Abstract
Purpose
Recent evidence shows that nitric oxide (NO) may exhibit both pro-cancer and anti-cancer activities. The present study aimed to determine the differentially expressed proteins in NO-treated NIH/3T3 fibroblasts in order to investigate whether NO induces proteins with pro-cancer or anti-cancer effects.
Materials and Methods
The cells were treated with 300 µM of an NO donor 3,3-bis-(aminoethyl)-1-hydroxy-2-oxo-1-triazene (NOC-18) for 12 h. The changed protein patterns, which were separated by two-dimensional electrophoresis using pH gradients of 4-7, were conclusively identified by matrix-assisted laser desorption/ionization-time of flight mass spectrometry (MALDI-TOF MS) analysis of the peptide digests.
Results
Seventeen differentially expressed proteins were identified in NOC-18-treated cells. Nine proteins [vinculin protein, keratin 19, ubiquitous tropomodulin, F-actin capping protein (α1 subunit), tropomyosin 3, 26S proteasome-associated pad1 homolog, T-complex protein 1 (ε subunit) NG-dimethylarginine dimethylaminohydrolase, and heat shock protein 90] were increased and eight proteins (heat shock protein 70, glucosidase II, lamin B1, calreticulin, nucleophosmin 1, microtubule-associated protein retinitis pigmentosa/end binding family member 1, 150 kD oxygen-regulated protein precursor, and heat shock 70-related protein albino or pale green 2) were decreased by NOC-18 in the cells. Thirteen proteins are related to the suppression of cancer cell proliferation, invasion, and metastasis while two proteins (heat shock protein 90 and NG-dimethylarginine dimethylaminohydrolase) are related to carcinogenesis. The functions of 150 kD oxygen-regulated protein precursor and T-complex protein 1 (ε subunit) are unknown in relation to carcinogenesis.
Nitric oxide synthase (NOS) produces nitric oxide (NO) by catalyzing a five-electron oxidation of a guanidino nitrogen of L-arginine. Three different isoforms of the NOS family have been identified: endothelial NOS (eNOS), neuronal NOS (nNOS) and inducible NOS (iNOS). The nNOS and eNOS isoforms are constitutively expressed in a variety of cell types including endothelial cells, platelets, and neurons. These constitutive NOS isoforms are activated by calcium-calmodulin binding and produce low concentrations of NO.1 Unlike nNOS and eNOS, iNOS is an inducible and calcium-independent isoform. The expression of iNOS is induced by cytokines [e.g., interferon-γ, interleukin (IL)-1β, tumor necrosis factor-α], bacterial endotoxins, and oxidative stress.2 Once induced, iNOS continues to produce large amounts of NO for many hours or days.
NO is an important signaling molecule that is involved in many physiological and pathological processes. Under a normal physiological state, NO contributes to the regulation of anti-inflammatory and antioxidant effects.3 However, in tissues with high concentrations of NO, NO interacts with O2 or O2-, which results in the formation of reactive nitrogen species, which affect diverse processes, such as proliferation, apoptosis, differentiation, tumorigenesis, and metastasis. In human malignant tumors such as breast, lung, prostate, bladder, and colorectal cancer, the expression level of iNOS is high.4 The expression level and activity of iNOS are higher in most tumors than those in adjacent normal tissues. High levels of NO produced by iNOS in tumor tissues may modulate different cancer-related processes such as angiogenesis, apoptosis, invasion, and metastasis.5 In contrast, NO has tumoricidal effect by inhibiting proliferation and invasion of pancreatic cancer cells6 and inducing cell death in melanoma cells7 and microglial cells.8 Even though it is suggested that NO may exhibit tumoricidal activity by posttranslational modification of proteins, the precise functions of NO in cancer biology remain unclear.
An NO donor 3,3-bis-(aminoethyl)-1-hydroxy-2-oxo-1-triazene (NOC-18) is a stable NO-amine complex that can release NO spontaneously under physiological conditions. It suppresses DNA synthesis in human airway smooth muscle cells.9 In our previous study, NOC-18 activated nuclear factor κ-B (NF-κB) and activator protein-1 to induce IL-8 expression in gastric epithelial AGS cells.10 High amounts of NO induced DNA damage and apoptosis in gastric cancer cells, as well as chondrocytes in osteoarthritic cartilage.11,12 These studies suggest that an NO donor such as NOC-18 may have a growth inhibitory effect on cancer cells.
The present study aimed to determine the differentially expressed proteins in NO-treated NIH/3T3 fibroblasts in order to investigate whether NO induces proteins having pro-cancer or anti-cancer effects. The cells were treated with 300 µM of an NO donor (NOC-18) for 12 h. The changed protein patterns, which were separated by two-dimensional electrophoresis (2-DE), were conclusively identified by matrix-assisted laser desorption/ionization-time of flight mass spectrometry (MALDI-TOF MS) analysis of the peptide digests.
