Abstract
Purpose
Stem cell-based therapies represent new promises for the treatment of urinary incontinence. This study was performed to assess optimized cell passage number, cell dose, therapeutic efficacy, feasibility, toxicity, and cell trafficking for the first step of the pre-clinical evaluation of human amniotic fluid stem cell (hAFSC) therapy in a urinary incontinence animal model.
Materials and Methods
The proper cell passage number was analyzed with hAFSCs at passages 4, 6, and 8 at week 2. The cell dose optimization included 1×104, 1×105, and 1×106 cells at week 2. The in vivo cell toxicity was performed with 0.25×106, 0.5×106, and 1×106 cells at weeks 2 and 4. Cell tracking was performed with 1×106 cells at weeks 2 and 4.
Results
The selected optimal cell passage number was smaller than 6, and the optimal cell dose was 1×106 for the mouse model. In our pre-clinical study, hAFSC-injected animals showed normal values for several parameters. Moreover, the injected cells were found to be non-toxic and non-tumorigenic. Furthermore, the injected hAFSCs were rarely identified by in vivo cell trafficking in the target organs at week 2.
Treatment for stress urinary incontinence (SUI) has had limited success with frequent adverse reactions.1 Stem cell therapy has been proposed as an alternative to overcome the limitations and side effects of existing therapeutic procedures.2 Human amniotic fluid stem cells (hAFSCs) have been reported recently as a promising stem cell source.3,4 For the development of hAFSCs as a feasible cell-based medicinal product, pre-clinical studies are mandatory. Major parameters for pre-clinical studies are cell passage number and dose, efficacy, toxicity and tumorigenicity, and cell trafficking in an animal model.5,6 For example, the selection of optimized passage number has been reported to be an important factor since mesenchymal stem cell potency is inversely passage-dependent.7,8,9 Cell dose is another critical factor,10 as high doses of cells may cause toxicity or tumorigenicity, whereas low doses result in insufficient therapeutic effect.11 Therefore, the optimum dose range of transplanted hAFSCs for SUI treatment needs to be identified.
The efficacy of hAFSC products should be evaluated for their application as medicinal products. The design of an efficient assay should comprise both functional and marker-based assays. Understanding the biological mechanism of the therapeutic effect will provide a solid basis.12 Examples of biological efficacy include evaluation of differentiation into myogenic and neurogenic lineages as well as endothelial genesis and immunogenicity.
Undifferentiated and proliferative stem cells have a potential risk of tumor formation, which may depend on the origin of cells. hAFSCs are classified as mesenchymal stem cells, but show pluripotent ability like embryonic stem cells13 and give rise to safety concerns when they are implanted into a target tissue. Therefore, tumorigenicity verification is required before the initial clinical application of hAFSCs.
In stem cell therapy, injected cell trafficking is important because cell migration might yield unwanted results. The fate of injected cells is dependent on the route of administration and the site of implantation. In this study, injected cells were identified with target organs since the risk of ectopic tissue formation is associated with stem cell distribution.
This study serves as an initial pre-clinical investigation for the selection of optimized hAFSC passage number and dose as well as an examination of hAFSC efficacy, toxicity, tumorigenicity, and cell trafficking in an animal model. For the selection of optimized cell passage number and dose, urodynamic analysis was performed. With the optimized cell passage number and dose, the therapeutic effect of hAFSCs for the regeneration of the urethral sphincter was then analyzed. Toxicity and tumorigenicity were evaluated according to the standard parameters. For cell trafficking, injected human cells were detected with human cell-specific
antibodies at targeted organs.
This study was completed in compliance with Good Laboratory Practices (GLP) and followed the standards of toxicity testing from the Korea Food & Drug Administration. This study was approved by the Animal Ethics Committee of Biotoxtec Inc. and the Ethics Committee of Kyungpook National University School of Medicine.
