestion, and leukocyte infiltration with slight alveolar edema in the lung tissue of rats.26 Although NO production through the iNOS isoform has been suggested to play a deleterious role in drug-induced lung injury,172728 the present study demonstrated the concomitant increase in iNOS and lung injury after CO2 pneumoperitoneum for the first time. Moreover, concentrations of IL-1β, IL-6, and TNF-α were all found to show increasing trends with higher IAPs, with all three cytokines significantly higher at 20 mm Hg than at 0 mm Hg. This increase in inflammatory cytokines seems be responsible for iNOS induction and, therefore, the increase in systemic inflammation and oxidative stress as well as local damage in the lung tissue. Although the use of laparoscopy has been found to lead to less production of biochemical markers of surgical stress than open procedures,293031 our results indicate that lower pressures should be employed when possible.
It is noteworthy that splanchnic ischemia due to oxidative stress has even been reported in previously healthy patients undergoing laparoscopic surgery1 and that it extends to extra-abdominal organs as well.24 The present study shows that arginase upregulation is clearly involved with the increased oxidative stress due to CO2 pneumoperitoneum, and this finding offers a potential target for treatment and prevention of ischemia-reperfusion injury during laparoscopic surgery. Recently, arginase inhibitors such as 2(S)-amino-6-boronohexanoic acid (ABH) or Nω-hydroxy-nor-L-arginine (nor-NOHA) have been found to be effective for the treatment of various cardiovascular diseases32 and even protection against hepatic33 and cardiac34 ischemia-reperfusion injury in experimental and clinical studies. Further studies on the application of arginase inhibitors in laparoscopic surgery for protection against oxidative stress due to ischemia-reperfusion may yield positive results leading to safer surgery in high-risk patients.
This study has several limitations. First, while the results of this study clearly show a reciprocal action between NOS and arginase during CO2 pneumoperitoneum, uncovering the mechanism underlying the exact interaction between the two enzymes and their subtypes was beyond the scope of this study. The exact role of arginase and its metabolism in the human body itself is not yet completely understood, and further research is needed to elucidate the significance of this enzyme in many pathophysiological states, including CO2 pneumoperitoneum. Secondly, many other factors that may affect the extent of oxidative stress, such as different durations of CO2 pneumoperitoneum, the nature of the gas used, and the position of the patient, were not studied. Due to the experimental design of this study, we were not able to evaluate the changes in the activity and expression of enzymes or levels of oxidative stress in a sequential manner; rather, our evaluation only took place at a certain time point. We were also not able to differentiate the metabolic effects of CO2 from the effect of increased IAP. A study with subdivided groups is needed to provide more information regarding the impact of various factors that may lead to different amounts of oxidative stress. Thirdly, this study was conducted in healthy rats; therefore, the results may not accurately represent the patterns of eNOS, iNOS, or arginase expression in humans or diseased conditions. While arginase is constitutively expressed in endothelial cells, the expression of specific isoforms is known to differ among mammalian species.22 Lastly, the expression of iNOS was studied only with lung tissue in this study. The main cellular source for iNOS during the inflammatory process is thought to be macrophages;6 therefore, studying iNOS expression and activity in peritoneal macrophages may have offered more insight into its involvement during CO2 pneumoperitoneum.
In conclusion, our study shows that while eNOS activity is decreased during CO2 pneumoperitoneum, the activity of iNOS is significantly increased. This contributes to the increased oxidative stress and inflammation due to CO2 pneumoperitoneum, which is evidenced by the increase in inflammatory cytokines and histopathological changes in lung tissue. Moreover, our findings demonstrate that arginase expression and activity is increased during CO2 pneumoperitoneum, which seems to act reciprocally in relation to the NO system. These results offer a new potential target for attenuating the adverse outcomes of laparoscopic surgery. Studies conducted in subjects of diseased states such as congestive heart failure or vascular diseases may help to anticipate the possible effectiveness of arginase inhibition during laparoscopic surgery in patients at high risk of oxidative stress and its side effects.
Figures and Tables
Fig. 1
Effect of CO2 pneumoperitoneum at different IAPs on plasma nitrite and tissue MDA concentrations. (A) Plasma nitrite levels were significantly lower in groups IAP-10 and IAP-20 than in group IAP-0. There was no difference between groups IAP-10 and IAP-20. (B) MDA levels were significantly higher in group IAP-20 than in groups IAP-0 and IAP-10. There was no difference between groups IAP-0 and IAP-10. *p<0.01, **p<0.001. MDA, malondialdehyde; IAP, intra-abdominal pressure.
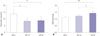
Fig. 2
Effect of CO2 pneumoperitoneum at different IAPs on eNOS, iNOS, Arg I, and Arg II protein expression. (A) Western blot for eNOS, iNOS, Arg I, Arg II, and β-actin (internal reference) in rat aorta tissue after different intra-abdominal pressures. (B) The expression of eNOS was significantly more suppressed in groups IAP-10 and IAP-20 than in group IAP-0, while there was no difference between groups IAP-10 and IAP-20. (C) There were no significant differences in iNOS expression among the three groups. (D) There were no significant differences in Arg I expression among the three groups. (E) Arg II expression was significantly higher in group IAP-20 than in group IAP-0. *p<0.05, **p<0.01. IAP, intra-abdominal pressure; NOS, nitric oxide synthase; eNOS, endothelial NOS; iNOS, inducible NOS; Arg, arginase.
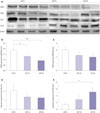
Fig. 3
Effect of CO2 pneumoperitoneum at different IAPs on eNOS, iNOS, and arginase activity. (A) The activity of eNOS was significantly lower in groups IAP-10 and IAP-20 than in group IAP-0, with no difference between groups IAP-10 and IAP-20. (B) The activity of iNOS was significantly higher in group IAP-20 than in groups IAP-0 and IAP-10. (C) Arginase activity was significantly higher in group IAP-20 than in groups IAP-0 and IAP-10. No difference was seen between groups IAP-0 and IAP-10. *p<0.05, **p<0.01, ***p<0.001. IAP, intra-abdominal pressure; NOS, nitric oxide synthase; eNOS, endothelial NOS; iNOS, inducible NOS.
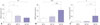
Fig. 4
Effect of CO2 pneumoperitoneum at different IAPs on proinflammatory cytokine concentrations. (A) IL-1β was significantly higher in group IAP-20 than in group IAP-0. (B) IL-6 was significantly higher in group IAP-20 than in groups IAP-0 and IAP-10; however, there was no difference between groups IAP-0 and IAP-10. (C) TNF-α was significantly higher in groups IAP-10 and IAP-20 than in IAP-0, while there was no difference between groups IAP-10 and IAP-20. *p<0.05, **p<0.01. IAP, intra-abdominal pressure; IL, interleukin; TNF, tumor necrosis factor.
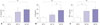
Fig. 5
The effects of different IAPs on histopathological changes in CO2 pneumoperitoneum-induced lung injury in rats. (A) Total scores of lung injury were significantly higher in group IAP-20 than in groups IAP-0 and IAP-10. (B) Normal lung tissue is seen in the IAP-0 group. (C) A slight increase in cellularity (arrows) is seen in the IAP-10 group. (D) Inflammatory cell infiltration (arrows) with protein exudation (asterisks) and alveolus collapse is seen in the IAP-20 group. *p<0.001. IAP, intra-abdominal pressure.
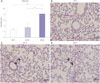
ACKNOWLEDGEMENTS
This study was supported by a faculty research grant from Yonsei University College of Medicine for 2010 (6-2010-0163).
References
1. Sammour T, Mittal A, Loveday BP, Kahokehr A, Phillips AR, Windsor JA, et al. Systematic review of oxidative stress associated with pneumoperitoneum. Br J Surg. 2009; 96:836–850.