NIH/3T3 cells (mouse embryonic fibroblast, ATCC CRL 1658) were obtained from the American Type Culture Collection (Manassas, VA, USA) and cultured in Dulbecco's modified Eagle's medium supplemented with 10% fetal bovine serum (GIBCO-BRL, Grand Island, NY, USA) and antibiotics (100 U/mL penicillin and 100 µg/mL streptomycin). The cells were plated at a density of 2×106/mL in a 100-mm culture plate (Falcon 3047, Becton Dickinson Labware, Lincoln Park, NJ, USA), treated with NOC-18 (Sigma, St. Louis, MO, USA) at a concentration of 300 µM, and cultured for 12 h. The dose of the NO donor and duration of exposure time to the cells were adapted from our previous study, which showed that an NO donor induced NF-κB activation and inflammatory cytokine expression.10
Protein extraction, isoelectric focusing (IEF), and 2-DE separation were performed as described previously.13 Briefly, the cells were trypsinized, dissolved in 40 mM Tris buffer (pH 9.5), and passed ten times through a 22-gauge needle. Particulates were removed by centrifugation (15000×g, 15 min), and the supernatant was collected and diluted with extraction buffer. A 17-cm immobilized pH gradient (IPG) strip was used to absorb 300 µg of protein in 300 µL of extraction buffer, and this was electrophoresed on IEF cells. Following IEF, the IPG strips were subjected to equilibration for 15 min, re-equilibrated for 15 min, and separated using the Protean II xi cell gel SDS-PAGE system (Bio-Rad, Hercules, CA, USA) by molecular weight. Silver staining and image analyses were performed as described previously.13 Three batches of cell proteins extracted from non-treated cells and NO-donor-treated cells were subjected to 2-DE, and replicate gels were simultaneously run three times. The expression level was determined by the relative spot volume of each protein as compared to the total spot volume (sum of each protein spot) in the gel and expressed as a percentage of the volume. The differentially expressed proteins that had expression levels at least two times higher in NO-donor-treated cells than in non-treated cells were selected for the MALDI-TOF MS analysis. After in-gel digestion of proteins from silver stained gels, the peptide mixture was solubilized with 0.5% trifluoroacetic acid for MS analysis. MS was performed on a Micromass MALDI-TOF (Manchester, UK). Mass spectra were externally calibrated with autodigest peaks of trypsin (MH+: 906.505 Da, 1020.504 Da, 1153.574 Da, 2163.057 Da, 2273.160 Da). The peptide mass maps produced by MALDI-TOF MS were searched against the published databases using the MS-Fit module in Protein Prospector (http://prospector.ucsf.edu/prospector/mshome.htm) and Mascot (Marix Science, http://www.matrixscience.com). A mass tolerance of 50 ppm was used for peptide searches.
Fig. 1 shows the representation of the three separate experiments. The silver-stained gels were scanned with the GS 690 Imaging Densitometer at a 400-ppi grayscale level. After spot detection, background subtraction, and volume normalization, the differentially expressed proteins were detected in NOC-18-treated cells (NOC-18) more than the non-treated cells (none).
Differentially expressed proteins between non-treated cells and NOC-18-treated cells were excised from 2-DE gels and identified using peptide mass fingerprinting. A mascot search using the peptide mass fingerprinting data indicated that certain proteins [vinculin protein, keratin 19, ubiquitous tropomodulin, F-actin capping protein (α1 subunit), tropomyosin 3, 26S proteasome-associated pad1 homolog, T-complex protein 1 (ε subunit), NG-dimethylarginine dimethylaminohydrolase, and heat shock protein 90 (HSP90)] were increased (Fig. 2, Table 1), and eight proteins [heat shock protein 70 (HSP70), glucosidase II, lamin B1, calreticulin, nucleophosmin 1, microtubule-associated protein retinitis pigmentosa/end binding (RP/EB) family member 1, 150 kD oxygen-regulated protein precursor, and heat shock 70-related protein albino or pale green 2 (APG-2)] were decreased (Fig. 3, Table 2) by the treatment of NOC-18 in the cells.
The expression of HSP70 decreased in NOC-18-treated cells compared to non-treated cells in the present study. HSP70 is overexpressed in human tumors of various origins, such as breast, lung, colorectal, and cervical carcinomas as well as osteosarcoma.14 Its expression correlates with increased proliferation, poor differentiation, lymph node metastasis, and poor therapeutic outcome in human breast cancer.15 Several studies demonstrate that HSP70 promotes tumorigenesis through inhibiting lysosomal membrane permeabilization, activation, and translocation of pro-apoptotic factors such as Jun kinase, Bid, Bax, apoptosis-inducing factors, and the release of cytochrome c from mitochondria.15,16 Therefore, the decreased expression level of HSP70 by NOC-18 implies that a high amount of NO may suppress the proliferation of cancer cells. In contrast, HSP90 was increased by NOC-18 treatment in the present study. A high expression of HSP90 promotes stability and function of several oncogenic client protein kinases such as human epidermal growth factor receptor 2 and epidermal growth factor receptor in tumors.17,18 These HSP90-client proteins play crucial roles in establishing cancer cell hallmarks. One recent study suggests that dual targeting of HSP90 and HSP70 promotes cell death and enhances the anticancer effect of chemotherapeutic agents in bladder cancer.19 Therefore, increased expression of HSP90 may induce carcinogenesis. Differential expression levels of HSP70 and HSP90 by NOC-18 treatment may be explained by their roles as molecular chaperones that regulate protein folding and respond to cellular stress.20 More studies are required to determine the effect of NO donors on the expression of HSPs in relation to cancer cell proliferation and invasion.