Amniotic fluids (10 mL) were obtained from four women undergoing routine amniocentesis at a gestational age of 15 to 19 weeks at Kyungpook National University Hospital, and sent to CORESTEM Inc. (Seoul, Korea) for cell preparation in the Good Manufacturing Practices (GMP) facility. During cell preparation, validation of the manufacturing process, in vitro assessment of genotypic instability, tumorigenicity, and phenotypic profile of the product were conducted. In case #AFSC1301, a cell population with high expression of C-KIT and SSEA4 (stem cell markers) as well as low expression of HLA-DR (immune tolerance marker) was selected and used for this study.
Five-week-old male and female ICR mice were purchased from Orientbio Inc. (Seongnam, Korea). Animal groupings were listed in Table 1. For these experiments, cells from passage 4 were used to ensure a sufficient cell number, and the maximum cell number was 1×106 cells for injection in the mouse urethral sphincter. In the toxicity analysis, animals of both genders were used to eliminate gender differences. ICR mice were prepared for aseptic surgery under general anesthesia with isoflurane. The SUI model was created using a bilateral pudendal nerve transection technique. One week after the incompetent urethral sphincter model was generated, hAFSCs were resuspended in saline (Choongwae Pharma Corp., Seoul, Korea) under aseptic conditions to a final volume of 5 µL and injected at the external sphincter.
Leak point pressure (LPP) and closing pressure (CP) were measured two weeks after cell injection as previously described.13 The intravesical pressure was increased from 0 cm H2O upward in steps of 1- to 3-cm H2O until visual identification of the leak point height. The pressure at this leak point was referred to as the LPP. The intravesical pressure was then decreased downward in steps of 1- to 3-cm H2O until the leak has ceased. The pressure at this leak cessation point was taken as the CP.3 Tissue specimens were prepared for hematoxylin and eosin (H&E) staining and immunohistochemical (IHC) staining. The possibility of host myogenic, neurogenic, and endothelial genetic conversion induced by the in vivo injection of hAFSCs was analyzed with IHC (antibody information, Table 2). The in vivo immunosuppression effect was examined one week after injection with a cytotoxic T cell marker (CD8). For injected human cell trafficking and analysis of migration to target organs, human nuclear specific antibody (HuNu) was used. The IHC results were confirmed with real-time PCR using human primers and host responses were analyzed with mouse primers (primer sequence information, Table 3). Total RNA was extracted with an RNeasy-kit (Qiagen, Hilden, Germany) according to the manufacturer's instructions. A total of 2 µg of RNA was used for cDNA synthesis using cDNA reverse transcription kits (Applied Biosystems, Warrington, UK). The primers were designed with Primer Express Software (Applied Biosystems). Assays were carried out using the ABI Prism Sequence Detection System 7500 with SYBR Green PCR Master Mix (Applied Biosystems). To analyze data, the 2-ΔΔCt method of relative quantification was adapted to estimate copy numbers.
With optimized cell number and dose, pre-clinical aspects were analyzed. On the day of dosing, general symptoms were examined at each time point. The mortalities were measured and pathological examinations were carried out immediately before treatment and every other week after treatment for four weeks. After the termination of observations, all animal organs and tissues were visually inspected and examined by microscopy for necropsy.
All values are presented as mean±SD. Statistical analysis was undertaken with Student's t-test or one-way analysis of variance (ANOVA) using the Statistical Package for the Social Sciences v.19 (SPSS, IBM, Armonk, NY, USA). A p-value of less than 0.05 was considered statistically significant. When the value was found to be significant after assessment using the ANOVA statistical test, the Tukey's post-hoc comparison was used.
LPP and CP were measured two weeks after injection of hAFSCs at different passages (Fig. 1A). The mean LPP values for passages 4 and 6 were significantly higher than the mean LPP of the Ctrl(+) group, and the mean LPP of passage 8 was similar to that of the Ctrl(+) group. The mean CP values for passages 4 and 6 were similar to the mean CP value of the Ctrl(+) group. At passage 8, the mean CP value decreased significantly, even though the values were higher than those of the Ctrl(-) group. Real-time PCR analysis for myogenic, neurogenic, and endothelial lineage markers of passage 4 showed significant gene expression, and the degree of expression was inversely proportional to the number of passages (Fig. 1B). With this result, the optimized passage number was selected to be smaller than 6. When using cells prior to passage 4, cell number was limited for several experiments.
