Abstract
Kawasaki disease (KD) is characterized with acute systemic vasculitis, occurs predominantly in children between 6 months to 5 years of age. Patients with this disease recover well and the disease is self-limited in most cases. Since it can lead to devastating cardiovascular complications, KD needs special attention. Recent reports show steady increases in the prevalence of KD in both Japan and Korea. However, specific pathogens have yet to be found. Recent advances in research on KD include searches for genetic susceptibility related to KD and research on immunopathogenesis based on innate and acquired immunity. Also, search for etiopathogenesis and treatment of KD has been actively sought after using animal models. In this paper, the recent progress of research on KD was discussed.
Kawasaki disease (KD) was first reported in 1967 by T. Kawasaki, a Japanese pediatrician.1 The first Korean KD patient was reported in 1973.2 Since then, the incidence of this disease has increased continuously. KD is characterized with acute systemic vasculitis that occurs predominantly in children between 6 months to 5 years of age. Although the clinical symptoms of KD are well characterized, the causes of this disease are not yet known. For this reason, KD is usually diagnosed by clinical symptoms, such as a fever for 5 days or more, bilateral nonpurulent conjunctival congestion, changes of the lips and oral mucosa, polymorphous exanthema along the trunk, changes of the peripheral extremities and subsequent desquamation of finger tips, and nonpurulent cervical lymphadenopathy over 1.5 cm in size (Table 1). Other than these criteria, erythema on Bacille Calmette-Guérin (BCG) vaccination sites is also thought to be an important finding in children younger than the age of 2 years.3 This is an especially important finding for KD patients in Korea, since BCG vaccination is included in a national immunization program (NIP) during the neonatal period.
Patients with this disease recover well, and the disease is self-limited in most cases. However, since it can lead to devastating cardiovascular complications, KD carries great clinical importance and needs special attention. Among cardiovascular complications, coronary artery lesions in particular can lead to long term morbidity and mortality. Without proper treatment in a timely manner during the acute phase of coronary artery complications, about 20% of patients develop coronary artery aneurysm. Giant aneurysm, with a diameter exceeding 8 mm, is known to develop in about 0.5-1% of KD patients. Once a giant aneurysm is formed, recovery is almost impossible and can result in coronary stenosis, obstruction, or acute thrombosis. These complications cause ischemic heart disease and/or myocardial infarction later and may also cause rare but fatal sudden cardiac death in children.45 In particular, occurrence of myocardial infarction results in poor prognosis with reported mortality after the first myocardial infarction at 22% and with subsequent episodes of myocardial infarction causing 70-80% mortality.6
In developed countries, including South Korea, incidences of congenital heart disease are significantly diminishing, although KD still ranks as the most common cause for acquired heart disease in children. In fact, the prevalence of KD in South Korea is second only to Japan. Recent reports show steady increases in the prevalence of KD per 100000 children less than 5 years old: 86.4 in 2000 to 2002, 105.0 in 2003 to 2005, 113.1 in 2006 to 2008, and 134.4 in 2011. Hence, KD is not regarded as a rare disease.7 Interestingly, while Japan and South Korea show continuous increase in incidence,7 United States and Canada showed constant prevalences without increase.8
The etiology of KD has been studied extensively, and viral or bacterial infections, autoimmune factors, and genetic factors have been suggested. It is noteworthy to mention high prevalence of KD in children between 6 months and 5 years old, when maternal immune function decreases. Other points to note include a self-limited clinical course without specific treatment and the epidemic nature of occurrence patterns. These points raise suspicions of an infectious disease; however, specific pathogens have yet to be found.
Almost 50 years have passed since KD was first recognized. Recent advances in research on KD include searches for genetic susceptibility related to KD and research on immunopathogenesis based on the idea that innate immunity has a more prominent role than the acquired immunity. Also, search for etiopathogenesis and treatment of KD has been actively sought after using animal models. This paper will present the recent progress of research on KD.
In understanding the pathophysiology of KD, genetic susceptibility has received much attention owing to the likely occurrence patterns in specific races and families. According to data published in 2005, incidence rates per 100000 children under age of 5 were 134.2 for Japan, 104.6 for Korea, and 66 for Taiwan, compared to reports of 18.5 in US, 8.1 in UK, and 6.2 in Sweden. Also, according to reports of an epidemiological survey in California, US from 1995 to 1999, the incidence rate per 100000 children under the age of 5 years old was 15.3. However, the incidence rates according to ethnic background were as follows: 35.3 for Asians, 24.6 for Blacks, 14.7 for Caucasians, 9.6 for Hispanics, and 8.6 for Native Americans.9 In an epidemiology survey taken in Hawaii from 1996 to 2001, incidence rates per 100000 children under the age of 5 were 35.3 for Caucasians and 197.7 for Japanese living in Hawaii. This incidence rate for Japanese children was even higher than the incidence rate in Japan and shows that prevalence among races is clearly different.10
Reports also have been made on family history. Matsubara, et al.11 have reported KD occurred five times in three siblings among one family within 6 years. Several studies have found incidence rates among siblings to be 1.4 to 2.1%; this is much higher than that for the general population.1213 Also, as the first generation patients with initial KD go into reproductive years, increasing incidences in their children, a familial incidence rate, have been reported.14 These differences in ethnicity and family suggest genetic factors play a strong role in the development of KD.
Initial research on genetic susceptibility has mainly focused on human leukocyte antigen (HLA) and allotypes of immunoglobulin. HLA-DRB1, HLA B5, Bw51, and Bw4415161718 and kappachainallotype Km1 and heterozygocity of Km1 with Gm and etc.19 are reported in association with susceptibility to KD. Huang, et al.20 reported HLA-DRB1 is not associated with coronary aneurysm in Taiwanese patients, and Barron, et al.21 reported that no association could be found between HLA class II allele and KD.