Endoplasmic reticulum (ER)-chaperone calreticulin was decreased by the treatment of an NO donor in the present study. Calreticulin resides in ER and functions as a molecular chaperone.21 Calreticulin is overexpressed in bladder cancer, prostatic adenocarcinoma, hepatocellular carcinoma, pancreatic cancer, gastric cancer, colon cancer, melanoma, and leukemia.21 Overexpression of calreticulin is known to be associated with invasive and advanced malignant processes as well as poor prognoses. Calreticulin knockdown suppressed proliferation, migration, and attachment of cancer cells.22 Therefore, the down-regulation of ER-chaperone calreticulin by NOC-18 in the present study suggests that high levels of NO may suppress the properties of cancer cells including proliferation, invasion, and metastasis.
Present results show that microtubule-associated protein RP/EB family member 1 was decreased by treatment of NOC-18. Microtubule-associated proteins bind to tubulin subunits that make up microtubules to regulate their stability. Microtubule-associated proteins are known to be interacting partners of adenomatous polyposis coli (APC), enhancing APC function in colorectal cancer.23 Microtubule-associated proteins have been reported to be overexpressed in gastric adenocarcinoma, hepatocellular carcinoma, esophageal squamous-cell carcinoma, and breast cancer.24 Its overexpression is known to be involved in tumorigenesis and the promotion of tumor cell growth via the Wnt signaling pathway or Aurora-B activation.25 Down-regulation of microtubule-associated proteins induced apoptosis of cancer cells through the mitochondrial death pathway.26 Therefore, decreased expression of microtubule-associated proteins by an NO donor implies that high amounts of NO may inhibit cancer development.
Lamin B1 functions in nuclear envelope lamina and possesses a transcriptional co-regulatory activity that has an important role in DNA replication, cellular aging, and stress responses. Overexpression of lamin B1 in cancer cells is associated with low differentiation, increased metastasis, and poor prognosis of pancreatic cancer patients.27 Silencing lamin B1 inhibits the proliferation and invasion of pancreatic cancer cells.28 In our study, we found that the expression of lamin B1 was decreased by NOC-18. Therefore, the decreased expression of lamin B1 by NOC-18 suggests that the treatment of NO donors may inhibit cancer progression, including proliferation and metastasis.
In the present study, we found that expressions of glucosidase II and nucleophosmin 1 were decreased by NOC-18. Glucosidase II plays a central role in the processing of nascent glycoproteins in ER lumina by removing certain glucose units from core oligosaccharide moieties. Suradej, et al.29 showed that glucosidase II is overexpressed in human lung tumor tissues and exhibits a stress response similar to p53, indicating that it may have a crucial role in lung tumorigenesis. Nucleophosmin 1 is an estrogen-regulated nuclear protein. The expression of nucleophosmin 1 is correlated with lymph node metastasis and a poor survival rate of human colon cancer patients.30 Down-regulation of nucleophosmin 1 using siRNA reduced cell viability and caused a concomitant increase in cellular senescence and cell cycle arrest for colon cancer.30 These studies demonstrate that expressions of glucosidase II and nucleophosmin 1 are associated with growth, invasion, and metastasis of cancer cells. Therefore, treatment of NO donors may inhibit carcinogenesis by down-regulating glucosidase II and nucleophosmin 1.
150 kD oxygen-regulated protein (ORP150) precursor was also decreased by NOC-18 treatment. ORP150 takes part in the process of endoplasmic reticulum stress.31 Even though the role of ORP150 has not been identified in cancer, it may affect cancer cell proliferation through the pathway of endoplasmic reticulum stress.
APG-2, a mammalian heat shock protein belonging to the heat shock protein 110 (HSP110) family, was previously found to be overexpressed in BaF3-BCR/ABL cells [break point cluster region-abelson (BCR-ABL) transfected murine pro-B Ba/F3 cells].32 Overexpression of APG-2 in BaF3-BCR/ABL cells increases cell proliferation and protects cells from oxidative damage,32 which may play an important role in carcinogenesis. APG-2 was shown to have a chaperone ability similar to HSP110 and an anti-apoptotic role in hepatic cancer cells.33 These studies suggest that the HSP110 family members play important roles in hepatocarcinogenesis and leukemia development through their chaperoning activities. NOC-18-induced decrease in APG-2 suggests that NO donors have a beneficial role in treating various types of cancer by suppressing proliferation.
NOC-18 induced the expression of vinculin (VCL) in the present study. VCL is an adapter protein with binding sites for more than 15 proteins and plays a critical role in the linkage of integrin adhesion molecules to the cytoskeleton.34 VCL functions as a tumor suppressor by increasing anchorage-dependent cell growth and by suppressing tumor metastasis through reducing cell motility.34,35
