Abstract
Purpose
The genus Aeromonas is a pathogen that is well known to cause severe clinical illnesses, ranging from gastroenteritis to sepsis. Accurate identification of A. hydrophila, A. caviae, and A. veronii is important for the care of patients. However, species identification remains difficult using conventional methods. The aim of this study was to compare the accuracy of different methods of identifying Aeromonas at the species level: a biochemical method, matrix-assisted laser desorption ionization mass spectrometry-time of flight (MALDI-TOF MS), 16S rRNA sequencing, and housekeeping gene sequencing (gyrB, rpoB).
Materials and Methods
We analyzed 65 Aeromonas isolates recovered from patients at a university hospital in Korea between 1996 and 2012. The isolates were recovered from frozen states and tested using the following four methods: a conventional biochemical method, 16S rRNA sequencing, housekeeping gene sequencing with phylogenetic analysis, and MALDI-TOF MS.
Results
The conventional biochemical method and 16S rRNA sequencing identified Aeromonas at the genus level very accurately, although species level identification was unsatisfactory. MALDI-TOF MS system correctly identified 60 (92.3%) isolates at the species level and an additional four (6.2%) at the genus level. Overall, housekeeping gene sequencing with phylogenetic analysis was found to be the most accurate in identifying Aeromonas at the species level.
Conclusion
The most accurate method of identification of Aeromonas to species level is by housekeeping gene sequencing, although high cost and technical difficulty hinder its usage in clinical settings. An easy-to-use identification method is needed for clinical laboratories, for which MALDI-TOF MS could be a strong candidate.
The genus Aeromonas is a Gram-negative facultative anaerobe and is a ubiquitous inhabitant of aquatic ecosystems. Aeromonas is known to cause human infections, ranging from hepatobiliary tract infections, soft tissue infections, and diarrhea to septicemia in immunocompromised hosts.1 There are currently 24 valid Aeromonas species reported in the literature.2 Among them Aeromonas hydrophila, A. caviae, and A. veronii biovar sobria are described as the most important human pathogens.1 Accurate identification of Aeromonas at the species level can be difficult due to limitations in the accuracy of currently available methods.3 DNA-DNA hybridization is known as the gold standard for identification of Aeromonas,2 although it is not widely used in clinical laboratories due to its high cost and time required for analysis. Similar problems have been reported in sequencing of 16S rRNA and housekeeping genes, such as gyrB, dnaJ, and rpoD.2,4,5 On the contrary, matrix-assisted laser desorption ionization mass spectrometry-time of flight (MALDI-TOF MS) has recently been suggested as a new alternative to conventional method of identification due to its favorable speed and application.3,6,7
This study was performed to compare the accuracy and applicability of different methods of identifying Aeromonas at the species level: a conventional biochemical method, MALDI-TOF MS, 16S rRNA sequencing, and housekeeping gene sequencing (gyrB, rpoB).
A total of 65 cases of Aeromonas were recovered from various clinical specimens, including blood, body fluid, stool, and so on, among patients treated at a university hospital between 1996 and 2012. Isolates were identified upon isolation by conventional phenotypic methods, using the VITEK Sr-60, mini-ATB, and VITEK 2 (bioMérieux, Marcy l'Etoile, France) systems. Isolates were kept frozen at -80℃ until further identification was performed.
Bacterial genomic DNA samples were extracted using an InstaGenetm Matrix BIO-RAD (Hercules, CA, USA). The primers used for the PCR are summarized in Table 1. The PCR reaction was performed with 20 ng of genomic DNA as a template in a 30 µL reaction mixture by using EF-Taq (SolGent, Korea) for the following cycles: activation of Taq polymerase at 95℃ for 2 minutes, followed by 35 cycles at 95℃, 55℃, and 72℃ for 1 minute each, finishing with a 10-minute step at 72℃.
The amplification products were purified with a multiscreen filter plate (Millipore Corps., Billerica, MA, USA). Sequencing reaction was performed using a PRISM BigDye Terminator v3.1 Cycle sequencing kit (Applied Biosystems, Foster City, CA, USA). The DNA samples containing the extension products were added to Hi-Di formamide (Applied Biosystems, Foster City, CA, USA). The mixture was incubated at 95℃ for 5 minutes, followed by 5 minutes on ice, and then analyzed by an ABI Prism 3730XL DNA analyzer (Applied Biosystems, Foster City, CA, USA). 16S rRNA sequencing was performed in Macrogen (Seoul, Korea).
gyrB sequencing primers were designed after Yáñez, et al.8 (Table 1). The PCR reaction for gyrB sequencing was performed with 20 ng of genomic DNA as a template in a 30 µL reaction mixture using HF-Taq (Macrogen, Seoul, Korea). The PCR conditions were as follows: activation of Taq polymerase at 95℃ for 2 minutes, 35 cycles of 94℃ for 30 seconds, 55℃ for 30 seconds, and 72℃ for 1 minute, finishing with a 10-minute step at 72℃.
The amplification products were purified with a multiscreen filter plate (Millipore Corp., MA, USA). Sequencing reaction was performed using a PRISM BigDye Terminator v3.1 Cycle sequencing kit. The DNA samples containing the extension products were added to Hi-Di formamide (Applied Biosystems, CA, USA). The mixture was incubated at 95℃ for 5 minutes, followed by 5 minutes on ice, and then analyzed by an ABI Prism 3730XL DNA analyzer (Applied Biosystems, CA, USA).
The rpoB primer sets were designed after Korczak, et al.9 (Table 1). The PCR reaction was performed with 20 ng of genomic DNA as a template in a 30 µL reaction mixture using HF-Taq (Macrogen, Seoul, Korea); activation of Taq polymerase at 94℃ for 3 minutes, 35 cycles of 94℃ for 30 seconds, 54℃ for 30 seconds, and 72℃ for 30 seconds, finishing with a 7 minute step at 72℃. The amplification products were purified with a multiscreen filter plate (Millipore Corp., MA, USA). Sequencing reaction was performed using a PRISM BigDye Terminator v3.1 Cycle sequencing kit. The DNA samples containing the extension products were added to Hi-Di formamide (Applied Biosystems, CA, USA). The mixture was incubated at 95℃ for 5 minutes, followed by 5 minutes on ice, and then analyzed by an ABI Prism 3730XL DNA analyzer (Applied Biosystems, CA, USA).
After sequencing, we processed the raw sequence file (.abi) using Lasergene SeqMan pro 7.1 (DNASTAR, Madison, WI, USA) to obtain a trimmed nucleotide sequence. A phylogenetic tree was generated using the neighbor-joining method based on nucleotide sequences of clinical isolates with reference strain sequences prepared from the NCBI database (http://www.ncbi.nlm.nih.gov/) using Mega 5.0 software.10
gyrB and rpoB gene sequencing were performed by Macrogen (Seoul, Korea).
Reference identification was done by summing up commercial phenotypic methods with 16S rRNA sequencing results. However, if the phenotypic systems failed to identify the species or in the case of discordant results, when compared to the 16S rRNA sequencing, we compared two housekeeping gene sequences (gyrB, rpoB) with phylogenetic analysis to see whether the results matched.
Thawed clinical isolates were plated on blood agar plates and after 24 hours of incubation at 37℃, a subculture was made to another blood agar plate (Asan Pharmaceutical, Seoul, Korea). The cells were extracted using a formic acid/acetonitrile method, as described previously.11 One microliter of each extract was spotted onto a sample target, overlaid with an HCCA (acyano-4-hydroxycinnamic acid) matrix solution, and loaded into BIOTYPER 2.0 (Bruker Daltonics, Bremen, Germany).
