Abstract
Purpose
Neural stem cells (NSCs) effectively reverse some severe central nervous system (CNS) disorders, due to their ability to differentiate into neurons. Agmatine, a biogenic amine, has cellular protective effects and contributes to cellular proliferation and differentiation in the CNS. Recent studies have elucidated the function of microRNA let-7a (let-7a) as a regulator of cell differentiation with roles in regulating genes associated with CNS neurogenesis.
Materials and Methods
This study aimed to investigate whether agmatine modulates the expression of crucial regulators of NSC differentiation including DCX, TLX, c-Myc, and ERK by controlling let-7a expression.
Neural stem cells (NSCs) maintain multipotency and are capable of self-renewal;1 and it has been demonstrated that dividing NSCs in the subventricular and subgranular zone2 can become neurons.3 NSCs have been studied extensively as promising novel treatments for central nervous system (CNS) disorders.4 Several studies have demonstrated that proliferation of endogenous NSCs is enhanced after brain injury, as is migration of neural progenitor cells to the brain lesion.5 In addition, NSCs migrate toward injured brain regions, differentiate into neurons, and then facilitate injury healing.6 Thus, NSC self-renewal and proliferation are important characteristics for the enhanced therapeutic efficiency of NSCs in the brain.7
Agmatine is an endogenous amine produced by decarbox-ylation of L-arginine by arginine decarboxylase present in glia and neurons,8 and it has been reported to affect various cellular processes, such as antioxidative pathways,9 and also has a role in CNS injuries including neurotrauma and animal models of ischemia.1011 Moreover, agmatine initiates early neurogenesis and cell proliferation.12
MicroRNAs can regulate target mRNAs via translational inhibition or mRNA degradation.13 Recent studies have focused on the mechanisms of microRNA action on NSCs, in order to discover potential treatments for CNS diseases.14 MicroRNA let-7 (let-7) is a family of microRNAs present in multiple genomic locations,15 and consists of 9 members.16 let-7 targets cyclin D1, and its overexpression promotes cell cycle exit and differentiation.17 Furthermore, let-7 suppresses differentiation of human embryonic stem cell derived neural progenitor cells,18 and influences self-renewal of NSCs.19 Recent studies have suggested a function of let-7 in cell fate mechanisms in neurons.2021 In addition, overexpression of let-7a, one of the members of let-7 family, influences NSC proliferation and differentiation.2223 However, the mechanisms of action of the let-7 family on NSC proliferation or neuronal differentiation are poorly understood and are debatable. Agmatine has been shown to block neuronal nitric oxide synthase,1024 inducible nitric oxide synthase25 and N-methyl-D-aspartate receptor channels.26 Furthermore, the nitric oxide synthase inhibitor asymmetric di-methylarginine increased the levels of microRNAs such as microRNA-21.27 Taken together, therefore, it is quite possible that there exists a relationship between agmatine and let-7a in the regulation of NSC differentiation. Accordingly, the aim of the present study was to investigate the effects of agmatine on NSCs by controlling let-7a levels.
Cortical NSCs were obtained from pregnant imprinting control region mice (E13.5). The cortices were dissected and washed 1–2 times with Hank's Balanced Salt Solution (HBSS; HyClone Laboratories, South Logan, UT, USA). To each piece of washed tissue, 5 mL of HBSS were added, and the tissue was dissociated by pipetting up and down. Tissues were triturated by repeated passages through a fire-polished constricted Pasteur pipette. Dissociated tissues were allowed to settle for 3 min. Supernatants were transferred to a fresh tube, and were centrifuged at 1200 g for 3 min. Pellets were resuspended in NSC basal media with a proliferation supplement (Stem Cell Technologies, Vancouver, Canada), and 20 ng/mL epidermal growth factor (EGF, Invitrogen, Carlsbad, CA, USA). NSCs were plated on poly-D-ornithine (Sigma-Aldrich, St. Louis, MO, USA) treated dishes at a density of 2.5×104 cells/mL. Cultures were maintained in a humidified atmosphere of 95% air and 5% CO2 at 37℃. Culture medium was replaced every 3 days. NSCs were used for experiments after 2–3 passages.