LPP and CP were measured two weeks after injection of hAFSCs (Fig. 1C). The mean LPP for the group injected with 1×106 cells was similar to that of the Ctrl(+) group (p=0.874), and it was significantly different from the groups with doses of 1×105 and 1×104 cells (p=0.003). The mean CP for the 1×106 dose group was significantly different from the 1×105 and 1×104 dose groups (p=0.012 and p=0.003, respectively). Real-time PCR analysis for myogenic, neurogenic, and endothelial lineage markers showed that gene expression for the 1×106 dose group was significant and directly proportional to the number of cells (Fig. 1D). With this result, the optimized cell dose for injection was selected to be 1×106. This cell number is the maximum in this animal model because of the limited size of the urethral sphincter.
The therapeutic efficacy of hAFSCs was evaluated using cells obtained at passage 6 and a cell number of 1×106 at two weeks after injection. Histological examination revealed no apparent sign of inflammation or tissue damage at the injection sites (Fig. 2A). Compared with the Ctrl(+) group, the Ctrl(-) group showed only a scant muscle layer at the atrophic urethral sphincter. In the hAFSCs group, a normal appearance of circular muscle mass regeneration and a number of capillaries were identified at the urethral sphincter region. This histological result was confirmed by IHC staining using myogenesis-, neurogenesis-, and endothelial genesis-related antibodies. The hAFSCs group was positive for the antibodies and the intensity was higher in this group compared with the Ctrl(-) group. In immunogenicity analysis, CD8 signals for cytotoxic T cells were not detected in the hAFSCs group. The histologic results were confirmed with real-time PCR analysis. The evaluated genes were related to myogenesis, neurogenesis, and endothelial lineage differentiation. In mouse primer analysis at week 2, most of the genes were expressed at significantly higher levels in the hAFSCs group than in the Ctrl(-) group, with the exception of Nestin and CD34 (Fig. 2B). In contrast, human gene expressions were relatively decreased compared with mouse gene expression (Fig. 2C).
At week 2 after cell injection, one female mouse from the control group had corneal opacity, and a male mouse had secondary wound closure. However, these symptoms were within the tolerance levels and were not considered significant. With respect to weight change, food consumption, ophthalmology test, urine test, hematological test, clinical chemistry, estrous cycle, sperm motility, and organ weight, no significant changes were found between the experimental groups and the control group. At necropsy, there were no meaningful changes observed by histopathological examination in all animal groups, and there were no significant changes related to the injections in the brain, lungs, liver, kidney, and spinal cord. Histopathological findings showed no connection to the test materials (Table 4).
In vivo histological and IHC analysis with HuNu antibody identified the injected hAFSCs in situ. With H&E staining, the experimental groups showed normal organ histology compared to controls (Fig. 3A and B). IHC staining revealed focal detection of injected cells at the injection site and target organs two weeks after cell injection (Fig. 3C and D). Upon analysis of cell migration to other organs, cells were detected in the brain, heart, kidney, lung, spleen, prostate, thymus, testis, ovary, and urethra. The kidney (one case), spleen (four cases), prostate (one case), thymus (one case), and urethra (three cases) showed resident injected cells, which indicated that the injected cells migrated into neighboring organs. In the male experimental group, 7/24 (29.17%) cases showed HuNu positive cells in the kidney (2 cells in one case), spleen (3, 6, 2, 2 cells in four cases), prostate (1 cell in one case), and urethra (1 cell in one case). In the female experimental group, 2/24 (16.6%) cases showed HuNu positive cells in the thymus and urethra (1 cell at each organ in 1 case) and urethra (1 cell in 1 case). When compared with the injected cell number (1×106), the positive cell number was significantly lower, and the positive cells were not detected at week 4 (data not shown). Therefore, injected cell migration and resident cell influence were not considered a factor for hAFSC therapy.
To evaluate whether hAFSCs may provide a feasible cell-based clinical product for treatment of SUI, pre-clinical parameters, including optimal cell passage number, dose, efficacy, toxicity, tumorigenicity, and cell trafficking were eval-uated.
