Abstract
Purpose
Peroxynitrite plays a critical role in vascular pathophysiology by increasing arginase activity and decreasing endothelial nitric oxide synthase (eNOS) activity. Therefore, the aims of this study were to investigate whether arginase inhibition and L-arginine supplement could restore peroxynitrite-induced endothelial dysfunction and determine the involved mechanism.
Materials and Methods
Human umbilical vein endothelial cells (HUVECs) were treated with SIN-1, a peroxynitrite generator, and arginase activity, nitrite/nitrate production, and expression levels of proteins were measured. eNOS activation was evaluated via Western blot and dimer blot analysis. We also tested nitric oxide (NO) and reactive oxygen species (ROS) production and performed a vascular tension assay.
Results
SIN-1 treatment increased arginase activity in a time- and dose-dependent manner and reciprocally decreased nitrite/nitrate production that was prevented by peroxynitrite scavenger in HUVECs. Furthermore, SIN-1 induced an increase in the expression level of arginase I and II, though not in eNOS protein. The decreased eNOS phosphorylation at Ser1177 and the increased at Thr495 by SIN-1 were restored with arginase inhibitor and L-arginine. The changed eNOS phosphorylation was consistent in the stability of eNOS dimers. SIN-1 decreased NO production and increased ROS generation in the aortic endothelium, all of which was reversed by arginase inhibitor or L-arginine. NG-Nitro-L-arginine methyl ester (L-NAME) prevented SIN-1-induced ROS generation. In the vascular tension assay, SIN-1 enhanced vasoconstrictor responses to U46619 and attenuated vasorelaxant responses to acetylcholine that were reversed by arginase inhibition.
The endothelium plays a pivotal role in the maintenance of vascular homeostasis by modulating vascular tone, leukocyte adhesion, platelet aggregation, smooth muscle cell proliferation, and migration. Recent discoveries have revealed that endothelial nitric oxide (NO) is an important vasoprotective molecule contributing to endothelial functions, and impaired NO signaling associated with endothelial dysfunction is now regarded as an early marker of cardiovascular diseases.
Endothelial arginase inhibits endothelial NO synthase (eNOS) activity by depleting its common substrate (L-arginine), which lowers NO bioavailability and leads to vascular diseases in aging, hypertension, and atherosclerosis.1234 In atherogenic-prone apolipoprotein E-null (ApoE-/-) mice, arginase inhibition restores NO and reactive oxygen species (ROS) balance, endothelial function, and arterial stiffness to the wild-type phenotype.5 In the aging cardiovascular system, inhibition of S-nitrosylation-dependent arginase I activation restores depressed endothelial function.6 Furthermore, we demonstrated that in both pathophysiological and normal physiological vasculature, arginase inhibition induced eNOS activation through phosphorylation at Ser1177.7
Redox-imbalance may result in the generation of peroxynitrite, which is associated with cardiovascular diseases as it initiates the modification of DNA, carbohydrates, and proteins. Several protein molecules including eNOS and arginase are affected by post-translational modifications such as peroxynitrite-induced S-nitrosylation, which is involved in the regulation of enzyme activity. In endothelial cells, peroxynitrite induces the S-nitrosylation of eNOS and the oxidation of the essential cofactor for NOS, (6R-)5,6,7,8-tetrabiopterin (BH4), resulting in impaired endothelial function through NOS uncoupling.8 In addition, peroxynitrite also reduces intracellular L-arginine concentration by decreasing endothelial L-arginine influx and increasing L-arginine efflux via nitrosylation of cationic amino acid transporter. The decline in L-arginine concentration results in less NO production.9
Although a peroxynitrite generator, 5-amino-3-morpholinyl-1,2,3-oxadiazolium chloride (SIN-1), induces the upregulation of arginase activity and expression contributing to endothelial dysfunction,10 it is not completely known whether arginase inhibition and L-arginine supplement could restore peroxynitrite-dependent endothelial dysfunction and if so, which mechanism is involved. Therefore, with the hypothesis that peroxynitrite-dependent endothelial dysfunction may be induced by L-arginine depletion, which was derived from arginase activation and further provoked eNOS uncoupling, we tested whether increased L-arginine bioavailability to eNOS via arginase inhibition and L-arginine supplement could restore eNOS activation and depressed endothelial function. In our study, we demonstrated that stimulation of human umbilical vein endothelial cells (HUVECs) with peroxynitrite increased arginase activity with an effect on expression levels of arginase I/II. Increased arginase activity was associated with eNOS uncoupling. Arginase inhibition and L-arginine supplement enhanced the phosphorylation of Ser1177, reduced the phosphorylation of Thr495, induced the augmented stability of eNOS dimers, and reciprocally regulated NO production and ROS generation. Furthermore, impaired vascular reactivity caused by peroxynitrite preincubation was restored by treatment with an arginase inhibitor. These findings suggest a new vasoprotective effect of arginase inhibition during nitroso-redox imbalance.
ABH [2(S)-amino-6-boronohexanoic acid], SIN-1 (Amino-3-morpholinyl-1,2,3-oxadiazolium chloride), ONOO-, and FeTPPS [5,10,15,20-Tetrakis (4-sulfonatophenyl) prophyrinato iron (III)] were purchased from Calbiochem (Darmstadt, Germany). All other chemicals were obtained from Sigma-Aldrich (St. Louis, MO, USA) unless otherwise stated.
HUVECs were purchased from Cascade Biologics (Portland, OR, USA) and maintained in Medium 200 containing low serum growth supplement according to the supplier's protocol.
Arginase activity was measured by determination of urea content using α-isonitrosopropiophenone as described previously.3 Briefly, supernatants of extracted cell lysates were prepared by incubation with lysis buffer (50 mM Tris-HCl, pH7.5, 0.1 mM EDTA, and protease inhibitors) for 30 min at 4℃ followed by centrifugation at 14000× g for 20 min at 4℃. HUVECs were treated with arginase inhibitor (ABH) before SIN-1 stimulation. Assays on aortic vessel samples were performed following homogenization in lysis buffer.