The obtained spectra profiles were compared with a database (V2.0.4.0) containing reference spectra of roughly 1900 microbial species. The peak intensities for each isolate were associated with the respective classes. Based on these mass classes, a similarity matrix was calculated to compare the results of the MALDI-TOF MS with the results of the other identification methods
Among the 65 isolates tested, 35 isolates of A. hydrophila, 23 isolates of A. caviae, six isolates of A. veronii and an A. aquariorum were identified to the species level (Table 2).
Conventional biochemical method and 16S rRNA sequencing showed poor correlation with housekeeping gene sequencing test. Conventional biochemical method and 16S rRNA sequencing correctly identified 31 (47.7%) and 28 (43.1%) isolates at the species level, as well as an additional 34 (52.3%) and 36 (55.4%) isolates at the genus level, respectively.
The MALDI-TOF MS system correlated well with the reference identification, showing 92.3% coincidence at the species level. All A. hydrophila and A. veronii strains identified by MALDI-TOF MS were fully compatible with the housekeeping gene results, although a case of A. aquariorum was identified as A. hydrophila. Otherwise, three cases of A. caviae were identified as A. hydrophila and there was one case that had no peak value.
Aeromonas have been reported to cause a wide range of clinically significant problems, such as hepatobiliary tract infections, soft tissue infection, diarrhea, and septicemia.2,12,13 Tho-ugh there are many Aeromonas species, only a few species, A. hydrophila, A. caviae, and A. veronii biovar sobria, have been unquestionably established as human pathogens. Therefore, identification of Aeromonas to species level has clinical significance.14
Several methods have been developed for identification of Aeromonas, which include biochemical methods, 16S rRNA gene sequencing, and multiple molecular markers sequencing; among these, DNA-DNA hybridization remains as the gold standard.15 Nevertheless, the cost and complexity of the DNA-DNA hybridization method has hindered its use in clinical laboratories. Identification of Aeromonas is difficult by biochemical methods, as it can be easily confused with other oxidase-positive fermenters (Vibrio and Plesiomonas).16 16S rRNA gene sequencing has been widely adapted for identification of bacteria; however, the genus Aeromonas comprises very similar genetic groups, with species differing only by a few nucleotides, making them difficult to identify.17 MALDI-TOF looks promising as an identification tool of Aeromonas, although it still shows difficulties in identifying some organisms, such as viridans group streptococci, Shigella, and Aeromonas spp.18
In this study, we compared two housekeeping gene sequences (gyrB, rpoB), as two or more housekeeping genes sequencing could be useful to improve the reliability of the phylogenies. The results matched in every isolate, except isolate16, which was compatible with A. caviae in gyrB and with A. hydrophila in rpoB. To resolve the discrepancy, we referred to phylogenetic analysis and the isolate was concluded to be A. caviae (Figs. 1 and 2). This result coincides with other studies that emphasize the usefulness of multiple housekeeping gene sequencing.5,15 As demonstrated in the present phylogenetic analysis, the use of several housekeeping genes is an invaluable approach for the classification of Aeromonas species and for the proper identification of novel, closely related isolates. The resolution of the 16S rRNA gene sequence to split species has been displaced by the more variable sequences of the gyrB and rpoD genes.19
In our case, biochemical identification of Aeromonas proved not satisfactory as a species-level discriminator (Table 2). This could have resulted from the considerable phenotypic diversity within the Aeromonas species.20
16S rRNA sequencing has been widely used to identify clinical isolates in modern laboratories. However, as demonstrated in this study, as well as in several other studies, 16S rRNA sequencing has limitations in differentiating between species, including A. aquariourm and A. caviae, as well as A. hydrophila and A. caviae.5 The lack of an ability to differentiate between these Aeromonas species can be explained by the fact that 16S rRNA gene sequences are less divergent than other housekeeping genes (gyrB, rpoD, and so on), and furthermore, some species may have several copies of small subunits of ribosomal RNA at the same time, causing intragenomic heterogeneity of small subunits.2,21
Recently, MALDI-TOF MS has been rapidly introduced to clinical laboratories. Many published articles support its use in identification of Aeromonas at the species level.3,6,22 In our study, MALDI-TOF MS showed very good correlation with the results of housekeeping gene sequencing, especially for A. hydrophila and A. veronii strains. Considering that there is still a debate about the novelty of A. aquariorum,23 discrepancies in MALDI-TOF MS results were virtually confined to A. caviae. In this study, three cases of A. caviae were identified as A. hydrophila and a case of A. caviae gave rise to an "unidentified" result. Therefore, we concluded that MALDI-TOF MS could differentiate Aeromonas at the species level, while genus-level differentiation may show some discrepancies, as shown in Table 2.
We summarized various test results (phylogenetic grouping, 16S sequencing) for the verification of the housekeeping gene sequencing and the results were not changed. Microbial identification by MALDI-TOF MS is based on the assessment of proteins, and some environmental strains could exhibit unique strain-differentiating biomarkers not supported in manufacturers' databases.24 An "unidentified" result could not be explained clearly, but considering that repeated tests gave the same result, interactions between matrices and the organism or lack of a library of mass spectral fingerprints for identification could be considered as a cause. By using MALDI-TOF MS, Donohue, et al.22 reported a successful identification rate of 93%, with only 7% identification failure. This value coincides with our rates of 92.3% and 7.7%, respectively (Table 2). There is another application of MALDI-TOF MS, by which using the signals generated from the analysis of the protein masses as a biomarkers for the differentiation below the species level. We plan to study this next to extend the application of MALDI-TOF MS in the field of Aeromonas species identification.
We found a case that was initially reported as A. hydrophila but re-identified as A. aquariorum by a molecular method. A. aquariorum is rarely reported in Korea and there is just one recent case report.25 This discrepancy in the results is obviously a concern and it once again accentuates the need for the development of more accurate methods of identifying Aeromonas at the species level, particularly when there is a report to suggest the presence of important subset of virulence genes in A. aquariorum.26
Though the accuracy of MALDI-TOF MS in detecting Aeromonas at the species levels was not perfect in our study, we feel that MALDI-TOF MS has several advantages over many other methods in terms of its rapidity and low cost. Further study is required to analyze the reason for the lack of accuracy in differentiation of Aeromonas species in our study and also to better establish its applicability in routine clinical settings.
Figures and Tables
Fig. 1
Unrooted neighbor-joining phylogenetic trees based on gyrB, showing relationships in the genus Aeromonas. Type strains are included.
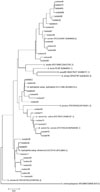
Fig. 2
Unrooted neighbor-joining phylogenetic trees based on rpoB, showing relationships in the genus Aeromonas. Type strains are included.
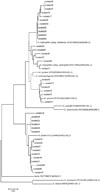
Table 1
Primers Used in 16S rRNA, gyrB, and rpoB Sequencing