Recently, many studies on various genes associated with different immunologic reactions among diverse groups have been undertaken. Associations with ethnicity, region, and gender have shown different results, and have been interpreted as differences in genetic background among ethnic groups.
Searches for genes associated with KD are still ongoing and Table 2 lists the candidate genes that have been studied to date.2223
Ongoing genetic research has sought to find associations with susceptibility to KD, severity of KD, contraindications to immunoglobulin treatment, and development of coronary artery complications. With the start of genome-wide association studies (GWAS), remarkable progress has been made. Currently, the most interesting candidates of KD-susceptibility genes are the inositol 1,4,5-triphosphate 3-kinase (ITPKC) gene, caspase-3 (CASP3) gene, B lymphocyte kinase (BLK), CD40 gene, Fc fragment of IgG low affinity IIa receptor (FCGR2A), and HLA. Recent reports from meta-analysis of GWASes from Japan, Taiwan, and Korea have shown that the above genes hold significant value within these three countries (Table 3).24 Differences in single nucleotide polymorphisms (SNPs) of genes, excluding previously reported SNPs of ITPKC genes by Onouchi,25 were reported. Onouchi25 also reported different risk allele frequencies among Japan, Taiwan, and Europe populations, which reflect differences between ethnicity.
Japan shows the highest incidence of KD worldwide, and a nationwide GWAS was executed on 78 families with KD among siblings. The results showed the strongest associations in 12q24 and 19q13.2 regions.26 In 2008, this research team surveyed and analyzed 1222 SNPs from 1671 Japanese individuals (KD patients: 637, normal control: 1034) and 209 family members with a family history of KD. In doing so, functional SNPs with highly significant associations with KD were found in ITPKC, which is present in the 19q13.2 region of the human chromosome. This result was also confirmed through family analysis. These results showed similar trends in not only Japanese, but American children as well. Thereafter, the functional SNP found and analyzed by the study has been reported to have a high susceptibility to KD and to increase risk of coronary complications in both Eastern and Western populations.27
ITPKC, which is a secondary messenger molecule involved in the Ca++/NFAT signal pathway, acts as a negative regulator of T-cell activation. ITPKC polymorphism results in increased activation of T cells with an increased expression of cytokines.27 This can result in more prolonged expansion of proinflammatory T cells during the acute phase, which may lead to KD susceptibility and disease severity.
However, in Koreas, researchers have yet to find a significant polymorphism of ITPKC genes. Rather, the rs4236089 C allele in chloride intracellular channel 5 (CLIC5), located at 6p21.1, has been reported to be very important.28 This discrepancy may be because Japanese and Americans have different genetic backgrounds than Koreans. Reports from Taiwan also show differences in polymorphism incidences of ITPKC genes attributed to differences in ethnicity.29 Interestingly, Taiwan study results showed an association between ITPKC gene polymorphism and erythema of BCG vaccination sites. This observation requires further investigation to see whether other gene polymorphisms exert synergistic effects.
Onouchi, et al.30 also reported that a SNP within the CASP3 gene located on 4q34-35 was associated with KD susceptibility by a positional candidate gene study. This was significantly associated with KD susceptibility in both Japanese and Euro-American populations. CASP3 is associated with apoptosis of immature cells. The G to A substitution within exon 1 of CASP3 (rs113420705) reduces the binding of NFAT to the DNA surrounding the SNP and then decreases the transcription of CASP3 mRNA, which in turn activates immune cells to increase cytokines expression. This might lead to increased susceptibility of KD. However, CASP3 gene polymorphism has not been shown to be associated with development of coronary arterial lesion (CAL) or inadequate response to IVIG.
Interestingly, functional SNPs in both ITPKC and CASP3 genes confer unresponsiveness of patients to IVIG and risk for CAL.31 This might be from synergistic effects of genetic variants of ITPKC and CASP3, which could be observed in other diseases.3233 Based on these results, administration of cyclosporine A for the treatment of IVIG-resistant KD patients is theoretically feasible, because cyclosporine A suppresses activation of NFAT. Currently, cyclosporine A is already being used34 in treatment and further research projects are ongoing.
Another study showed a strong association for the susceptibility of KD with rs1801274 on FCGR2A.35 FCGR2A is expressed on the surface of various immune cells, such as dendritic cells, monocytes, macrophages, and neutrophils, and transduces activation signals into the cell when ligated with immune complexes.36 Evidence of immune activation during the acute phase of KD might be activated upon signaling through FCGR2A ligation with circulating immune complex, which can be found in the sera of KD patients.37
In 2012, two GWAS studies3538 independently reported significant associations with KD that were observed in the FAM167A-BLK region on chromosome 8p23-p22. The association peak in this locus was located at the intergenic region between BLK and FAM167A. BLK is a src family tyrosine kinase expressed mainly in B cells and involved in B cell receptor signal transduction.39 This may indicate possible involvement of B cells for the development of KD.4041 Moreover, BLK is required in the development of IL-17-producing γδT cells in mice.42 During the acute phase of KD, increased IL-17 levels have been reported,43 which might be associated with polymorphism of BLK. This might be associated with dysregulation of Th17/Treg cell imbalance in patients in patients with KD.44 However, the function of FAM167A molecule is unclear.
The association KD and SNPs around CD40 located on chromosome 20q12-q13.2 have also been reported in a Japanese GWAS38 and a Taiwanese GWAS.45 CD40 is expressed on B cells, as well as on monocytes/macrophages, endothelial cells, epithelial cells, smooth muscle cells, fibroblasts, and adipocytes. Following activation, its receptor, CD40L is upregulated. Elevated expression of CD40L during the acute phase of KD and significantly higher expression thereof in KD patients with CALs have been reported.46 It is likely that CD40-CD40L signal pathways may play a facilitating role in the disease progression of KD and could be a novel target of molecular therapies.