A NO assay kit (Calbiochem) was used to measure nitrite/nitrate (NOx) via the Griess reaction, after conversion of nitrate to nitrite with nitrate reductase. The concentration of NOx from cell lysates was expressed as µmol/g protein.
All experimental procedures using mice were approved by the Institutional Review Board at Kangwon National University. Aortic rings from 10-week-old male wild-type C57BL/6J mice were prepared for arginase activity assays or labeled with dihydroethidine (DHE, 1 µmol/L, 5 min) or 4-amino-5-methylamino-2',7'-difluorescein (DAF, 5 µmol/L, 5 min), fluorescent probes for superoxide and NO, respectively. Images were acquired using an Olympus BX51 epifluorescence microscope. Fluorescent intensity was measured as previously described5 using MetaMorph software (Molecular Devices, Inc., Sunnyvale, CA, USA).
Cells were lysed in SDS-sample buffer (62.5 mMTris, pH 6.8, 2% SDS, and 10% glycerol) and then sonicated for 5 s to reduce sample viscosity. Each sample was resolved by 10% SDS-polyacrylamide gel electrophoresis (SDS-PAGE), transferred to polyvinylidene difluoride (PVDF) membrane (BioRad, Hercules, CA, USA), analyzed with antibodies [eNOS, inducible nitric oxide synthase (iNOS), hArg1, hArg2 antibodies from Santa Cruz Biotechnology and phospho-eNOS Ser1177 and phosphoeNOS Thr495 antibodies from BD Biosciences] according to the suppliers' protocols, and visualized with peroxidase and an enhanced-chemiluminescence system (Thermo Scientific Pierce, Waltham, MA, USA). Normalization was performed using an anti-β-tubulin antibody (BD bioscience, 1:1000). A densitometry analysis of bands was performed using NIH ImageJ. Dimers and monomers of eNOS were separated by low-temperature SDS-PAGE and analyzed as described above.
Male wild-type (WT, C57BL/6J) mice were anesthetized using isoflurane, and the thoracic aortic vessel was rapidly removed. Each aorta was placed on ice-cold oxygenated Krebs-Ringer bicarbonate solution (NaCl 118.3, KCl 4.7, MgSO4 1.2, KH2PO4 1.2, CaCl2 1.6, NaHCO3 25, glucose 11.1 mM) and cleared of connective tissue. Each mouse aorta was cut into 1.5-mm rings and suspended between two wire stirrups (150 µm) in a myograph (Multi myograph system DMT-620) containing 10 mL Krebs-Ringer solution (95% O2-5% CO2, pH 7.4, 37℃). One stirrup was connected to a three-dimensional micromanipulator, and the other stirrup was connected to a force transducer. The rings were passively stretched at 10-minute intervals in increments of 100 mg in order to reach optimal tone (600 mg). After the arterial rings were stretched to their optimal resting tone, the contractile response to 100 mMKCl was determined. The response to a maximal dose of KCl was used to normalize the responses to agonist across vessel rings. A dose response (10-9–10-5 M) to the vasoconstrictor, U46619, was analyzed, followed by a dose response (10-9–10-5 M) to the vasodilator, acetylcholine (Ach), after preconstriction with U46619 (10-8 M). To confirm that the vasorelaxation activity was NO-dependent, the endothelial layer was physically removed by gentle rubbing.
The intracellular concentration of L-arginine was determined via high-performance liquid chromatography (HPLC) using pre-column derivatization with o-phthalaldehyde (OPA) according to a modification of a previously published method.11 L-arginine (100 µmol/L) was added to the cell lysate (0.5 mL) as an internal standard. The samples were extracted on solid-phase extraction cartridges (CBA Bond elute, Varian). Recovery rates were 87.5±3.9%. Eluates were dried over nitrogen and resuspended in double-distilled water for HPLC analysis. HPLC was performed on a computer-controlled Waters chromatography system (M600E) consisting of an automatic injector (M7725i, Waters Co., Victoria, Australia) and a fluorescence detector (FP-1520, Jasco Co., Queensland, Australia) in the Central Laboratory of Kangwon National University. Samples were incubated for exactly 1 minute with OPA reagent (5.4 mg/mL OPA in borate buffer, pH 8.4, containing 0.4% 2-mercaptoethanol) before automatic injection into the HPLC. The OPA derivative of L-arginine was separated on a 150×4.6 mm (3.5 µm) Zorbax Eclipse XDB-C18 column with a fluorescence detector set at Ex 340 nm and Em 450 nm. Samples were eluted from the column with 0.96% citric acid/methanol (70:30, pH 6.8) at a flow rate of 1.5 mL/min.
First, we measured arginase enzyme activity in SIN-1-treated HUVECs. SIN-1 treatment induced a time-dependent increase in arginase activity (Fig. 1A, *p<0.05 vs. untreated), with a maximal effect at 18 hours of 50 µM SIN-1 (127.4±5.0%). In addition, the different concentration of SIN-1 at 18 hours increased arginase activity in a dose-dependent manner (Fig. 1B, *p<0.01 vs. untreated) with a maximal effect at 50 µmol/L of SIN-1 (135.7±3.8%). As reactivities of major products of SIN-1 (NO, superoxide, and peroxynitrite) are relatively high, it is possible that the effect of SIN-1 is highly dependent on time and concentration. Hence, the optimal concentration (50 µmol/L) and treatment time (18 hours) for SIN-1 were used in all subsequent experiments unless otherwise stated.
As SIN-1 releases equimolar NO and superoxide, which react to form peroxynitrite,12 we next tested whether the effect of SIN-1 is dependent on peroxynitrite generation. HUVECs were preincubated with 5 µM FeTPPS, a decomposition catalyst of peroxynitrite, followed by stimulation with SIN-1. FeTPPS blocked the increased arginase activity induced by SIN-1 stimulation (Fig. 1C, *p<0.01 vs. untreated; #p<0.01 vs. SIN-1 alone). Peroxynitrite (50 µmol/L, 18 hours) also significantly increased arginase activity (Fig. 1C, *p<0.01 vs. untreated). These data indicate that SIN-1-derived peroxynitrite increased arginase activity.
The increased arginase activity was associated with a decrease in NO metabolites (nitrite and nitrate). As demonstrated in Fig. 1D, SIN-1 stimulation resulted in a time-dependent decrease in NOx production after 12 hours of SIN-1 treatment (Fig. 1D, *p<0.01 vs. untreated). Moreover, peroxynitrite decomposition with FeTPPS (5 µM) also prevented a SIN-1-dependent decrease in NOx production (Fig. 1D, #p<0.01 vs. SIN-1 alone for 18 hours, 97.8±1.3% vs. 80.3±1.1%).