The expressions of ubiquitous tropomodulins, tropomyosin 3, and F-actin capping protein α1 subunit (CAPZA1) were increased by NOC-18 in the present study. Tropomodulins are proteins that cap the slow-growing end of actin filaments and require tropomyosin for optimal function.36 Franzén, et al.37 found that tropomyosin 3 was down-regulated in fibroblasts transformed by oncogenes. Zheng, et al.38 reported that overexpression of tropomyosin 3 suppressed invasion and metastasis of cancer cells. F-actin capping protein binds to fast growing ends of actin filaments. CAPZA1 is known to be upregulated in gastric cancer tissues when compared to normal tissues.39 Overexpression of CAPZA1 suppresses the migration and invasion of gastric cancer cells.40 Therefore, NO donor-induced upregulation of tropomodulins, tropomyosin 3, and CAPZA1 suggests a potential role of NO donors for suppressing the migration and invasion of cancer cells.
Keratins are intermediate filament proteins responsible for the structural integrity of epithelial cells. In this study, we found that NOC-18 increased the expression of keratin 19. Schoenfeld, et al.41 reported that keratin 19 is overexpressed in tumor cells which are disseminated in the lymph nodes, peripheral blood, and bone marrow of breast cancer patients. Keratin 19 acts as a potential tumor suppressor that inhibits proliferation, invasion, and metastasis in breast cancer.42 Therefore, NO donors may inhibit carcinogenesis through up-regulation of keratin 19.
Another protein increased by NOC-18 treatment was T-complex protein 1 (TCP-1), ε subunit. TCP is a member of the chaperonin-containing TCP-1 complex (CCT).43 CCT is involved in the folding of cytoskeleton proteins and other proteins such as cyclin E1, histone deacetylase, and protein phosphatase 2A regulatory subunit B.43 Even though the role of the ε subunit of TCP-1 is unknown, it may act as a stress-sensitive chaperone by holding actin and tubulin in the cytosol.44
The 26S proteasome is the main nonlysosomal protease in eukaryotic cells. Head and neck small cancer cells with low proteasome activity showed a significantly higher self-renewal capacity and increased tumorigenicity.45 Therefore, NO donor-induced expression of 26S-proteasome-associated pad1 homolog may reduce the self-renewing capacity of cancer cells. Further researched should be performed to determine the role of 26S proteasome activity and its related proteins on carcinogenesis.
NG-dimethylarginine dimethylaminohydrolase (DDAH) metabolizes the endogenous inhibitor of NO synthesis, asymmetric dimethylarginine. Constitutive overexpression of DDAH1, the isoform primarily associated with nNOS, results in increased tumor growth and vascularization, as well as elevated secretion of vascular endothelial growth factor.46 Induction of DDAH by NOC-18 treatment is controversial compared to the induction of other proteins related to cancer suppression. Further studies are required in order to relate DDAH to NO treatment.
In the present study, 17 differentially expressed proteins were identified after the treatment of NO donor NOC-18 (Table 3). Among them, 13 proteins are related to the suppression of cancer cell proliferation, invasion, and metastasis while two proteins (HSP90, DDAH) are related to carcinogenesis. The functions of ORP150 and TCP-1, ε subunit, have not been known to be related to cancer development. Even though most proteins differentially expressed by NOC-18 are involved in inhibiting cancer development, further study should be performed to determine anti-cancer effects of NO donors, using experimental animal models with various types of cancers.
Figures and Tables
Fig. 1
2-DE gel map derived from non-treated cells (none) and NOC-18-treated cells (NOC-18). (A) Increased proteins in non-treated cells (none) compared to NOC-18-treated cells (NOC-18) in a 12-h culture. (B) Increased proteins in NOC-18-treated cells (NOC-18) compared to non-treated cells (none) in a 12-h culture. NOC-18, NO donor 3,3-bis-(aminoethyl)-1-hydroxy-2-oxo-1-triazene; 2-DE, two-dimensional electrophoresis; SDS-PAGE, sodium dodecyl sulphate-polyacrylamide gel electrophoresis; APG, albino or pale green; RP/EB, retinitis pigmentosa/end binding.
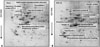
Fig. 2
Segments of a 2-DE gel map derived from non-treated cells (none) and NOC-18-treated cells (NOC-18). Arrowed spots are the proteins that increased in NOC-18-treated cells when compared to non-treated cells (none) in a 12-h culture. Protein expression level is expressed as a percentage of the volume, which is determined by the relative spot volume of each protein compared to the total spot volume (total amount of proteins/sum of each protein spot) in the gel. NOC-18, NO donor 3,3-bis-(aminoethyl)-1-hydroxy-2-oxo-1-triazene; 2-DE, two-dimensional electrophoresis.
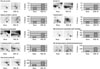
Fig. 3
Segments of a 2-DE gel map derived from non-treated cells (none) and NOC-18-treated cells (NOC-18). Arrowed spots are the proteins that decreased in NOC-18-treated cells when compared to non-treated cells (none) in a 12-h culture. Protein expression level is expressed as a percentage of the volume, which is determined by the relative spot volume of each protein compared to the total spot volume (total amount of proteins/sum of each protein spot) in the gel. NOC-18, NO donor 3,3-bis-(aminoethyl)-1-hydroxy-2-oxo-1-triazene; 2-DE, two-dimensional electrophoresis.