The association of HLA alleles with susceptibility to diseases, especially autoimmune diseases, is well known. Recently, a significant association of the SNPs in the HLA class II region, HLA-DQB2, and HLA-DOB, with KD was reported.38
Striking immune derangement has been reported to occur in acute KD, including various changes of immune cells, marked cytokine cascade stimulation, and endothelial cell activation (Table 4).
As shown in Table 4, many kinds of proinflammatory cytokines and chemokines are increased during the acute phase of KD. The key processes leading to coronary arteritis are still being clarified, although endothelial cell activation, activation of CD68+ monocytes/macrophages, CD8+ T lymphocytes, and oligoclonal IgA plasma cells appear to be involved in the development of coronary artery arteritis.484950 The infiltration of IgA plasma cells in the respiratory tract, which is similar to findings in fatal viral respiratory infections, suggests a respiratory portal entry of an etiologic agent or agents.51 Rodó, et al.52 suggested that a KD agent is transported by the wind, possibly from a shared source region, across the Pacific. This hypothesis may support the plausibility of the entry of causative agents through the respiratory tract leading to the development of KD. Enzymes, such as MMPs,5354 that are capable of damaging arterial cell wall integrity could be important in the development of aneurysmal dilatation of coronary arteries. CD40L, VGEF, MCP-1, and various cytokines also could play important roles in the development of vasculitis.46555657585960 With these results, Kim47 proposed important roles of acquired immune events in the development of vasculitis in KD.
Meanwhile, increased expression of toll-like receptor 2 (TLR2) on the peripheral blood monocytes suggests that innate immunity may be involved in the pathogenesis of KD.61 Ikeda, et al.62 also reported that innate immunity may be involved in the pathogenesis or pathophysiology of vasculitis in KD. Nishio, et al.63 reported that the pathological findings of Nod1 ligand-induced coronary arteritis in mice were consistent with those of human coronary artery lesions in the acute phase of KD. With this result, they suggest that the innate immune system could play an important role in the development of KD. Kusuda, et al.64 reported that serum KD-specific molecules possess molecular structures common to microbe-associated molecular pattern (MAMP) from Bacillus cereus, Bacillus subtilis, Yersinia pseudotuberculosis, and Staphylococcus aureus, and these molecules decreased after treatment with IVIG. Dr. Hara65 proposed that increased MAMPS and damage-associated molecular patterns (DAMPs) in the sera of acute phase patients with KD activate the immune system and vascular cells through innate immune pattern recognition receptor (PRR) to release chemokines and cytokines, thereby inducing KD in genetically predisposed individuals.
Researchers have faced some obstacles in outlining the etiopathogenesis and treatment modalities for KD. First, usually KD patients come to the hospital after development of full-blown clinical symptoms. Thus, clinical samples can only be obtained from progressed stages of the disease. Second, tissue biopsy from coronary arteries, in which the most important complication of the disease occurs, cannot be obtained easily. Also, tissue samples of coronaries from autopsy are rare. Third, due to the peak incidence at an early age, collecting blood samples from KD patients and obtaining informed consent from guardians are difficult. For these reasons, development of animal models of KD is needed.
In 1979, Murata66 developed the first animal model of KD in DD and DDY strains of mice induced by Candida albicans water soluble fraction (CAWS). After this report, much effort has been made to develop various animal models of KD (Table 5).67
Taken together with the results of research from murine models of KD, Takahashi, et al.76 suggested a hypothesis for the development of vasculitis. Upon α-mannan binding to Dectin-2, Syk activates NF-kB. The NF-kB activates cytokine genes, including pro-IL-1β, IL-23, and IL-12. These cytokines stimulate differentiation of Th17 cells. Via Th17 cells, neutrophils and macrophages are recruited to inflammatory sites, stimulating the production of various pro-inflammatory cytokines and chemokines. Also, various cytokines and chemokines stimulate the activation of endothelial cells and vascular smooth muscle cells. Inflammatory cells adhere to activated endothelium and migrate within the vascular wall. Thereby, vasculitis develops. Motomura, et al.77 recently reported cardiac CD11C+ macrophages, especially, play a pivotal role in the development of acute coronary arteritis in FK565-treated mice. This suggests innate immunity is important to the development of coronary vasculitis in KD. Nevertheless, further studies are needed to clarify the etiopathogenesis of KD.
Generally, KD patients without known cardiac sequelae during the first month of KD appear to return to their previous (usually excellent) state of health without signs or symptoms of cardiac impairment.78 However, identification of appropriate long term follow-up of these patients has proven difficult, because no clear picture of the potential sequelae of the disease without coronary artery aneurysms has emerged. Some authors have suggested that KD survivors involve increased arterial stiffness and are more likely to develop hypertension.7980 A Japanese registry study showed that for KD patients, mortality after the acute phase of the disease is increased only in males with early cardiac lesions, such as aneurysms. However, this group had a standardized mortality ratio of 2.6, with the major cause of death arising from noncardiac origins.8182 Recently, Holve, et al.83 also reported that long-term cardiac outcomes encompass a low rate of adverse cardiovascular events up to age 21. Meaningful knowledge about long-term myocardial function, late-onset valvular regurgitation, and coronary artery status in this population awaits careful surveillance in the future decades.
Though almost 50 years has passed since KD was first recognized, many topics remain to be clarified, including etiology, immunopathogenesis, genetics, development of coronary artery aneurysms, treatment of IVIG resistant patients, and long-term cardiac sequelae. In the future, overcoming KD will reflect the efforts of many researchers in various fields.
Figures and Tables
Table 1
Criteria for Diagnosis of KD