As SIN-1 induced an increase in arginase enzyme activity and a decrease in NOx production, we wished to determine if protein expression levels were changed. As shown in Fig. 1E and F, protein abundance of eNOS was not affected, and iNOS was not detected. However, the expression of arginase I and II proteins increased in SIN-1-treated HUVECs for 18 hours (Fig. 1G and H, *p<0.05 vs. untreated).
Given that SIN-1 stimulation decreased NOx production without affecting eNOS abundance, we wished to determine the molecular mechanism underlying this phenomenon. It is reported that eNOS increases enzymatic activity when the Ser1177 site is phosphorylated and the Thr495 site is de-phosphorylated. As shown in Fig. 2A and B, SIN-1 treatment significantly reduced phospho-eNOS Ser1177 and increased phospho-eNOS Thr495 (Fig. 2B, *p<0.05 vs. untreated). However, arginase inhibition and L-arginine supplement markedly reversed the effect of SIN-1 on eNOS phosphorylation (Fig. 2B, #p<0.05 vs. SIN-1 alone, ##p<0.01 vs. SIN-1 alone).
We next determined the effects of SIN-1 on the stability of eNOS dimers. As shown in Fig. 2C, SIN-1 induced a significant decrease in the abundance of eNOS dimers (Fig. 2C, *p<0.05 vs. untreated, 1.28±0.06 vs. 1.49±0.05). On the other hand, preincubation with ABH and L-arginine before SIN-1 treatment significantly enhanced the stability of eNOS dimers (Fig. 2D, *p<0.01 vs. SIN-1 alone, 1.89±0.08 vs. 1.38±0.05; #p<0.01 vs. SIN-1 alone, 1.93±0.06 vs. 1.38±0.05). These data suggest that an increased intracellular L-arginine concentration may play a major role in eNOS coupling in peroxynitrite-dependent pathological conditions.
With the hypothesis that arginase inhibition and intracellular L-arginine amount are involved in SIN-1-dependent regulation of eNOS activity, we next determined changes in NO and ROS production in the endothelium of isolated mouse aortas using the fluorescent dyes DAF and DHE (see Materials and Methods).5 Incubation of aortic rings with SIN-1 decreased the DAF fluorescence slope (Fig. 3A, *p<0.01 vs. untreated, 0.69±0.08 vs. 1.28±0.34 fluorescence intensity/second). Remarkably, arginase inhibition (ABH, 10 µM) restored the DAF fluorescence rate (Fig. 3A, #p<0.01 vs. SIN-1 alone, 1.41±0.40 vs. 0.69±0.08 fluorescence intensity/second). To confirm the effects of L-arginine supplement on eNOS phosphorylation and dimer stability (Fig. 2), we directly added L-arginine (10 mM) to SIN-1-treated aortic rings and then performed a similar DAF bioassay. The DAF fluorescence rate decrease induced by SIN-1 was markedly attenuated in L-arginine-treated aortic endothelium (Fig. 3A, #p<0.01 vs. SIN-1 alone, 1.24±0.15 vs. 0.69±0.08 fluorescence intensity/second). However, the increased slope of DAF fluorescence by ABH incubation did not change with treatment of iNOS inhibitor (1400W, data not shown). Taken together, these data suggest that the SIN-1-induced decrease in endothelial NO production may be dependent on arginase activity affecting the intracellular L-arginine level. NOS inhibitor, NG-Nitro-L-arginine methyl ester (L-NAME, 100 µM) resulted in almost complete reduction of the slope of DAF fluorescence to 0.
To determine whether arginase activation by SIN-1 was associated with increased ROS production via eNOS uncoupling, we measured O2•- production using DHE in isolated aortas treated with L-NAME (100 µM), ABH (10 µM), L-arginine (10 mM), or all three. There was a significant increase in ROS production in the endothelium of SIN-1 treated aortas (Fig. 3B, *p<0.01 vs. untreated, 2.54±0.97 vs. 1.02±0.33 fluorescence intensity/second). Consistent with this data, ROS production was significantly decreased with ABH treatment (Fig. 3B, #p<0.01 vs. SIN-1 alone, 1.02±0.29 vs. 2.54±0.97 fluorescence intensity/second) and L-arginine incubation (Fig. 3B, #p<0.01 vs. SIN-1 alone, 1.02±0.32 vs. 2.54±0.97 fluorescence intensity/second). Interestingly, incubation of SIN-1-treated aortic rings with L-NAME prevented a SIN-1-induced increase in ROS production, and pretreatment with ABH or L-arginine resulted in a slope similar to the DHE response in untreated aortas (Fig. 3B, NS: non-significant among the groups). These results suggest that enhanced ROS production under peroxynitrite-dependent stress condition is due to eNOS uncoupling.
We also measured the intracellular L-arginine concentration in HUVECs. Under control conditions, the intracellular content of L-arginine was 100±5.0% (170±8.5 µM L-arginine), and the L-arginine content fell to 82.8±4.8% after exposure to SIN-1 (Fig. 4, *p<0.01 vs. untreated). However, preincubation with ABH maintained L-arginine content at 96.9±2.8% (Fig. 4, #p<0.01 vs. SIN-1 alone).
Considering that both arginase inhibition and L-arginine restored the SIN-1-induced decrease in vascular NO production, we determined whether vascular function was altered in SIN-1 treated aortas. First, we determined the vascular response to KCl. SIN-1 treatment did not significantly change the maximal tension development in response to 60 mMKCl (Fig. 5A, NS: non-significant between untreated vs. SIN-1, 1.60±0.12 g vs. 1.45±0.15 g).
The vasoconstriction response to the agonist, U-46619, was markedly enhanced in SIN-1-treated aortas, as manifest by a leftward shift in the dose-response curves, an increase in Emax (Fig. 5B, *p<0.01 vs. untreated, 241.6±5.0% vs. 209.3±5.2%), and a reduction in log EC50 (Fig. 5B, *p<0.01 vs. untreated, -7.92±0.05 M vs. -7.76±0.05 M). However, incubation with ABH for 18 hours significantly reduced Emax (Fig. 5B, #p<0.01 vs. SIN-1 alone, 172.0±6.6% vs. 241.6±5.0%) and increased log EC50 (Fig. 5B, #p<0.01 vs. SIN-1 alone, -7.43±0.07 M vs. -7.92±0.05 M). Moreover, peroxynitrite decomposition with FeTPPS (5 µM) also reduced the Emax of the SIN-1-treated aortas to the same level as the Emax of untreated aortas (Fig. 5B, SIN-1+FeTPPS vs. untreated, 201.7±5.3% vs. 209.3± 5.2%, non-significant).