let-7a mimics, inhibitors, and siTLX were purchased from Ambion (Austin, TX, USA); they were mmu-let-7a-5p (let-7a mimic) (cat. #4464066) and let-7a inhibitor (cat. #4464066; assay ID MH10050). For RNA duplex transfection, 20 nM solutions in Opti-MEM (Sigma, St. Louis, MO, USA) were combined with Lipofectamine 2000 (Invitrogen, Carlsbad, CA, USA). After 15 min, the mixtures were added to the cells incubated for 72 h.
Images of neurosphere cultures were taken using an inverted microscope (Olympus microscopy BH3, Tokyo, Japan). The magnification (10×) ensured coverage of a significant area of each well of the 24-well plates. Image analysis software (Image J) was used to evaluate the size of neurospheres.
To examine the expression of TLX, DCX, and c-Myc in NSCs, RT-PCR was performed using specific primers. Briefly, the cell pellets were lysed with TRIzol reagent (Invitrogen, Carlsbad, CA, USA). PCR was conducted by the following manual's conditions, and the following primers (5' to 3'): TLX, forward (F): GGC TCT CTA CTT CCG TGG ACA, reverse (R): GTC AGT ATT CAT GCC AGA TAC AGC CAG TG; DCX, (F): AAT CCC AAC TGG TCT GTC AAC, (R): GTT TCC CTT CAT GAC TCG GCA; c-Myc, (F): TCA AGA GGC GAA CAC ACA AC, (R): GGC CTT TTC ATT GTT TTC CA, and GAPDH, (F): ACA GTC CAT GCC ATC ACT GCC, (R): GCC TGC TTC ACC ACC TTC TTG. GAPDH was used as an internal control.
For quantitative analysis of let-7a, reverse transcription was first performed using the TaqMan Micro RNA Reverse Transcription Kit (Takara, Otsu, Shiga, Japan) with total RNA samples of 10 ng. PCR was then performed according to the manufacturer's instructions using the TaqMan Universal PCR Master Mix, No Amp Erase UNG (Applied Biosystems, Foster City, CA, USA); PCR amplification was carried out in an ABI 7500 Real-Time PCR cycler (Bio-Rad, Philadelphia, PA, USA). We used the following primers (5' to 3'): let-7a, (F): GCG CCT GAG GTA GTA GGT TG, (R): CAG TGC AGG GTC CGA GGT; and U6, (F): CTC GCT TCG GCA GCA CAT ATA CT, (R): ACG CTT CAC GAA TTT GCG TGT C. The let-7a levels were normalized to the internal control U6.
The NSCs were homogenized in lysis buffer and centrifuged (12000 g at 4℃) for 15 min. Equal amounts of protein (30 µg) from the supernatants were separated on a 10% acrylamide gel, and the proteins were electrotransferred onto nitrocellulose membranes. After blocking, the membranes were incubated with primary antibody at 4℃. The primary antibodies included anti-DCX (1:2000, Millipore, Billerica, MA, USA), anti-NeuN (1:1000, Santa Cruz Biotechnology, Santa Cruz, CA, USA), and anti-β-actin (1:2000, Santa Cruz Biotechnology) antibodies. The membranes were then incubated with secondary antibodies. The blots were rinsed, and protein bands were visualized using an enhanced chemiluminescence detection system (Amersham, Pittsburgh, PA, USA).
The NSCs were incubated with primary antibody overnight at 4℃ (goat anti-DCX; 1:200; Millipore). Cell samples were then incubated with a rhodamine-conjugated donkey anti-goat antibody (1:200, Jackson ImmunoResearch, West Grove, PA, USA) for 2 h at room temperature (RT). The NSCs were washed 3 times again, for 3 min each with PBS. The cells were then counterstained with 1 µg/mL 4', 6-diamidino-2-phenylindole (DAPI; 1:100; Invitrogen, Carlsbad, CA, USA) for 10 min at RT and were photographed using a confocal microscope (Carl Zeiss, Thornwood, NY, USA).
All calculations were conducted using SPSS 18.0 software (IBM Corp., Armonk, NY, USA). Data are expressed as mean±SEM. Significance of intergroup differences was determined by one-way analysis of variance followed by Bonferroni post hoc multiple-comparison tests. Each experiment included at least three repeats per condition. Differences with a p value less than 0.05 were considered statistically significant.
We conducted western blot analyses of the expression of neuronal markers (NeuN28 and DCX29) in NSCs after agmatine treatment (Fig. 1). Treatment with agmatine (100, 200 µM) increased expression of NeuN (Fig. 1A) and DCX (Fig. 1B), while 50 µM agmatine treatment showed a small decrease of DCX protein level (Fig. 1B). Specifically, 100 µM agmatine treatment visibly increased the protein levels of NeuN (Fig. 1A) and DCX (Fig. 1B). On the basis of these findings, we chose 100 µM agmatine for the following experiments with an assumption that 100 µM ag-matine would promote neuronal differentiation in NSCs.