Table 2

Table 3
Novel Susceptibility Locus for KD from the Meta-Analysis of Three GWASes24

Gene | SNP | p value | SNP (Onouchi)25 |
---|---|---|---|
ITPKC | rs28493229 | 3.07×10-9 | rs28493229 |
CASP3 | rs2720377 | 2.66×10-9 | rs113420705 |
BLK | rs2736340 | 1.23×10-16 | rs2254546 |
CD40 | rs1883832 | 1.76×10-8 | rs4813003 |
HLA class 2 | rs189914842 | 4.57×10-11 | rs2857151 |
Table 4
Evidence of Derangement of the Immune System in Acute KD (Modified from Kim47)

Table 5
The History of Animal Models of KD (Modified from Chun, et al.67)
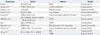
Researchers | Animal | Inducers | Results |
---|---|---|---|
Murata66 | DD an DDY mice | CAWS | Coronary arteritis |
Lehman, et al.68 | C57BL/6, BALB/c mice | Group B Lactobacillus | Coronary arteritis |
Felsburg, et al.69 | Young dogs | Naturally occurred | Necrotizing vasculitis |
Onouchi, et al.70 | Weanling rabbits | Serum sickness | Coronary arteria dilatation |
Duong, et al.71 | C57BL/6, BALB/c mice | Group B Lactobacillus casei cell wall extract | Coronary arteritis |
Takahashi, et al.72 | CD-1, C57BL/6N, C3H/HeN, DBA/2N, CBA/JN, BALB/c mice | CAWS | Systemic vasculitis |
Ohno73 | DBA/2, C3H/HeN mice | CAWS | Coronary arteritis |
Nakamura, et al.74 | C57BL/6 mice | BCG crude extract of M. intracellulare | Autoimmune vasculitis |
Schulte, et al.75 | C57BL/6, RAG1-/- B6, B-null mice | Group B Lactobacillus casei cell wall extract | Coronary arteritis |
Chun, et al.67 | PD-1-/- BALB/c, PD-1-/- C56BL/6 | BCG | Coronary arteritis |
HSP65 | Systemic vasculitis |
References
1. Kawasaki T. Acute febrile mucocutaneous syndrome with lymphoid involvement with specific desquamation of the fingers and toes in children. Arerugi. 1967; 16:178–222.
2. Park CS, Suh CJ, Cho SH, LEE DB. Muco-cutaneous lymphnode syndrome: five cases report. J Korean Pediatr Soc. 1973; 16:61–67.
3. Uehara R, Igarashi H, Yashiro M, Nakamura Y, Yanagawa H. Kawasaki disease patients with redness or crust formation at the Bacille Calmette-Guérin inoculation site. Pediatr Infect Dis J. 2010; 29:430–433.