To determine the effects of arginase inhibition on endothelial-dependent vasorelaxation, mouse aortas were preconstricted with U46619 (10-8 mol/L), and dose-response curves were constructed in response to the endothelial-dependent vasodilator, Ach, and the endothelial-independent NO donor, sodium nitroprusside (SNP). Ach resulted in significant dose-dependent relaxation in the mouse aortas. The vasorelaxation responses to SIN-1 in the aortic rings were significantly attenuated compared with those from untreated controls. The Emax was 85.9±2.3% vs. 90.2±2.5% (Fig. 5C, *p<0.05 vs. untreated), and the log EC50 was -7.10±0.07 M vs. -7.48±0.10 M (Fig. 5C, *p<0.05 vs. untreated). FeTPPS (5 µM) increased the Emax of SIN-1-treated aortas to the untreated control level (Fig. 5C, SIN-1+FeTPPS vs. untreated, 93.2±1.8% vs. 90.2±2.5%, non-significant). Surprisingly, the Emax in vessel rings treated with SIN-1 in the presence of ABH were enhanced compared to those of SIN-1 treated rings (Fig. 5C, #p<0.01 vs. SIN-1 alone, 109.5±2.6% vs. 85.9±2.3%); the log EC50, however, was not significantly changed (Fig. 5C, SIN-1 vs. SIN-1+ABH, -7.10±0.07 M vs. -7.09±0.06 M). De-endothelialized rings did not response to Ach (Fig. 5C). In contrast, vessels preconstricted with U46619 did not show a significant change in cumulative dose responses to SNP among the groups (Fig. 5D).
First, the concentration of peroxynitrite (Calbiochem, Feltham, UK) was determined spectrophotometrically based on an extinction coefficient of 1670 M-1 cm-1 at 302 nm in 1.5 M NaOH. We quantified peroxynitrite generated from SIN-1 decomposition by quenching the NADH fluorescence, which has been reported to correspond to the conversion of NADH to NAD+ by peroxynitrite.13 NADH fluorescence was measured with an excitation wavelength of 340 nm and an emission wavelength of 460 nm (LS-5B, Perkin-Elmer Instruments, Waltham, MA, USA). SIN-1 treatment at 50 µM, which was used in all experiments, generated peroxynitrite at a concentration of 0.65 nM over 30 min.
NO is a vasoprotective molecule that has led to the development of therapies to enhance NO signaling, including dietary L-arginine,1415161718 drug-eluting stents,19 inhalational NO gas,2021 NOS gene therapy,2223 and recently arginase inhibition.1524 Unfortunately, gene therapy remains limited due to problems with the delivery system and vectors, as well as mutational concerns. Inhalational NO gas has a limited use in terms of dose, exposure, and systemic toxicity.2526 Pharmacological NO donors may contribute to vascular injury prior to initiation of therapy due to surgical manipulation. Moreover, oral L-arginine therapy generated controversial results.151617 On the other hand, inhibition of arginase, a novel therapeutic target, actively augments NO production, and has beneficial effects on cardiac and vascular wall function in atherogenesis, diabetes, and aging.1452427 Our data support the hypothesis that arginase inhibiton, as well as L-arginine supplementation, has protective effects on endothelial dysfunction and hypertension.
The activation and expression of endothelial arginase can be induced by a variety of vascular insults, although the dominance of arginase I and II expression may depend on the organ, disease state, and species.28 Angiotensin II is known to elevate arginase activity through the induction of arginase I via RhoA/RhoA activated kinase (ROCK) activation.29 Interestingly, arginase I activity was increased by stabilization of the arginase I trimer via S-nitrosylation at Cys303,6 which required direct interaction with the oxygenase domain of iNOS.30 In the case of arginase II, the enzymatic activity was increased in endothelial cells by incubation with thrombin31 and oxidized low-density lipoprotein (oxLDL).3 These agonists, signaling through RhoA-dependent ROCK activation, activate arginase II without effecting arginase protein expression. Taken together, it seems that activation of the RhoA/ROCK pathway can elevate arginase expression and activity. Interestingly, a previous study reported that the RhoA molecule also contains a redox-active cysteine (Cys18), which ROS and reactive nitrogen species (RNS) may target. ROS and RNS, particularly the superoxide anion radical (O2•-) and nitrogen dioxide (•NO2), can enhance GDP release from RhoA and thus modulate RhoA activity.32
Peroxynitrite is a highly reactive oxidant formed by the reaction of superoxide and NO33 and is an indicator of the redox state. Increased formation of peroxynitrite, referred to as redox imbalance, has a wide array of tissue-damaging effects in vivo, including lipid peroxidation, inactivation of enzymes and ion channels via protein oxidation and nitration, and inhibition of mitochondrial respiration.33 Peroxynitrite has been detected in disease conditions such as diabetes, ischemia-reperfusion, sepsis, inflammation, and atherosclerosis.343536 Endothelial NOS may play an important role in the regulation of redox balance in endothelial cells, as ROS and NO production are dependent on the stability of the eNOS dimer. Several mechanisms could explain the uncoupling of eNOS to produce predominantly ROS in pathophysiological conditions including: 1) substrate (L-arginine) depletion, 2) cofactor (BH4) depletion, 3) loss of dimerization, and 4) altered eNOS phosphorylation. Among them, peroxynitrite can induce eNOS uncoupling via BH4 depletion (an oxidation reaction in BH2), disruption of the eNOS-heme complex,37 and oxidation of the zinc-thiolate complex.38 Peroxynitrite can also nitrosylate the cationic amino acid transporter, resulting in decreased endothelial L-arginine transport and an increased rate of arginine efflux, thus reducing intracellular L-arginine concentration and negatively effecting NO production.39 Furthermore, increased iNOS-dependent NO can induce increased arginase I enzyme activity by S-nitrosylation leading to decreased intracellular L-arginine bioavailability.6 These effects of peroxynitrite might explain why chronic treatment for 18 hours with SIN-1 resulted in activation of arginase (Fig. 1A, B, and C) and decreased NO production (Fig. 1D and 3A), although eNOS expression did not change (Fig. 1E).
Reduced intracellular BH4 level has been demonstrated as a cause of endothelial NOS uncoupling in experimental vascular disease states. It is also reported that decreased L-arginine bioavailability alone is sufficient to uncouple NOS.40 Interestingly, preincubation with arginase inhibitor or L-arginine prevented peroxynitrite-dependent eNOS uncoupling (Fig. 2D). This result is consistent with a previous report showing that inhibition of arginase prevented the decrease in eNOS dimers in endothelial cells after treatment with oxLDL, and the effect of arginase on NOS uncoupling was independent of vascular BH4 concentration.5 Furthermore, our data suggest that increased arginase activity is associated with eNOS uncoupling, and enhancement of the L-arginine amount may play a critical role in maintaining the stability of eNOS dimers through increased eNOS phosphorylation at Ser1177 and decreased phosphorylation at Thr495, which contribute to the restoration of the nitroso-redox balance in endothelial cells (Fig. 5). Hence, arginine inhibition and L-arginine supplementation are able to reverse the effects of peroxinitrite on endothelial cells, as shown in our data.