Selected cell passage numbers smaller than 6 showed to be optimal through urodynamic results in vivo. This passage number was similar to that described by Choi, et al.14 who have reported in vitro data with bone marrow mesenchymal stem cells (MSCs) with the early passages (1, 3, and 5) and the late passages (7 and 9). The early passage cells showed rapidly proliferating, small, and spindle-like cells, whereas the cells from the late passages comprised of larger and slower dividing cells. Critical passages were determined to be between 5 and 7, where the number of large cells (slower dividing) increased in the cell population. Furthermore, the doubling time was expanded two- to three-fold. It was suggested that the smaller spindle-like cells could give rise to larger flatter cells through asymmetric cell division as well as a rapid progression of cell senescence with telomere shortening. In addition, the late passage cells showed reduced ability to differentiate into adipocytes, osteocytes, or chondrocytes.14,15 In our PCR results, myogenic, neurogenic, and endothelial genetic differential abilities were inversely dependent on passage number. The late passage cells also demonstrated gradually decreased cytokine and growth factor activity.14,16,17 Modulation of the microenvironment by MSCs plays an important role in the maintenance of stem cell properties and differentiation.14 Considering these reported in vitro results, hAFSCs with fewer than 6 passages could maintain the secretion of cell survival molecules, cytokines, and growth factors, which might provide a beneficial effect.
Cell dose selection is important for identification of safety and cost-saving strategies. The objective of our cell dose study was to compare low (1×104), moderate (1×105), and high (1×106) doses of hAFSC injections. The functional analysis of the sphincters revealed that LPP and CP measured in the high dose hAFSC-injected group were restored to normal values, while the values of the low and moderate cell dose groups had reduced functional recovery of the urethral sphincter muscle. The PCR results showed that myogenic, neurogenic, and endothelial genetic differential abilities were dependent on cell dose. These results indicate that periurethral injection of higher doses of hAFSCs into a denervated urethral sphincter can effectively restore normal urethral sphincter function. It should be considered that this selected cell number was obtained using the mouse model. Therefore, when applied to a clinical trial, the cell number should be optimized for human patients.
This study evaluated general toxicity and tumorigenicity by clinical signs and examinations. There were no significant differences between the experimental and control groups regarding clinical outcome, hematology, and clinical chemistry analysis. Although necropsy and histopathological analysis showed minor organ profile alterations, no significant toxicity was noted and any changes were determined to be accidental and not associated with hAFSC injections
In vivo trafficking of stem cells after grafting is essential for understanding the effects of stem cells on migrational properties and potential complications to target organs. After two weeks, few hAFSCs migrated into the kidney, spleen, thymus, prostate, and urethra, however, the cells frequently populated the spleen. A cause for hAFSC migration into these organs may be due to the fact that the spleen and kidney are the main blood filtration organs, and the injected cells could localize at these locations following circulation. In the case of the spleen, it is reported to be an organ that harbors a unique population of multipotent stem cells. Moreover, it is capable of self-renewal, differentiation, restoration, and/or changes in the functions of numerous tissues.18 The thymus has also been identified to attract hAFSC localization. Thus, our putative explanation is the existence of similar surface markers19 expressed in thymus cells and MSCs,20 which could lead to the migration of hAFSCs to the thymus. Localization in the prostate might be explained by the proximity of the prostate to the injection site. Thus, some exudated cells could have been induced into the prostate. The low detection of hAFSCs in the urethra is based on previously reported data.13 The injected hAFSCs gradually disappeared over the time course, and the existing cell number in the urethra was below the detectable range by day 14.
In future studies, several different types of hAFSCs should be tested as primary cultured hAFSCs could show varying results. Additionally, cell number will need to be increased to accommodate large animal models to achieve the proximate results of a clinical trial.