4. Sugimura T, Yokoi H, Sato N, Akagi T, Kimura T, Iemura M, et al. Interventional treatment for children with severe coronary artery stenosis with calcification after long-term Kawasaki disease. Circulation. 1997; 96:3928–3933.


5. Kitamura S. The role of coronary bypass operation on children with Kawasaki disease. Coron Artery Dis. 2002; 13:437–447.


6. Kato H, Ichinose E, Kawasaki T. Myocardial infarction in Kawasaki disease: clinical analyses in 195 cases. J Pediatr. 1986; 108:923–927.


7. Park YW, Han JW, Hong YM, Ma JS, Cha SH, Kwon TC, et al. Epidemiological features of Kawasaki disease in Korea, 2006-2008. Pediatr Int. 2011; 53:36–39.


8. Uehara R, Belay ED. Epidemiology of Kawasaki disease in Asia, Europe, and the United States. J Epidemiol. 2012; 22:79–85.


9. Chang RK. Epidemiologic characteristics of children hospitalized for Kawasaki disease in California. Pediatr Infect Dis J. 2002; 21:1150–1155.


10. Holman RC, Curns AT, Belay ED, Steiner CA, Effler PV, Yorita KL, et al. Kawasaki syndrome in Hawaii. Pediatr Infect Dis J. 2005; 24:429–433.


11. Matsubara T, Furukawa S, Ino T, Tsuji A, Park I, Yabuta K. A sibship with recurrent Kawasaki disease and coronary artery lesion. Acta Paediatr. 1994; 83:1002–1004.


12. Yanagawa H, Nakamura Y, Yashiro M, Fujita Y, Nagai M, Kawasaki T, et al. A nationwide incidence survey of Kawasaki disease in 1985-1986 in Japan. J Infect Dis. 1988; 158:1296–1301.


13. Fujita Y, Nakamura Y, Sakata K, Hara N, Kobayashi M, Nagai M, et al. Kawasaki disease in families. Pediatrics. 1989; 84:666–669.


14. Uehara R, Yashiro M, Nakamura Y, Yanagawa H. Clinical features of patients with Kawasaki disease whose parents had the same disease. Arch Pediatr Adolesc Med. 2004; 158:1166–1169.


15. Krensky AM, Grady S, Shanley KM, Berenberg W, Yunis EJ. Epidemic and endemic HLA-B and DR associations in mucocutaneous lymph node syndrome. Hum Immunol. 1983; 6:75–77.


16. Kaslow RA, Bailowitz A, Lin FY, Koslowe P, Simonis T, Israel E. Association of epidemic Kawasaki syndrome with the HLA-A2, B44, Cw5 antigen combination. Arthritis Rheum. 1985; 28:938–940.


17. Krensky AM, Berenberg W, Shanley K, Yunis EJ. HLA antigens in mucocutaneous lymph node syndrome in New England. Pediatrics. 1981; 67:741–743.


18. Kato S, Kimura M, Tsuji K, Kusakawa S, Asai T, Juji T, et al. HLA antigens in Kawasaki disease. Pediatrics. 1978; 61:252–255.


19. Shulman ST, Melish M, Inoue O, Kato H, Tomita S. Immunoglobulin allotypic markers in Kawasaki disease. J Pediatr. 1993; 122:84–86.


20. Huang FY, Chang TY, Chen MR, Hsu CH, Lee HC, Lin SP, et al. Genetic variations of HLA-DRB1 and susceptibility to Kawasaki disease in Taiwanese children. Hum Immunol. 2007; 68:69–74.
21. Barron KS, Silverman ED, Gonzales JC, St Clair M, Anderson K, Reveille JD. Major histocompatibility complex class II alleles in Kawasaki syndrome--lack of consistent correlation with disease or cardiac involvement. J Rheumatol. 1992; 19:1790–1793.
23. Onouchi Y. Identification of susceptibility genes for Kawasaki disease. Nihon Rinsho Meneki Gakkai Kaishi. 2010; 33:73–80.