In conclusion, peroxynitrite stimulation induced an increase in arginase activity and reciprocally regulated NO production that was restored by increasing the L-arginine level. The increased L-arginine level was associated with eNOS dimerization via eNOS phosphorylation at Ser1177. Furthermore, arginase inhibition and L-arginine supplementation attenuated peroxynitrite-induced vascular dysfunction by a mechanism involving increased stability of eNOS dimers.
Figures and Tables
Fig. 1
Peroxynitrite generated by SIN-1 increased arginase activity and expression. (A) HUVECs were incubated with SIN-1 (50 µM) at different time points, and then arginase activity was measured (*p<0.05 vs. untreated). (B) HUVECs were incubated with increasing doses of SIN-1 (5–100 µM) for 18 h, after which cellular arginase activity was measured (*p<0.01 vs. untreated). (C) HUVECs were incubated with SIN-1 (50 µM, 18 h) in the presence or absence of the peroxynitrite scavenger, FeTPPS (5 µM), and arginase activity was measured (*p<0.01 vs. untreated, #p<0.01 vs. SIN-1 alone). ONOO-(50 µM, 18 h) was used as the control (*p<0.01 vs. untreated). (D) HUVECs were incubated with SIN-1 at different time points, and cellular NOx was measured. SIN-1 resulted in a significant decrease in endothelial-cell NOx production. Preincubation of cells with FeTPPS (5 µM) prevented the SIN-1-induced decrease in NOx production [*p<0.01 vs. untreated, #p<0.01 vs. SIN-1 (18 h)]. HUVECs, human umbilical vein endothelial cells; NOx, nitrite/nitrate. (E) Protein abundance was analyzed in HUVECs treated with SIN-1 (50 µM, 18 h). Densitometry analysis was performed [F, G, and H, *p<0.05 vs. untreated; PC, positive control for iNOS (RAW264.7 macrophage stimulated with lipopolysaccharide)]. HUVECs, human umbilical vein endothelial cells; NOx, nitrite/nitrate; iNOS, inducible nitric oxide synthase.
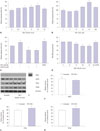
Fig. 2
Effects of peroxynitrite on eNOS phosphorylation and dimerization were prevented by arginase inhibition and L-arginine supplement. (A) Western blot and (B) quantitative analysis of eNOS phosphorylation at Ser1177 and Thr495. SIN-1 treatment reduced p-eNOS Ser1177 and increased p-eNOS Thr495 (*p<0.05 vs. untreated), which was reversed by arginase inhibitor, ABH (10 µM for 2 h) and L-arginine supplement (10 mM for 2 h; #p<0.05 vs. SIN-1 alone, ##p<0.01 vs. SIN-1 alone). (C) Native low-temperature SDS-PAGE and Western blot analysis were performed after SIN-1 treatment (*p<0.05 vs. untreated). (D) The reduced ratio of eNOS dimer/monomer was significantly restored by preincubation with ABH (10 µM, *p<0.01 vs. SIN-1 alone) and L-arginine supplement (10 mM, #p<0.01 vs. SIN-1 alone). L-Arg, L-arginine; eNOS, endothelial nitric oxide synthase.
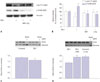
Fig. 3
Incubation with arginase inhibitor and L-arginine altered NO production and ROS generation in SIN-1-stimulated mouse aortas. (A) Isolated aortic rings were incubated with SIN-1 (50 µM, 18 h), and endothelial NO production (endothelial side up) was measured using DAF-FM (5 µM). SIN-1 incubation resulted in a decreased slope of DAF-FM (*p<0.01 vs. untreated) that was restored to untreated control levels by treatment with the arginase inhibitor, ABH (10 µM), and L-arginine (10 mM; #p<0.01 vs. SIN-1 alone). L-NAME was used as a control. (B) ROS generation from the aortic endothelium was measured after preloading with DHE (5 µM; *p<0.01 vs. untreated; #p<0.01 vs. SIN-1 alone), whereas L-NAME (10 µM) treatment prevented the ROS generation induced by SIN-1 stimulation (NS, non-significant among the groups). Mn(III)tetrakis (4-benzoic acid) porphyrin (MnTBAP) was used as a superoxide scavenger. NO, nitric oxide; ROS, reactive oxygen species; DHE, dihydroethidine; L-NAME, NG-Nitro-L-arginine methyl ester.
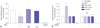
Fig. 4
Effect of arginase inhibition on intracellular L-arginine concentration. Intracellular L-arginine concentration was measured using HPLC (see Materials and Methods section). Treatment of HUVECs with SIN-1 resulted in decreased intracellular L-arginine that was recovered by pretreatment with the arginase inhibitor, ABH (*p<0.01 vs. untreated, #p<0.01 vs. SIN-1 alone; n=6). HPLC, high-performance liquid chromatography; HUVECs, human umbilical vein endothelial cells.
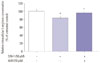
Fig. 5
Arginase inhibition restored impaired vascular function induced by SIN-1 in mouse aortas. (A) Constriction was induced by high potassium in SIN-1-treated and -untreated descending aortas. Constrictions induced by a high-potassium solution did not differ between groups (NS, non-significant). (B) Contractile responses to the agonist, U46619, were significantly enhanced in SIN-1-incubated aortic rings, and incubation with the arginase inhibitor (ABH, 10 µM) attenuated vasoconstrictor responses to U46619 (*p<0.01 vs. untreated; #p<0.01 vs. SIN-1 alone). Vessels were preconstricted, and cumulative dose responses to Ach (C) and SNP (D) were performed. The vasorelaxant EC50 to Ach was attenuated in aortic rings incubated with SIN-1 (*p<0.05 vs. untreated). Arginase inhibition enhanced maximal vasorelaxation in SIN-1-treated vessels (#p<0.01 vs. SIN-1 alone). De-endothelialized vessels did not respond to Ach. Vasorelaxant responses to SNP did not differ among groups. Ach, acetylcholine; SNP, sodium nitroprusside.
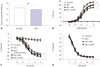
ACKNOWLEDGEMENTS
This work was supported by the Basic Science Research Program of the National Research Foundation of Korea (NRF), funded by the Ministry of Education, Science and Technology (2015R1D1A3A01017911), by the Small and Medium Business Administration (C-1012329-01-01), and by a 2014 Research grant from Kangwon National University (No. 120140393).
References
1. Berkowitz DE, White R, Li D, Minhas KM, Cernetich A, Kim S, et al. Arginase reciprocally regulates nitric oxide synthase activity and contributes to endothelial dysfunction in aging blood vessels. Circulation. 2003; 108:2000–2006.