2. Abassi Z, Bishara B, Karram T, Khatib S, Winaver J, Hoffman A. Adverse effects of pneumoperitoneum on renal function: involvement of the endothelin and nitric oxide systems. Am J Physiol Regul Integr Comp Physiol. 2008; 294:R842–R850.


3. Ishizaki Y, Bandai Y, Shimomura K, Abe H, Ohtomo Y, Idezuki Y. Changes in splanchnic blood flow and cardiovascular effects following peritoneal insufflation of carbon dioxide. Surg Endosc. 1993; 7:420–423.


4. Nickkholgh A, Barro-Bejarano M, Liang R, Zorn M, Mehrabi A, Gebhard MM, et al. Signs of reperfusion injury following CO2 pneumoperitoneum: an in vivo microscopy study. Surg Endosc. 2008; 22:122–128.


5. Demyttenaere S, Feldman LS, Fried GM. Effect of pneumoperitoneum on renal perfusion and function: a systematic review. Surg Endosc. 2007; 21:152–160.


6. Förstermann U, Sessa WC. Nitric oxide synthases: regulation and function. Eur Heart J. 2012; 33:829–837.


7. Ali NA, Eubanks WS, Stamler JS, Gow AJ, Lagoo-Deenadayalan SA, Villegas L, et al. A method to attenuate pneumoperitoneum-induced reductions in splanchnic blood flow. Ann Surg. 2005; 241:256–261.


8. Shimazutsu K, Uemura K, Auten KM, Baldwin MF, Belknap SW, La Banca F, et al. Inclusion of a nitric oxide congener in the insufflation gas repletes S-nitrosohemoglobin and stabilizes physiologic status during prolonged carbon dioxide pneumoperitoneum. Clin Transl Sci. 2009; 2:405–412.


9. Bishara B, Karram T, Khatib S, Ramadan R, Schwartz H, Hoffman A, et al. Impact of pneumoperitoneum on renal perfusion and excretory function: beneficial effects of nitroglycerine. Surg Endosc. 2009; 23:568–576.


10. Bishara B, Abu-Saleh N, Awad H, Goltsman I, Ramadan R, Khamaysi I, et al. Pneumoperitoneum aggravates renal function in cases of decompensated but not compensated experimental congestive heart failure: role of nitric oxide. J Urol. 2011; 186:310–317.


11. Durante W, Johnson FK, Johnson RA. Arginase: a critical regulator of nitric oxide synthesis and vascular function. Clin Exp Pharmacol Physiol. 2007; 34:906–911.