Target | Forward | Backward |
---|---|---|
16S rRNA | AGAGTTTGATCMTGGCTCAG | TACGGYTACCTTGTTACGACTT |
gyrB* | TCCGGCGGTCTGCACGGCGT | TTGTCCGGGTTGTACTCGTC |
rpoB† | GCAGTGAAAGARTTCTTTGGTTC | GTTGCATGTTNGNACCCAT |
Table 2
Comparison of MALDI-TOF MS, 16S rRNA Sequencing, and a Conventional Method with Housekeeping Gene Sequencing
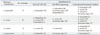
MALDI-TOF MS, Matrix Assisted Laser Desorption/Ionization Time of Flight Mass Spectrometry.
*A. aquariourm/A. caviae (5), A. aquariorum/A. trota (1), A. aquariorum/A. hydrophila/A. caviae (1), A. hydrophila/A. caviae (4), A. hydrophila/A. media (1), A. veronii/A. media (2).
†A. hydrophila/A. caviae (2), Aeromonas spp. (10).
‡A. aquariorum/A. caviae (2), A. caviae/A. trota (2), A. hydrophila/A. caviae (17).
§A. hydrophila/A. caviae (1), Aeromonas spp. (6).
∥A. aquariorum/A. caviae (1).
References
1. Steinberg J, Burd EM. Other Gram-Negative and Gram-Variable Bacilli. In : Mandell GL, Bennett JE, Dolin R, editors. Mandell, Douglas, and Bennett's Principles and Practice of Infectious Diseases. 7th ed. New York: Churchill Livingstone;2010. p. 465–478.
2. Janda JM, Abbott SL. The genus Aeromonas: taxonomy, pathogenicity, and infection. Clin Microbiol Rev. 2010; 23:35–73.