2. Demougeot C, Prigent-Tessier A, Marie C, Berthelot A. Arginase inhibition reduces endothelial dysfunction and blood pressure rising in spontaneously hypertensive rats. J Hypertens. 2005; 23:971–978.


3. Ryoo S, Lemmon CA, Soucy KG, Gupta G, White AR, Nyhan D, et al. Oxidized low-density lipoprotein-dependent endothelial arginase II activation contributes to impaired nitric oxide signaling. Circ Res. 2006; 99:951–960.


4. White AR, Ryoo S, Li D, Champion HC, Steppan J, Wang D, et al. Knockdown of arginase I restores NO signaling in the vasculature of old rats. Hypertension. 2006; 47:245–251.


5. Ryoo S, Gupta G, Benjo A, Lim HK, Camara A, Sikka G, et al. Endothelial arginase II: a novel target for the treatment of atherosclerosis. Circ Res. 2008; 102:923–932.
6. Santhanam L, Lim HK, Lim HK, Miriel V, Brown T, Patel M, et al. Inducible NO synthase dependent S-nitrosylation and activation of arginase1 contribute to age-related endothelial dysfunction. Circ Res. 2007; 101:692–702.


7. Hwang HM, Lee JH, Min BS, Jeon BH, Hoe KL, Kim YM, et al. A novel arginase inhibitor derived from scutellavia indica restored endothelial function in ApoE-null mice fed a high-cholesterol diet. J Pharmacol Exp Ther. 2015; 355:57–65.