24. Johnson TA, Wu J, Yoon D, Hata A, Kubo M, Takahashi A. A Meta-analysis of Three Genome-wide Association Studies Identifies a Novel Susceptibility Locus for Kawasaki Disease. In : International Kawasaki Disease Symposium; Honolulu, Hawaii, USA. 2015. p. 93.
25. Onouchi Y. Genetics of Kawasaki disease: what we know and don't know. Circ J. 2012; 76:1581–1586.
26. Onouchi Y, Tamari M, Takahashi A, Tsunoda T, Yashiro M, Nakamura Y, et al. A genomewide linkage analysis of Kawasaki disease: evidence for linkage to chromosome 12. J Hum Genet. 2007; 52:179–190.


27. Onouchi Y, Gunji T, Burns JC, Shimizu C, Newburger JW, Yashiro M, et al. ITPKC functional polymorphism associated with Kawasaki disease susceptibility and formation of coronary artery aneurysms. Nat Genet. 2008; 40:35–42.


28. Kim KY, Jung MK, Bae Y, Ji W, Shin DJ, Woo B, et al. Genome-wide Association Study Identified New Susceptibility Loci to Coronary Artery Aneurysm-related in Kawasaki Disease. In : International Kawasaki Disease Symposium; Honolulu, Hawaii, USA. 2015. p. 94.
29. Lin MT, Wang JK, Yeh JI, Sun LC, Chen PL, Wu JF, et al. Clinical Implication of the C Allele of the ITPKC Gene SNP rs28493229 in Kawasaki Disease: Association With Disease Susceptibility and BCG Scar Reactivation. Pediatr Infect Dis J. 2011; 30:148–152.


30. Onouchi Y, Ozaki K, Buns JC, Shimizu C, Hamada H, Honda T, et al. Common variants in CASP3 confer susceptibility to Kawasaki disease. Hum Mol Genet. 2010; 19:2898–2906.


31. Onouchi Y, Suzuki Y, Suzuki H, Terai M, Yasukawa K, Hamada H, et al. ITPKC and CASP3 polymorphisms and risks for IVIG unresponsiveness and coronary artery lesion formation in Kawasaki disease. Pharmacogenomics J. 2013; 13:52–59.


32. Bae Y, Park C, Han J, Hong YJ, Song HH, Shin ES, et al. Interaction between GNB3 C825T and ACE I/D polymorphisms in essential hypertension in Koreans. J Hum Hypertens. 2007; 21:159–166.


33. Hiramatsu M, Oguri M, Kato K, Horibe H, Fujimaki T, Watanabe S, et al. Synergistic effects of genetic variants of APOA5 and BTN2A1 on dyslipidemia or metabolic syndrome. Int J Mol Med. 2012; 30:185–192.


34. Suzuki H, Terai M, Hamada H, Honda T, Suenaga T, Takeuchi T, et al. Cyclosporin A treatment for Kawasaki disease refractory to initial and additional intravenous immunoglobulin. Pediatr Infect Dis J. 2011; 30:871–876.


35. Khor CC, Davila S, Breunis WB, Lee YC, Shimizu C, Wright VJ, et al. Genome-wide association study identifies FCGR2A as a susceptibility locus for Kawasaki disease. Nat Genet. 2011; 43:1241–1246.


36. Nimmerjahn F, Ravetch JV. Fcgamma receptors as regulators of immune responses. Nat Rev Immunol. 2008; 8:34–47.
37. Mason WH, Jordan SC, Sakai R, Takahashi M, Bernstein B. Circulating immune complexes in Kawasaki syndrome. Pediatr Infect Dis. 1985; 4:48–51.


38. Onouchi Y, Ozaki K, Burns JC, Shimizu C, Terai M, Hamada H, et al. A genome-wide association study identifies three new risk loci for Kawasaki disease. Nat Genet. 2012; 44:517–521.


39. Bewarder N, Weinrich V, Budde P, Hartmann D, Flaswinkel H, Reth M, et al. In vivo and in vitro specificity of protein tyrosine kinases for immunoglobulin G receptor (FcgammaRII) phosphorylation. Mol Cell Biol. 1996; 16:4735–4743.


40. Kim DS, Han BH, Lee SK, Lee HK, Chwae YJ, Lee KY. Evidence for selection of 11 amino acid CDR3 domains in V kappa III-derived immunoglobulin light chains in Kawasaki disease. Scand J Rheumatol. 1997; 26:350–354.


41. Lee HH, Park IH, Shin JS, Kim DS. Immunoglobulin V(H) chain gene analysis of peripheral blood IgM-producing B cells in patients with Kawasaki disease. Yonsei Med J. 2009; 50:493–504.