3. Benagli C, Demarta A, Caminada A, Ziegler D, Petrini O, Tonolla M. A rapid MALDI-TOF MS identification database at genospecies level for clinical and environmental Aeromonas strains. PLoS One. 2012; 7:e48441.
4. Nhung PH, Hata H, Ohkusu K, Noda M, Shah MM, Goto K, et al. Use of the novel phylogenetic marker dnaJ and DNA-DNA hybridization to clarify interrelationships within the genus Aeromonas. Int J Syst Evol Microbiol. 2007; 57(Pt 6):1232–1237.


5. Küpfer M, Kuhnert P, Korczak BM, Peduzzi R, Demarta A. Genetic relationships of Aeromonas strains inferred from 16S rRNA, gyrB and rpoB gene sequences. Int J Syst Evol Microbiol. 2006; 56(Pt 12):2743–2751.


6. Lamy B, Kodjo A, Laurent F. ColBVH Study Group. Identification of Aeromonas isolates by matrix-assisted laser desorption ionization time-of-flight mass spectrometry. Diagn Microbiol Infect Dis. 2011; 71:1–5.


7. Murray PR. Matrix-assisted laser desorption ionization time-of-flight mass spectrometry: usefulness for taxonomy and epidemiology. Clin Microbiol Infect. 2010; 16:1626–1630.