8. Förstermann U, Münzel T. Endothelial nitric oxide synthase in vascular disease: from marvel to menace. Circulation. 2006; 113:1708–1714.
9. Belik J, Stevens D, Pan J, McIntyre BA, Kantores C, Ivanovska J, et al. Pulmonary vascular and cardiac effects of peroxynitrite decomposition in newborn rats. Free Radic Biol Med. 2010; 49:1306–1314.


10. Sankaralingam S, Xu H, Davidge ST. Arginase contributes to endothelial cell oxidative stress in response to plasma from women with preeclampsia. Cardiovasc Res. 2010; 85:194–203.


11. Böger RH, Bode-Böger SM, Mügge A, Kienke S, Brandes R, Dwenger A, et al. Supplementation of hypercholesterolaemic rabbits with L-arginine reduces the vascular release of superoxide anions and restores NO production. Atherosclerosis. 1995; 117:273–284.


12. Mohr S, Stamler JS, Brüne B. Mechanism of covalent modification of glyceraldehyde-3-phosphate dehydrogenase at its active site thiol by nitric oxide, peroxynitrite and related nitrosating agents. FEBS Lett. 1994; 348:223–227.


13. Martin-Romero FJ, Gutiérrez-Martin Y, Henao F, Gutiérrez-Merino C. Fluorescence measurements of steady state peroxynitrite production upon SIN-1 decomposition: NADH versus dihydrodichlorofluorescein and dihydrorhodamine 123. J Fluoresc. 2004; 14:17–23.


14. Blum A, Hathaway L, Mincemoyer R, Schenke WH, Kirby M, Csako G, et al. Oral L-arginine in patients with coronary artery disease on medical management. Circulation. 2000; 101:2160–2164.


15. Dudek D, Legutko J, Heba G, Bartus S, Partyka L, Huk I, et al. L-arginine supplementation does not inhibit neointimal formation after coronary stenting in human beings: an intravascular ultrasound study. Am Heart J. 2004; 147:E12.