42. Laird RM, Laky K, Hayes SM. Unexpected role for the B cell-specific Src family kinase B lymphoid kinase in the development of IL-17-producing γδ T cells. J Immunol. 2010; 185:6518–6527.


43. Sohn MH, Noh SY, Chang W, Shin KM, Kim DS. Circulating interleukin 17 is increased in the acute stage of Kawasaki disease. Scand J Rheumatol. 2003; 32:364–366.


44. Jia S, Li C, Wang G, Yang J, Zu Y. The T helper type 17/regulatory T cell imbalance in patients with acute Kawasaki disease. Clin Exp Immunol. 2010; 162:131–137.


45. Lee YC, Kuo HC, Chang JS, Chang LY, Huang LM, Chen MR, et al. Two new susceptibility loci for Kawasaki disease identified through genome-wide association analysis. Nat Genet. 2012; 44:522–525.


46. Wang CL, Wu YT, Liu CA, Lin MW, Lee CJ, Huang LT, et al. Expression of CD40 ligand on CD4+ T-cells and platelets correlated to the coronary artery lesion and disease progress in Kawasaki disease. Pediatrics. 2003; 111:E140–E147.


48. Kim DS, Lee KY. Serum soluble E-selectin levels in Kawasaki disease. Scand J Rheumatol. 1994; 23:283–286.


49. Brown TJ, Crawford SE, Cornwall ML, Garcia F, Shulman ST, Rowley AH. CD8 T lymphocytes and macrophages infiltrate coronary artery aneurysms in acute Kawasaki disease. J Infect Dis. 2001; 184:940–943.


50. Rowley AH, Shulman ST, Spike BT, Mask CA, Baker SC. Oligoclonal IgA response in the vascular wall in acute Kawasaki disease. J Immunol. 2001; 166:1334–1343.


51. Rowley AH, Shulman ST, Mask CA, Finn LS, Terai M, Baker SC, et al. IgA plasma cell infiltration of proximal respiratory tract, pancreas, kidney, and coronary artery in acute Kawasaki disease. J Infect Dis. 2000; 182:1183–1191.


52. Rodó X, Curcoll R, Robinson M, Ballester J, Burns JC, Cayan DR, et al. Tropospheric winds from northeastern China carry the etiologic agent of Kawasaki disease from its source to Japan. Proc Natl Acad Sci U S A. 2014; 111:7952–7957.


53. Gavin PJ, Crawford SE, Shulman ST, Garcia FL, Rowley AH. Systemic arterial expression of matrix metalloproteinases 2 and 9 in acute Kawasaki disease. Arterioscler Thromb Vasc Biol. 2003; 23:576–581.


54. Takeshita S, Tokutomi T, Kawase H, Nakatani K, Tsujimoto H, Kawamura Y, et al. Elevated serum levels of matrix metalloproteinase-9 (MMP-9) in Kawasaki disease. Clin Exp Immunol. 2001; 125:340–344.


55. Maeno N, Takei S, Masuda K, Akaike H, Matsuo K, Kitajima I, et al. Increased serum levels of vascular endothelial growth factor in Kawasaki disease. Pediatr Res. 1998; 44:596–599.


56. Wong M, Silverman ED, Fish EN. Evidence for RANTES, monocyte chemotactic protein-1, and macrophage inflammatory protein-1 beta expression in Kawasaki disease. J Rheumatol. 1997; 24:1179–1185.
57. Matsubara T, Furukawa S, Yabuta K. Serum levels of tumor necrosis factor, interleukin 2 receptor, and interferon-gamma in Kawasaki disease involved coronary-artery lesions. Clin Immunol Immunopathol. 1990; 56:29–36.


58. Lin CY, Lin CC, Hwang B, Chiang B. Serial changes of serum interleukin-6, interleukin-8, and tumor necrosis factor alpha among patients with Kawasaki disease. J Pediatr. 1992; 121:924–926.


59. Kim DS, Lee HK, Noh GW, Lee SI, Lee KY. Increased serum interleukin-10 level in Kawasaki disease. Yonsei Med J. 1996; 37:125–130.


60. Kim KC, Kim DS. The soluble interleukin 2 receptor levels in Kawasaki disease. J Korean Pediatr Soc. 1992; 35:1657–1666.
61. Hwang DH, Han JW, Choi KM, Shin KM, Kim DS. Expression of Toll-like Receptor-2 on the Peripheral Blood Monocytes in Kawasaki Disease Patients. Korean J Pediatr. 2005; 48:315–320.
62. Ikeda K, Yamaguchi K, Tanaka T, Mizuno Y, Hijikata A, Ohara O, et al. Unique activation status of peripheral blood mononuclear cells at acute phase of Kawasaki disease. Clin Exp Immunol. 2010; 160:246–255.