In this study, the selected optimal cell passage number was determined to be smaller than 6, and the optimal cell dose was determined to be 1×106 total cells for the mouse model. In our pre-clinical study, hAFSC-injected animals demonstrated normal values for the measured parameters, and hAFSCs were found to be non-toxic and non-tumorigenic. For in vivo cell trafficking, the injected hAFSCs were rarely identified in the target organs at week 2. Therefore, our study demonstrates for the first time a safe and effective pre-clinical model of hAFSC injection to treat urinary incontinence, providing a basis for future clinical applications.
Figures and Tables
![]() | Fig. 1Effect of hAFSC passage numbers and doses for urodynamics. (A) The mean leak point pressures (LPPs) for the groups of passages 4, 6, and 8 were similar to those of the Ctrl(+) group, while the closing pressure (CP) was significantly lower at passage 8 at two weeks after cell injection. (B) The urodynamic results according to the hAFSC passage numbers were confirmed with real-time PCR analysis at the same period. (C) The mean LPP and CP values for the 1×106 dose group was similar only to Ctrl(+) at two weeks after cell injection. (D) The urodynamic results were confirmed with real-time PCR analysis at the same period. The different letters on top of the bars show significant differences at p<0.05 by ANOVA Tukey's post-hoc test. Ctrl(+): sham operated normal control group, P4, P6, and P8: passages 4, 6, and 8 cell injected group after bilateral pudendal nerve transaction, respectively, 106, 105, and 104: cell injected group with number of 106, 105, and 104, respectively, Ctrl(-): PBS-injected (without cell) negative control group after bilateral pudendal nerve transaction. hAFSC, human amniotic fluid stem cell. |
![]() | Fig. 2Evaluation of efficacy at passage 6 with 1×106 hAFSCs. (A) Histological and immunohistochemical analysis at two weeks after cell injection. hAFSC-injected groups showed circular muscle mass regeneration, enhanced myogenesis, neurogenesis and endothelial genesis, and non-immunogenicity. (B) Real-time PCR analysis of the expression of myogenesis, neurogenesis, and endothelial genesis lineage markers with mouse primer at the same period. Most of genes were expressed significantly higher in hAFSC groups than in the Ctrl(-) group, except for Nestin and CD34. The different letters on top of the bars show significant differences at p<0.05. (C) Real-time PCR analysis with human primer at week 2. The human gene expressions were relatively decreased compared with mouse gene expression. Magnification at ×100. Ctrl(+): sham operated normal control group, Ctrl(-): PBS-injected (without cell) negative control group after bilateral pudendal nerve transaction, AFSC: hAFSC-injected group after bilateral pudendal nerve transaction. hAFSC, human amniotic fluid stem cell; H&E, hematoxylin and eosin. |
![]() | Fig. 3Histological and immunohistochemical analysis for in vivo cell trafficking. (A and B) Representative images of histological analysis at two weeks after cell injection. With H&E staining, target organs of the experimental groups (A, cell-injected) showed normal histology as the control groups (B, PBS-injected). (C and D) Representative images of immunohistochemical analysis at two weeks after cell injection. The spleen and urethra showed resident injected cells in the experiment group (D). The nucleus is shown as a blue dot with DAPI staining, and yellow arrows indicate detected hAFSCs with human nucleus-specific antibody in target organs. Magnification at ×100. hAFSC, human amniotic fluid stem cell; H&E, hematoxylin and eosin. |
Table 1
Animal Grouping of Various Conditions for Cell Passage Number, Dose, Toxicity, and Trafficking Analysis
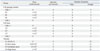
Table 2
Antibody Information for Immunohistochemical Analysis
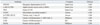
Table 3
Primer Sequences for Real-Time PCR
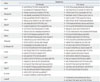
Table 4
Cell Toxicity Evaluation
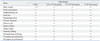
ACKNOWLEDGEMENTS
This research was supported by a grant of the Korea Health Technology R&D Project through the Korea Health Industry Development Institute (KHIDI), funded by the Ministry of Health & Welfare, Republic of Korea (HI14C1642).
References
2. Kim SO, Na HS, Kwon D, Joo SY, Kim HS, Ahn Y. Bone-marrow-derived mesenchymal stem cell transplantation enhances closing pressure and leak point pressure in a female urinary incontinence rat model. Urol Int. 2011; 86:110–116.