Table 1
Increased Proteins in NOC-18-Treated Cells Compared to Non-Treated Cells in a 12-h Culture, Identified Using MALDI-TOF MS
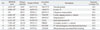
Table 2
Decreased Proteins in NOC-18-Treated Cells Compared to Non-Treated Cells in a 12-h Culture, Identified Using MALDI-TOF MS
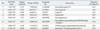
Table 3
Protein Expression Level in the Gel
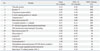
NOC-18, NO donor 3,3-bis-(aminoethyl)-1-hydroxy-2-oxo-1-triazene; RP/EB, retinitis pigmentosa/end binding; APG, albino or pale green.
The expression level was determined by the relative spot volume of each protein as compared to the total spot volume (total amount of proteins/sum of each protein spot) in the gel and expressed as a percentage of the volume.
ACKNOWLEDGEMENTS
This work was supported by a grant from the NRF of Korea, which is funded by the Korean government (MSIP) (2007-0056092).
References
1. Moncada S, Palmer RM, Higgs EA. Nitric oxide: physiology, pathophysiology, and pharmacology. Pharmacol Rev. 1991; 43:109–142.
2. Beck KF, Eberhardt W, Frank S, Huwiler A, Messmer UK, Mühl H, et al. Inducible NO synthase: role in cellular signalling. J Exp Biol. 1999; 202(Pt 6):645–653.