63. Nishio H, Kanno S, Onoyama S, Ikeda K, Tanaka T, Kusuhara K, et al. Nod1 ligands induce site-specific vascular inflammation. Arterioscler Thromb Vasc Biol. 2011; 31:1093–1099.


64. Kusuda T, Nakashima Y, Murata K, Kanno S, Nishio H, Saito M, et al. Kawasaki disease-specific molecules in the sera are linked to microbe-associated molecular patterns in the biofilms. PLoS One. 2014; 9:e113054.


65. Hara T. [Kawasaki disease and innate immunity]. Nihon Rinsho. 2014; 72:1542–1547.
66. Murata H. Experimental candida-induced arteritis in mice. Relation to arteritis in the mucocutaneous lymph node syndrome. Microbiol Immunol. 1979; 23:825–831.
67. Chun JK, Jeon BY, Kang DW, Kim DS. Bacille Calmette Guérin (BCG) can induce Kawasaki disease-like features in programmed death-1 (PD-1) gene knockout mice. Clin Exp Rheumatol. 2011; 29:743–750.
68. Lehman TJ, Walker SM, Mahnovski V, McCurdy D. Coronary arteritis in mice following the systemic injection of group B Lactobacillus casei cell walls in aqueous suspension. Arthritis Rheum. 1985; 28:652–659.


69. Felsburg PJ, HogenEsch H, Somberg RL, Snyder PW, Glickman LT. Immunologic abnormalities in canine juvenile polyarteritis syndrome: a naturally occurring animal model of Kawasaki disease. Clin Immunol Immunopathol. 1992; 65:110–118.


70. Onouchi Z, Ikuta K, Nagamatsu K, Tamiya H, Sakakibara Y, Ando M. Coronary artery aneurysms develop in weanling rabbits with serum sickness but not in mature rabbits. An experimental model for Kawasaki disease in humans. Angiology. 1995; 46:679–687.


71. Duong TT, Silverman ED, Bissessar MV, Yeung RS. Superantigenic activity is responsible for induction of coronary arteritis in mice: an animal model of Kawasaki disease. Int Immunol. 2003; 15:79–89.


72. Takahashi K, Oharaseki T, Wakayama M, Yokouchi Y, Naoe S, Murata H. Histopathological features of murine systemic vasculitis caused by Candida albicans extract--an animal model of Kawasaki disease. Inflamm Res. 2004; 53:72–77.


73. Ohno N. Murine model of Kawasaki disease induced by mannoprotein-beta-glucan complex, CAWS, obtained from Candida albicans. Jpn J Infect Dis. 2004; 57:S9–S10.
74. Nakamura T, Yamamura J, Sato H, Kakinuma H, Takahashi H. Vasculitis induced by immunization with Bacillus Calmette-Guérin followed by atypical mycobacterium antigen: a new mouse model for Kawasaki disease. FEMS Immunol Med Microbiol. 2007; 49:391–397.


75. Schulte DJ, Yilmaz A, Shimada K, Fishbein MC, Lowe EL, Chen S, et al. Involvement of innate and adaptive immunity in a murine model of coronary arteritis mimicking Kawasaki disease. J Immunol. 2009; 183:5311–5318.


76. Takahashi K, Oharaseki T, Yokouchi Y. Update on etio and immunopathogenesis of Kawasaki disease. Curr Opin Rheumatol. 2014; 26:31–36.


77. Motomura Y, Kanno S, Asano K, Tanaka M, Hasegawa Y, Katagiri H, et al. Identification of Pathogenic Cardiac CD11c+ Macrophages in Nod1-Mediated Acute Coronary Arteritis. Arterioscler Thromb Vasc Biol. 2015; 35:1423–1433.


78. Kato H, Sugimura T, Akagi T, Sato N, Hashino K, Maeno Y, et al. Long-term consequences of Kawasaki disease. A 10- to 21-year follow-up study of 594 patients. Circulation. 1996; 94:1379–1385.
79. Noto N, Okada T, Yamasuge M, Taniguchi K, Karasawa K, Ayusawa M, et al. Noninvasive assessment of the early progression of atherosclerosis in adolescents with Kawasaki disease and coronary artery lesions. Pediatrics. 2001; 107:1095–1099.


80. Cheung YF, Wong SJ, Ho MH. Relationship between carotid intima-media thickness and arterial stiffness in children after Kawasaki disease. Arch Dis Child. 2007; 92:43–47.


81. Nakamura Y, Yashiro M, Uehara R, Oki I, Kayaba K, Yanagawa H. Increasing incidence of Kawasaki disease in Japan: nationwide survey. Pediatr Int. 2008; 50:287–290.