8. Yáñez MA, Catalán V, Apráiz D, Figueras MJ, Martínez-Murcia AJ. Phylogenetic analysis of members of the genus Aeromonas based on gyrB gene sequences. Int J Syst Evol Microbiol. 2003; 53(Pt 3):875–883.


9. Korczak B, Christensen H, Emler S, Frey J, Kuhnert P. Phylogeny of the family Pasteurellaceae based on rpoB sequences. Int J Syst Evol Microbiol. 2004; 54(Pt 4):1393–1399.


10. Tamura K, Peterson D, Peterson N, Stecher G, Nei M, Kumar S. MEGA5: molecular evolutionary genetics analysis using maximum likelihood, evolutionary distance, and maximum parsimony methods. Mol Biol Evol. 2011; 28:2731–2739.


11. Mellmann A, Cloud J, Maier T, Keckevoet U, Ramminger I, Iwen P, et al. Evaluation of matrix-assisted laser desorption ionization-time-of-flight mass spectrometry in comparison to 16S rRNA gene sequencing for species identification of nonfermenting bacteria. J Clin Microbiol. 2008; 46:1946–1954.


12. Chong Y, Yi KN, Lee SY. Cultural and biochemical characteristics of clinical isolates of Aeromonas hydrophila. Yonsei Med J. 1980; 21:52–57.


13. Kwak CG, Chong Y, Lee SY, Hong CS, Koh CM. Species and antimicrobial susceptibility of Aeromonas strains isolated from patients and environmental sources. Korean J Infect Dis. 1987; 19:167–177.
14. Janda JM, Abbott SL. Evolving concepts regarding the genus Aeromonas: an expanding Panorama of species, disease presentations, and unanswered questions. Clin Infect Dis. 1998; 27:332–344.


15. Soler L, Yáñez MA, Chacon MR, Aguilera-Arreola MG, Catalán V, Figueras MJ, et al. Phylogenetic analysis of the genus Aeromonas based on two housekeeping genes. Int J Syst Evol Microbiol. 2004; 54(Pt 5):1511–1519.


16. Horneman AJ, Ali A. Aeromonas. In : Versalovic J, Carroll KC, Funke G, Jorgensen JH, Landry ML, Warnock DW, editors. Manual of Clinical Microbiology. 10th ed. Washington D.C.: ASM Press;2011. p. 658–665.
17. Martinez-Murcia AJ, Benlloch S, Collins MD. Phylogenetic interrelationships of members of the genera Aeromonas and Plesiomonas as determined by 16S ribosomal DNA sequencing: lack of congruence with results of DNA-DNA hybridizations. Int J Syst Bacteriol. 1992; 42:412–421.


18. Blondiaux N, Gaillot O, Courcol RJ. [MALDI-TOF mass spectrometry to identify clinical bacterial isolates: evaluation in a teaching hospital in Lille]. Pathol Biol (Paris). 2010; 58:55–57.
19. Saavedra MJ, Figueras MJ, Martínez-Murcia AJ. Updated phylogeny of the genus Aeromonas. Int J Syst Evol Microbiol. 2006; 56(Pt 10):2481–2487.
20. Abbott SL, Cheung WK, Janda JM. The genus Aeromonas: biochemical characteristics, atypical reactions, and phenotypic identification schemes. J Clin Microbiol. 2003; 41:2348–2357.


21. Morandi A, Zhaxybayeva O, Gogarten JP, Graf J. Evolutionary and diagnostic implications of intragenomic heterogeneity in the 16S rRNA gene in Aeromonas strains. J Bacteriol. 2005; 187:6561–6564.


22. Donohue MJ, Smallwood AW, Pfaller S, Rodgers M, Shoemaker JA. The development of a matrix-assisted laser desorption/ionization mass spectrometry-based method for the protein fingerprinting and identification of Aeromonas species using whole cells. J Microbiol Methods. 2006; 65:380–389.


23. Martínez-Murcia A, Monera A, Alperi A, Figueras MJ, Saavedra MJ. Phylogenetic evidence suggests that strains of Aeromonas hydrophila subsp. dhakensis belong to the species Aeromonas aquariorum sp. nov. Curr Microbiol. 2009; 58:76–80.


24. Sandrin TR, Goldstein JE, Schumaker S. MALDI TOF MS profiling of bacteria at the strain level: a review. Mass Spectrom Rev. 2013; 32:188–217.