In NSCs, we confirmed let-7a expression by a TaqMan assay (Fig. 2). Agmatine treatment decreased let-7a expression in NSCs compared with the normal control group. Although a few differences were observed between agmatine treatment and agmatine treatment along with let-7a overexpression (the same with let-7a mimic treatment), we found that agmatine dramatically reduced let-7a levels in spite of let-7a mimic treatment (Fig. 2), indicating that agmatine negatively regulates let-7a expression in NSCs.
Immunochemical images of DCX indicated that let-7a overexpression inhibited the expression of DCX (Fig. 3A). Agmatine treatment increased the number of DCX-positive cells in both the let-7a overexpression state (let-7a mimic treatment) and the normal state (Fig. 3A). Fig. 3B demonstrates that let-7a overexpression (let-7a mimic treatment) in NSCs decreased DCX mRNA levels compared with the normal control group (Fig. 3B). Agmatine treatment group shows DCX mRNA induction that occurred with let-7a overexpression (Fig. 3B). Our results suggest that DCX, a marker for immature neurons, was downregulated under let-7a overexpression, whereas DCX expression was increased under let-7a overexpression in agmatine treatment group (Fig. 3).
TLX mRNA levels are associated with self-renewal and proliferation30 of NSCs. We found that let-7a overexpression (let-7a mimic treatment) increased TLX expression (Fig. 3C). Although the expression of TLX was increased by agmatine in NSCs, it was noticeably reduced in agmatine treatment group under let-7a mimic treatment in comparison with the let-7a overexpression group (Fig. 3C). In addition, we observed a slight increase in TLX mRNA expression after the let-7a inhibitor treatment, in comparison with the let-7a overexpression group under agmatine treatment conditions (Fig. 3C). In the TLX knockdown group, let-7a expression increased slightly, whereas the agmatine co-treatment group showed a marked decrease in let-7a expression (Fig. 3D).
Expression of c-Myc mRNA in NSCs was measured using real-time quantitative RT-PCR (Fig. 4A), since c-Myc plays a crucial role in proliferation and self-renewal31 of NSCs. We observed increased expression of c-Myc in the let-7a overexpression group (let-7a mimic treatment) (Fig. 4A). After agmatine treatment, c-Myc mRNA expression was slightly increased. However, the let-7a overexpression with agmatine treatment reduced the expression of c-Myc (Fig. 4A).
In order to determine signaling pathway affected by agmatine and let-7a, we measured ERK phosphorylation which is related to NSC proliferation (Fig. 4B).12 Activation of ERK, as indicated by the level of phosphorylated ERK protein, was reduced in NSCs after let-7a mimic treatment. Agmatine increased the activation of ERK in NSCs, whereas the expression of ERK was decreased by let-7a overexpression (Fig. 4B). Given the fact that ERK activation is important for proliferation and self-renewal of NSCs,32 our results indicated that agmatine may reverse the decrease of ERK phosphorylation, induced by let-7a.
Understanding of NSC proliferation, self-renewal, and differentiation is necessary to enhance the therapeutic efficacy of NSCs.33 Agmatine has multiple roles in several biological processes.3435 In the present study, we found several lines of evidence indicating that agmatine can enhance NSC differentiation into neurons by modulating expression of let-7a. 100 µM agmatine was found to promote differentiation by upregulation of NeuN28 and DCX.29 Since let-7a was reduced in NSCs when treated with agmatine, our results suggest that agmatine may negatively regulate let-7a in NSCs. DCX is regarded as a marker of neurogenesis,36 the findings therefore suggest that agmatine promotes NSC differentiation into neurons: it is quite possible that agmatine may boost neuronal differentiation by inhibiting let-7a expression.
Previous studies have demonstrated that underexpression of let-7a attenuates the proliferation and self-renewal of NSCs.18 TLX is necessary for maintaining the proliferative potential of NSCs and neurogenesis.37 In the present study, we suggest that agmatine may enhance neurogenesis by negatively regulating expression of let-7a and TLX, and it may inhibit NSC proliferation by attenuating the increased TLX level in let-7a overexpression conditions.38
c-Myc regulates the genes involved in self-renewal, and proliferation39 of NSCs, and the let-7 family is known to regulate cell proliferation by targeting c-Myc.40 In our present study, overexpression of let-7a upregulated the expression of c-Myc, which is known to sustain the stemness of stem cells targeted by the let-7 family.41 These findings imply that agmatine may reduce c-Myc via controlling let-7a, thereby influencing self-renewal and proliferation of NSCs.