16. Walker HA, McGing E, Fisher I, Böger RH, Bode-Böger SM, Jackson G, et al. Endothelium-dependent vasodilation is independent of the plasma L-arginine/ADMA ratio in men with stable angina: lack of effect of oral L-arginine on endothelial function, oxidative stress and exercise performance. J Am Coll Cardiol. 2001; 38:499–505.


17. Wilson AM, Harada R, Nair N, Balasubramanian N, Cooke JP. L-arginine supplementation in peripheral arterial disease: no benefit and possible harm. Circulation. 2007; 116:188–195.


18. Böger RH. L-Arginine therapy in cardiovascular pathologies: beneficial or dangerous? Curr Opin Clin Nutr Metab Care. 2008; 11:55–61.


19. Ansel GM, Lumsden AB. Evolving modalities for femoropopliteal interventions. J Endovasc Ther. 2009; 16:2 Suppl 2. II82–II97.


20. Griffiths MJ, Evans TW. Inhaled nitric oxide therapy in adults. N Engl J Med. 2005; 353:2683–2695.


21. Ichinose F, Roberts JD Jr, Zapol WM. Inhaled nitric oxide: a selective pulmonary vasodilator: current uses and therapeutic potential. Circulation. 2004; 109:3106–3111.
22. Barbato JE, Kibbe MR, Tzeng E. The emerging role of gene therapy in the treatment of cardiovascular diseases. Crit Rev Clin Lab Sci. 2003; 40:499–545.


23. Kibbe MR, Tzeng E. Nitric oxide synthase gene therapy in vascular pathology. Semin Perinatol. 2000; 24:51–54.


24. Romero MJ, Platt DH, Tawfik HE, Labazi M, El-Remessy AB, Bartoli M, et al. Diabetes-induced coronary vascular dysfunction involves increased arginase activity. Circ Res. 2008; 102:95–102.


25. Matsumoto A, Momomura S, Hirata Y, Aoyagi T, Sugiura S, Omata M. Inhaled nitric oxide and exercise capacity in congestive heart failure. Lancet. 1997; 349:999–1000.


26. Roger N, Barberà JA, Roca J, Rovira I, Gómez FP, Rodriguez-Roisin R. Nitric oxide inhalation during exercise in chronic obstructive pulmonary disease. Am J Respir Crit Care Med. 1997; 156(3 Pt 1):800–806.


27. Steppan J, Ryoo S, Schuleri KH, Gregg C, Hasan RK, White AR, et al. Arginase modulates myocardial contractility by a nitric oxide synthase 1-dependent mechanism. Proc Natl Acad Sci U S A. 2006; 103:4759–4764.


28. Morris SM Jr. Recent advances in arginine metabolism: roles and regulation of the arginases. Br J Pharmacol. 2009; 157:922–930.


29. Shatanawi A, Romero MJ, Iddings JA, Chandra S, Umapathy NS, Verin AD, et al. Angiotensin II-induced vascular endothelial dysfunction through RhoA/Rho kinase/p38 mitogen-activated protein kinase/arginase pathway. Am J Physiol Cell Physiol. 2011; 300:C1181–C1192.


30. Dunn J, Gutbrod S, Webb A, Pak A, Jandu SK, Bhunia A, et al. S-nitrosation of arginase 1 requires direct interaction with inducible nitric oxide synthase. Mol Cell Biochem. 2011; 355:83–89.


31. Ming XF, Barandier C, Viswambharan H, Kwak BR, Mach F, Mazzolai L, et al. Thrombin stimulates human endothelial arginase enzymatic activity via RhoA/ROCK pathway: implications for atherosclerotic endothelial dysfunction. Circulation. 2004; 110:3708–3714.


32. Heo J, Campbell SL. Mechanism of redox-mediated guanine nucleotide exchange on redox-active Rho GTPases. J Biol Chem. 2005; 280:31003–31010.


33. Beckman JS, Koppenol WH. Nitric oxide, superoxide, and peroxynitrite: the good, the bad, and ugly. Am J Physiol. 1996; 271(5 Pt 1):C1424–C1437.


34. Wang P, Zweier JL. Measurement of nitric oxide and peroxynitrite generation in the postischemic heart. Evidence for peroxynitrite-mediated reperfusion injury. J Biol Chem. 1996; 271:29223–29230.


35. Torres-Dueñas D, Celes MR, Freitas A, Alves-Filho JC, Spiller F, Dal-Secco D, et al. Peroxynitrite mediates the failure of neutrophil migration in severe polymicrobial sepsis in mice. Br J Pharmacol. 2007; 152:341–352.


36. Salvemini D, Doyle TM, Cuzzocrea S. Superoxide, peroxynitrite and oxidative/nitrative stress in inflammation. Biochem Soc Trans. 2006; 34(Pt 5):965–970.


37. Zou MH, Shi C, Cohen RA. Oxidation of the zinc-thiolate complex and uncoupling of endothelial nitric oxide synthase by peroxynitrite. J Clin Invest. 2002; 109:817–826.


38. Chen W, Druhan LJ, Chen CA, Hemann C, Chen YR, Berka V, et al. Peroxynitrite induces destruction of the tetrahydrobiopterin and heme in endothelial nitric oxide synthase: transition from reversible to irreversible enzyme inhibition. Biochemistry. 2010; 49:3129–3137.