12. Berkowitz DE, White R, Li D, Minhas KM, Cernetich A, Kim S, et al. Arginase reciprocally regulates nitric oxide synthase activity and contributes to endothelial dysfunction in aging blood vessels. Circulation. 2003; 108:2000–2006.


13. Kim JH, Bugaj LJ, Oh YJ, Bivalacqua TJ, Ryoo S, Soucy KG, et al. Arginase inhibition restores NOS coupling and reverses endothelial dysfunction and vascular stiffness in old rats. J Appl Physiol (1985). 2009; 107:1249–1257.


14. Santhanam L, Lim HK, Lim HK, Miriel V, Brown T, Patel M, et al. Inducible NO synthase dependent S-nitrosylation and activation of arginase1 contribute to age-related endothelial dysfunction. Circ Res. 2007; 101:692–702.


15. Ryoo S, Gupta G, Benjo A, Lim HK, Camara A, Sikka G, et al. Endothelial arginase II: a novel target for the treatment of atherosclerosis. Circ Res. 2008; 102:923–932.
16. Förstermann U, Münzel T. Endothelial nitric oxide synthase in vascular disease: from marvel to menace. Circulation. 2006; 113:1708–1714.
17. Kristof AS, Goldberg P, Laubach V, Hussain SN. Role of inducible nitric oxide synthase in endotoxin-induced acute lung injury. Am J Respir Crit Care Med. 1998; 158:1883–1889.


18. Bishara B, Ramadan R, Karram T, Awad H, Abu-Saleh N, Winaver J, et al. Nitric oxide synthase inhibition aggravates the adverse renal effects of high but not low intraabdominal pressure. Surg Endosc. 2010; 24:826–833.


19. Ozmen MM, Zulfikaroglu B, Col C, Cinel I, Isman FK, Cinel L, et al. Effect of increased abdominal pressure on cytokines (IL1 beta, IL6, TNFalpha), C-reactive protein (CRP), free radicals (NO, MDA), and histology. Surg Laparosc Endosc Percutan Tech. 2009; 19:142–147.


20. Romeo C, Impellizzeri P, Antonuccio P, Turiaco N, Cifalá S, Gentile C, et al. Peritoneal macrophage activity after laparoscopy or laparotomy. J Pediatr Surg. 2003; 38:97–101.


21. Hajri A, Mutter D, Wack S, Bastien C, Gury JF, Marescaux J, et al. Dual effect of laparoscopy on cell-mediated immunity. Eur Surg Res. 2000; 32:261–266.


22. Morris SM Jr. Recent advances in arginine metabolism: roles and regulation of the arginases. Br J Pharmacol. 2009; 157:922–930.


23. Luiking YC, Ten Have GA, Wolfe RR, Deutz NE. Arginine de novo and nitric oxide production in disease states. Am J Physiol Endocrinol Metab. 2012; 303:E1177–E1189.


24. Pross M, Schulz HU, Flechsig A, Manger T, Halangk W, Augustin W, et al. Oxidative stress in lung tissue induced by CO(2) pneumoperitoneum in the rat. Surg Endosc. 2000; 14:1180–1184.


25. Sare M, Hamamci D, Yilmaz I, Birincioglu M, Mentes BB, Ozmen M, et al. Effects of carbon dioxide pneumoperitoneum on free radical formation in lung and liver tissues. Surg Endosc. 2002; 16:188–192.


26. Karapolat S, Gezer S, Yildirim U, Dumlu T, Karapolat B, Ozaydin I, et al. Prevention of pulmonary complications of pneumoperitoneum in rats. J Cardiothorac Surg. 2011; 6:14.


27. Lin CC, Hsieh NK, Liou HL, Chen HI. Niacinamide mitigated the acute lung injury induced by phorbol myristate acetate in isolated rat's lungs. J Biomed Sci. 2012; 19:27.


28. Ni YF, Kuai JK, Lu ZF, Yang GD, Fu HY, Wang J, et al. Glycyrrhizin treatment is associated with attenuation of lipopolysaccharide-induced acute lung injury by inhibiting cyclooxygenase-2 and inducible nitric oxide synthase expression. J Surg Res. 2011; 165:e29–e35.


29. Mealy K, Gallagher H, Barry M, Lennon F, Traynor O, Hyland J. Physiological and metabolic responses to open and laparoscopic cholecystectomy. Br J Surg. 1992; 79:1061–1064.


30. Cho JM, LaPorta AJ, Clark JR, Schofield MJ, Hammond SL, Mallory PL 2nd. Response of serum cytokines in patients undergoing laparoscopic cholecystectomy. Surg Endosc. 1994; 8:1380–1383.


31. Glaser F, Sannwald GA, Buhr HJ, Kuntz C, Mayer H, Klee F, et al. General stress response to conventional and laparoscopic cholecystectomy. Ann Surg. 1995; 221:372–380.


32. Pernow J, Jung C. Arginase as a potential target in the treatment of cardiovascular disease: reversal of arginine steal. Cardiovasc Res. 2013; 98:334–343.