It has been demonstrated that agmatine regulates neuroprotection and increases neuronal differentiation in NSCs by activating ERK1/2.12 In our present study, we demonstrated that let-7a overexpression in NSCs decreases ERK phosphorylation, thus indicating that agmatine promotes ERK activation by negatively regulating let-7a, which is involved in neuronal differentiation and cell survival of NSCs.
In conclusion, we found four main findings in the present study. 1) Agmatine increased the expression of DCX and NeuN in NSCs. 2) Agmatine decreased the expression of let-7a in NSCs. 3) Agmatine attenuated the let-7a-induced upregulation of TLX and c-Myc. 4) Agmatine ameliorated the let-7a-induced ERK inactivation in NSCs. Therefore, we suggest that agmatine manipulates the mechanism linking let-7a, and induce neuronal differentiation for treatment of CNS disorders.
Figures and Tables
Fig. 1
Alterations in NeuN and DCX protein levels in NSCs by agmatine treatment. (A) The protein levels of NeuN increased in the agmatine treatment group. In particular, the 100 µM agmatine treatment significantly increased NeuN protein levels in NSCs. (B) DCX protein levels increased in the agmatine treatment group. Similar to NeuN, 100 µM agmatine significantly increased DCX protein levels in NSCs. β-actin was used as an internal control. Data are expressed as mean±SEM. *p<0.05, †p<0.001 compared to agmatine 0 uM group. Agm, agmatine treatment; NSCs, neural stem cells.
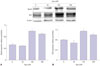
Fig. 2
Effects of alterations of let-7a levels in neural stem cells. A TaqMan assay was performed to check let-7a level. The level of let-7a decreased in the agmatine group compared with the let-7a mimic group. U6 was used as an internal control. Data are expressed as mean±SEM. *p<0.05, †p<0.001 compared to normal control group. let-7a mimic, let-7a mimic treatment/let-7a overexpression group; let-7a inhibitor, let-7a inhibitor treatment/let-7a suppression group; Agm, 100 µM agmatine treatment group; Veh, Lipofectamine treatment group.
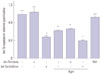
Fig. 3
The expression of DCX and TLX mRNA levels in neural stem cells. (A) Immunochemical images indicated that DCX-positive cells decreased in the let-7a mimic group. Scale bar: 200 µm. NC: the normal control group, 4', 6-diamidino-2-phenylindole (DAPI): blue, DCX: red. (B) Under agmatine treatment, the let-7a suppression group (let-7a inhibitor group) expressed lower DCX mRNA levels. GAPDH was used as an internal control. (C) The let-7a mimic group with agmatine had decreased TLX mRNA expression compared to the let-7a mimic group. GAPDH was used as an internal control. (D) Inhibition of TLX expression by agmatine decreased let-7a levels. U6 was used as an internal control. Data are expressed as mean±SEM. *p<0.05, †p<0.001 compared to normal control group, ‡p<0.001 compared to let-7a mimic group. let-7a mimic, let-7a mimic treatment/let-7a overexpression group; let-7a inhibitor, let-7a inhibitor treatment/let-7a suppression group; Normal, normal control group/no treatment; Agm, 100 µM agmatine treatment group; siTLX, TLX silencing group; siTLX+Agm, TLX silencing with agmatine treatment group.
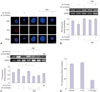
Fig. 4
Assessment of c-Myc mRNA and ERK phosphorylation in neural stem cells. (A) c-Myc mRNA expression was reduced in the let-7a mimic treatment with agmatine group in comparison with let-7a mimic group. β-actin was used as an internal control. (B) ERK phosphorylation significantly increased in the let-7a mimic treatment with agmatine group compared to let-7a mimic group. GAPDH was used as an internal control. Data are expressed as mean±SEM. *p<0.05, †p<0.001 compared to normal control group, ‡p<0.05 compared to let-7a mimic group. let-7a mimic, let-7a mimic treatment/let-7a overexpression group; let-7a inhibitor, let-7a inhibitor treatment/let-7a suppression group; Agm, 100 µM agmatine treatment group; Veh, Lipofectamine treatment group; p-ERK, ERK phosphorylation.
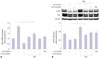
ACKNOWLEDGEMENTS
This research was supported by the Basic Science Research Program through the National Research Foundation of Korea (NRF) funded by the Ministry of Education, Science, and Technology (NRF-2014R1A2A2A01006556).
References
2. Ming GL, Song H. Adult neurogenesis in the mammalian brain: significant answers and significant questions. Neuron. 2011; 70:687–702.