3. Kanner J, Harel S, Granit R. Nitric oxide as an antioxidant. Arch Biochem Biophys. 1991; 289:130–136.


4. Lechner M, Lirk P, Rieder J. Inducible nitric oxide synthase (iNOS) in tumor biology: the two sides of the same coin. Semin Cancer Biol. 2005; 15:277–289.


5. Ying L, Hofseth LJ. An emerging role for endothelial nitric oxide synthase in chronic inflammation and cancer. Cancer Res. 2007; 67:1407–1410.


6. Sugita H, Kaneki M, Furuhashi S, Hirota M, Takamori H, Baba H. Nitric oxide inhibits the proliferation and invasion of pancreatic cancer cells through degradation of insulin receptor substrate-1 protein. Mol Cancer Res. 2010; 8:1152–1163.


7. Carneiro ZA, Biazzotto JC, Alexiou AD, Nikolaou S. Nitric oxide photorelease from a trinuclear ruthenium nitrosyl complex and its in vitro cytotoxicity against melanoma cells. J Inorg Biochem. 2014; 134:36–38.


8. Brantley EC, Guo L, Zhang C, Lin Q, Yokoi K, Langley RR, et al. Nitric oxide-mediated tumoricidal activity of murine microglial cells. Transl Oncol. 2010; 3:380–388.


9. Patel HJ, Belvisi MG, Donnelly LE, Yacoub MH, Chung KF, Mitchell JA. Constitutive expressions of type I NOS in human airway smooth muscle cells: evidence for an antiproliferative role. FASEB J. 1999; 13:1810–1816.