3. Kim BS, Chun SY, Lee JK, Lim HJ, Bae JS, Chung HY, et al. Human amniotic fluid stem cell injection therapy for urethral sphincter regeneration in an animal model. BMC Med. 2012; 10:94.


4. Chun SY, Cho DH, Chae SY, Choi KH, Lim HJ, Yoon GS, et al. Human amniotic fluid stem cell-derived muscle progenitor cell therapy for stress urinary incontinence. J Korean Med Sci. 2012; 27:1300–1307.


5. Kim H, Kim HY, Choi MR, Hwang S, Nam KH, Kim HC, et al. Dose-dependent efficacy of ALS-human mesenchymal stem cells transplantation into cisterna magna in SOD1-G93A ALS mice. Neurosci Lett. 2010; 468:190–194.


6. Morita E, Watanabe Y, Ishimoto M, Nakano T, Kitayama M, Yasui K, et al. A novel cell transplantation protocol and its application to an ALS mouse model. Exp Neurol. 2008; 213:431–438.


7. Banfi A, Muraglia A, Dozin B, Mastrogiacomo M, Cancedda R, Quarto R. Proliferation kinetics and differentiation potential of ex vivo expanded human bone marrow stromal cells: implications for their use in cell therapy. Exp Hematol. 2000; 28:707–715.


8. Bruder SP, Jaiswal N, Haynesworth SE. Growth kinetics, self-renewal, and the osteogenic potential of purified human mesenchymal stem cells during extensive subcultivation and following cryopreservation. J Cell Biochem. 1997; 64:278–294.


9. Uccelli A, Moretta L, Pistoia V. Mesenchymal stem cells in health and disease. Nat Rev Immunol. 2008; 8:726–736.


10. Rocha V, Labopin M, Gluckman E, Powles R, Arcese W, Bacigalupo A, et al. Relevance of bone marrow cell dose on allogeneic transplantation outcomes for patients with acute myeloid leukemia in first complete remission: results of a European survey. J Clin Oncol. 2002; 20:4324–4330.


11. Hohaus S, Wallwiener D, Martin S, Voso MT, Huober J, Fersis N, et al. Efficacy and toxicity of sequential high-dose therapy with peripheral blood stem cell support in patients with high-risk breast cancer. Semin Oncol. 1998; 25:2 Suppl 4. 7–11.
12. Plitnick LM, Herzyk DJ. Nonclinical development of novel biologics, biosimilars, vaccines and specialty biologics. Amsterdam: Academic Press;2013. p. 388–390.
13. De Coppi P, Bartsch G Jr, Siddiqui MM, Xu T, Santos CC, Perin L, et al. Isolation of amniotic stem cell lines with potential for therapy. Nat Biotechnol. 2007; 25:100–106.


14. Choi MR, Kim HY, Park JY, Lee TY, Baik CS, Chai YG, et al. Selection of optimal passage of bone marrow-derived mesenchymal stem cells for stem cell therapy in patients with amyotrophic lateral sclerosis. Neurosci Lett. 2010; 472:94–98.


15. Digirolamo CM, Stokes D, Colter D, Phinney DG, Class R, Prockop DJ. Propagation and senescence of human marrow stromal cells in culture: a simple colony-forming assay identifies samples with the greatest potential to propagate and differentiate. Br J Haematol. 1999; 107:275–281.


16. Haynesworth SE, Baber MA, Caplan AI. Cytokine expression by human marrow-derived mesenchymal progenitor cells in vitro: effects of dexamethasone and IL-1 alpha. J Cell Physiol. 1996; 166:585–592.


17. Liu CH, Hwang SM. Cytokine interactions in mesenchymal stem cells from cord blood. Cytokine. 2005; 32:270–279.


18. Faustman DL, Davis M. Stem cells in the spleen: therapeutic potential for Sjogren's syndrome, type I diabetes, and other disorders. Int J Biochem Cell Biol. 2010; 42:1576–1579.