3. Kazanis I, Lathia J, Moss L, ffrench-Constant C. The neural stem cell microenvironment. Cambridge: StemBook;2008.
4. Joo KM, Kang BG, Yeon JY, Cho YJ, An JY, Song HS, et al. Experimental and clinical factors influencing long-term stable in vitro expansion of multipotent neural cells from human adult temporal lobes. Exp Neurol. 2013; 240:168–177.


6. Kallur T, Darsalia V, Lindvall O, Kokaia Z. Human fetal cortical and striatal neural stem cells generate region-specific neurons in vitro and differentiate extensively to neurons after intrastriatal transplantation in neonatal rats. J Neurosci Res. 2006; 84:1630–1644.


8. Bokara KK, Kwon KH, Nho Y, Lee WT, Park KA, Lee JE. Retroviral expression of arginine decarboxylase attenuates oxidative burden in mouse cortical neural stem cells. Stem Cells Dev. 2011; 20:527–537.


9. Reis DJ, Regunathan S. Is agmatine a novel neurotransmitter in brain? Trends Pharmacol Sci. 2000; 21:187–193.


10. Kim JH, Yenari MA, Giffard RG, Cho SW, Park KA, Lee JE. Agmatine reduces infarct area in a mouse model of transient focal cerebral ischemia and protects cultured neurons from ischemia-like injury. Exp Neurol. 2004; 189:122–130.


11. Mun CH, Lee WT, Park KA, Lee JE. Regulation of endothelial nitric oxide synthase by agmatine after transient global cerebral ischemia in rat brain. Anat Cell Biol. 2010; 43:230–240.


12. Song HW, Kumar BK, Kim SH, Jeon YH, Lee YA, Lee WT, et al. Agmatine enhances neurogenesis by increasing ERK1/2 expression, and suppresses astrogenesis by decreasing BMP 2,4 and SMAD 1,5,8 expression in subventricular zone neural stem cells. Life Sci. 2011; 89:439–449.


13. Navarro A, Monzo M. MicroRNAs in human embryonic and cancer stem cells. Yonsei Med J. 2010; 51:622–632.


14. Cheng LC, Pastrana E, Tavazoie M, Doetsch F. miR-124 regulates adult neurogenesis in the subventricular zone stem cell niche. Nat Neurosci. 2009; 12:399–408.


16. Pasquinelli AE, Reinhart BJ, Slack F, Martindale MQ, Kuroda MI, Maller B, et al. Conservation of the sequence and temporal expression of let-7 heterochronic regulatory RNA. Nature. 2000; 408:86–89.


17. Zhao C, Sun G, Li S, Lang MF, Yang S, Li W, et al. MicroRNA let-7b regulates neural stem cell proliferation and differentiation by targeting nuclear receptor TLX signaling. Proc Natl Acad Sci U S A. 2010; 107:1876–1881.


18. Cimadamore F, Amador-Arjona A, Chen C, Huang CT, Terskikh AV. SOX2-LIN28/let-7 pathway regulates proliferation and neurogenesis in neural precursors. Proc Natl Acad Sci U S A. 2013; 110:E3017–E3026.


19. Zhao C, Sun G, Ye P, Li S, Shi Y. MicroRNA let-7d regulates the TLX/microRNA-9 cascade to control neural cell fate and neurogenesis. Sci Rep. 2013; 3:1329.