10. Seo JY, Yu JH, Lim JW, Mukaida N, Kim H. Nitric oxide-induced IL-8 expression is mediated by NF-kappaB and AP-1 in gastric epithelial AGS cells. J Physiol Pharmacol. 2009; 60:Suppl 7. 101–106.
11. Lim JW, Kim H, Kim KH. NF-kappaB, inducible nitric oxide synthase and apoptosis by Helicobacter pylori infection. Free Radic Biol Med. 2001; 31:355–366.


12. Davies CM, Guilak F, Weinberg JB, Fermor B. Reactive nitrogen and oxygen species in interleukin-1-mediated DNA damage associated with osteoarthritis. Osteoarthritis Cartilage. 2008; 16:624–630.


13. Yu JH, Yun SY, Lim JW, Kim H, Kim KH. Proteome analysis of rat pancreatic acinar cells: implication for cerulein-induced acute pancreatitis. Proteomics. 2003; 3:2446–2453.


14. Faure O, Graff-Dubois S, Bretaudeau L, Derré L, Gross DA, Alves PM, et al. Inducible Hsp70 as target of anticancer immunotherapy: Identification of HLA-A*0201-restricted epitopes. Int J Cancer. 2004; 108:863–870.


15. Gabai VL, Yaglom JA, Waldman T, Sherman MY. Heat shock protein Hsp72 controls oncogene-induced senescence pathways in cancer cells. Mol Cell Biol. 2009; 29:559–569.


16. Zhao Q, Wang J, Levichkin IV, Stasinopoulos S, Ryan MT, Hoogenraad NJ. A mitochondrial specific stress response in mammalian cells. EMBO J. 2002; 21:4411–4419.


17. Sidera K, Gaitanou M, Stellas D, Matsas R, Patsavoudi E. A critical role for HSP90 in cancer cell invasion involves interaction with the extracellular domain of HER-2. J Biol Chem. 2008; 283:2031–2041.


18. Miyata Y, Nakamoto H, Neckers L. The therapeutic target Hsp90 and cancer hallmarks. Curr Pharm Des. 2013; 19:347–365.


19. Ma L, Sato F, Sato R, Matsubara T, Hirai K, Yamasaki M, et al. Dual targeting of heat shock proteins 90 and 70 promotes cell death and enhances the anticancer effect of chemotherapeutic agents in bladder cancer. Oncol Rep. 2014; 31:2482–2492.


20. Guttmann DM, Koumenis C. The heat shock proteins as targets for radiosensitization and chemosensitization in cancer. Cancer Biol Ther. 2011; 12:1023–1031.


21. Michalak M, Robert Parker JM, Opas M. Ca2+ signaling and calcium binding chaperones of the endoplasmic reticulum. Cell Calcium. 2002; 32:269–278.


22. Hisaoka M, Matsuyama A, Nakamoto M. Aberrant calreticulin expression is involved in the dedifferentiation of dedifferentiated liposarcoma. Am J Pathol. 2012; 180:2076–2083.


23. Pfister AS, Hadjihannas MV, Röhrig W, Schambony A, Behrens J. Amer2 protein interacts with EB1 protein and adenomatous polyposis coli (APC) and controls microtubule stability and cell migration. J Biol Chem. 2012; 287:35333–35340.


24. Tamura N, Draviam VM. Microtubule plus-ends within a mitotic cell are 'moving platforms' with anchoring, signalling and force-coupling roles. Open Biol. 2012; 2:120132.


26. Kim MJ, Yun HS, Hong EH, Lee SJ, Baek JH, Lee CW, et al. Depletion of end-binding protein 1 (EB1) promotes apoptosis of human non-small-cell lung cancer cells via reactive oxygen species and Bax-mediated mitochondrial dysfunction. Cancer Lett. 2013; 339:15–24.


27. Sun S, Xu MZ, Poon RT, Day PJ, Luk JM. Circulating Lamin B1 (LMNB1) biomarker detects early stages of liver cancer in patients. J Proteome Res. 2010; 9:70–78.


28. Li L, Du Y, Kong X, Li Z, Jia Z, Cui J, et al. Lamin B1 is a novel therapeutic target of betulinic acid in pancreatic cancer. Clin Cancer Res. 2013; 19:4651–4661.


29. Suradej B, Pata S, Kasinrerk W, Cressey R. Glucosidase II exhibits similarity to the p53 tumor suppressor in regards to structure and behavior in response to stress signals: a potential novel cancer biomarker. Oncol Rep. 2013; 30:2511–2519.


30. Wong JC, Hasan MR, Rahman M, Yu AC, Chan SK, Schaeffer DF, et al. Nucleophosmin 1, upregulated in adenomas and cancers of the colon, inhibits p53-mediated cellular senescence. Int J Cancer. 2013; 133:1567–1577.


31. Deng WH, Chen C, Wang WX, Yu J, Li JY, Liu L. Effects of ORP150 on appearance and function of pancreatic beta cells following acute necrotizing pancreatitis. Pathol Res Pract. 2011; 207:370–376.


32. Li C, Liu D, Yuan Y, Huang S, Shi M, Tao K, et al. Overexpression of Apg-2 increases cell proliferation and protects from oxidative damage in BaF3-BCR/ABL cells. Int J Oncol. 2010; 36:899–904.


33. Gotoh K, Nonoguchi K, Higashitsuji H, Kaneko Y, Sakurai T, Sumitomo Y, et al. Apg-2 has a chaperone-like activity similar to Hsp110 and is overexpressed in hepatocellular carcinomas. FEBS Lett. 2004; 560:19–24.


34. Carisey A, Ballestrem C. Vinculin, an adapter protein in control of cell adhesion signalling. Eur J Cell Biol. 2011; 90:157–163.


35. Goldmann WH, Auernheimer V, Thievessen I, Fabry B. Vinculin, cell mechanics and tumour cell invasion. Cell Biol Int. 2013; 37:397–405.


36. Kostyukova AS. Tropomodulins and tropomodulin/tropomyosin interactions. Cell Mol Life Sci. 2008; 65:563–569.


37. Franzén B, Linder S, Uryu K, Alaiya AA, Hirano T, Kato H, et al. Expression of tropomyosin isoforms in benign and malignant human breast lesions. Br J Cancer. 1996; 73:909–913.


38. Zheng Q, Safina A, Bakin AV. Role of high-molecular weight tropomyosins in TGF-beta-mediated control of cell motility. Int J Cancer. 2008; 122:78–90.


39. Lim BH, Cho BI, Kim YN, Kim JW, Park ST, Lee CW. Overexpression of nicotinamide N-methyltransferase in gastric cancer tissues and its potential post-translational modification. Exp Mol Med. 2006; 38:455–465.


40. Lee YJ, Jeong SH, Hong SC, Cho BI, Ha WS, Park ST, et al. Prognostic value of CAPZA1 overexpression in gastric cancer. Int J Oncol. 2013; 42:1569–1577.


41. Schoenfeld A, Luqmani Y, Sinnett HD, Shousha S, Coombes RC. Keratin 19 mRNA measurement to detect micrometastases in lymph nodes in breast cancer patients. Br J Cancer. 1996; 74:1639–1642.


42. Ju JH, Yang W, Lee KM, Oh S, Nam K, Shim S, et al. Regulation of cell proliferation and migration by keratin19-induced nuclear import of early growth response-1 in breast cancer cells. Clin Cancer Res. 2013; 19:4335–4346.


43. Guenther MG, Yu J, Kao GD, Yen TJ, Lazar MA. Assembly of the SMRT-histone deacetylase 3 repression complex requires the TCP-1 ring complex. Genes Dev. 2002; 16:3130–3135.


44. Nikawa J, Kimura M. A novel function of the human chaperonin CCT epsilon subunit in yeast. Biosci Biotechnol Biochem. 2012; 76:199–201.