20. Wu YC, Chen CH, Mercer A, Sokol NS. Let-7-complex microRNAs regulate the temporal identity of Drosophila mushroom body neurons via chinmo. Dev Cell. 2012; 23:202–209.


21. Kucherenko MM, Barth J, Fiala A, Shcherbata HR. Steroid-induced microRNA let-7 acts as a spatio-temporal code for neuronal cell fate in the developing Drosophila brain. EMBO J. 2012; 31:4511–4523.


22. Schwamborn JC, Berezikov E, Knoblich JA. The TRIM-NHL protein TRIM32 activates microRNAs and prevents self-renewal in mouse neural progenitors. Cell. 2009; 136:913–925.


24. Hong S, Son MR, Yun K, Lee WT, Park KA, Lee JE. Retroviral expression of human arginine decarboxylase reduces oxidative stress injury in mouse cortical astrocytes. BMC Neurosci. 2014; 15:99.


25. Halaris A, Plietz J. Agmatine : metabolic pathway and spectrum of activity in brain. CNS Drugs. 2007; 21:885–900.
26. Yang XC, Reis DJ. Agmatine selectively blocks the N-methyl-D-aspartate subclass of glutamate receptor channels in rat hippocampal neurons. J Pharmacol Exp Ther. 1999; 288:544–549.
27. Iannone L, Zhao L, Dubois O, Duluc L, Rhodes CJ, Wharton J, et al. miR-21/DDAH1 pathway regulates pulmonary vascular responses to hypoxia. Biochem J. 2014; 462:103–112.
28. Mullen RJ, Buck CR, Smith AM. NeuN, a neuronal specific nuclear protein in vertebrates. Development. 1992; 116:201–211.


29. Fu X, Brown KJ, Yap CC, Winckler B, Jaiswal JK, Liu JS. Doublecortin (Dcx) family proteins regulate filamentous actin structure in developing neurons. J Neurosci. 2013; 33:709–721.


30. Shi Y, Sun G, Zhao C, Stewart R. Neural stem cell self-renewal. Crit Rev Oncol Hematol. 2008; 65:43–53.


31. Wang J, Wang H, Li Z, Wu Q, Lathia JD, McLendon RE, et al. c-Myc is required for maintenance of glioma cancer stem cells. PLoS One. 2008; 3:e3769.


32. Tocharus C, Puriboriboon Y, Junmanee T, Tocharus J, Ekthuwapranee K, Govitrapong P. Melatonin enhances adult rat hippocampal progenitor cell proliferation via ERK signaling pathway through melatonin receptor. Neuroscience. 2014; 275:314–321.


33. Connor B, Gordon RJ, Jones KS, Maucksch C. Deviating from the well travelled path: precursor cell migration in the pathological adult mammalian brain. J Cell Biochem. 2011; 112:1467–1474.


34. El-Agamy DS, Makled MN, Gamil NM. Protective effects of agmatine against D-galactosamine and lipopolysaccharide-induced fulminant hepatic failure in mice. Inflammopharmacology. 2014; 22:187–194.


35. Park YM, Han SH, Seo SK, Park KA, Lee WT, Lee JE. Restorative benefits of transplanting human mesenchymal stromal cells overexpressing arginine decarboxylase genes after spinal cord injury. Cytotherapy. 2015; 17:25–37.


36. Couillard-Despres S, Winner B, Schaubeck S, Aigner R, Vroemen M, Weidner N, et al. Doublecortin expression levels in adult brain reflect neurogenesis. Eur J Neurosci. 2005; 21:1–14.


37. Liu HK, Belz T, Bock D, Takacs A, Wu H, Lichter P, et al. The nuclear receptor tailless is required for neurogenesis in the adult subventricular zone. Genes Dev. 2008; 22:2473–2478.


38. Wang T, Ren X, Xiong J, Zhang L, Qu J, Xu W. Tailless-like (TLX) protein promotes neuronal differentiation of dermal multipotent stem cells and benefits spinal cord injury in rats. Cell Mol Neurobiol. 2011; 31:479–487.


39. Kerosuo L, Piltti K, Fox H, Angers-Loustau A, Häyry V, Eilers M, et al. Myc increases self-renewal in neural progenitor cells through Miz-1. J Cell Sci. 2008; 121(Pt 23):3941–3950.

