Abstract
Purpose
The validity of tomotherapy-based simultaneous integrated boost (TOMOSIB) was assessed in terms of acute intestinal/urinary toxicity by comparing with 3-dimensional conformal radiotherapy (3DCRT) in cases of whole-pelvis radiation therapy (WPRT) for prostate cancer.
Materials and Methods
Thirty-eight consecutive patients who underwent curative WPRT were retrospectively reviewed. Twenty six (68.4%) received 3DCRT and the others (31.6%) were treated with TOMOSIB. A local boost to the prostate circumferential area was added to WPRT sequentially for 3DCRT and concomitantly for TOMOSIB. The total median prostate or prostatic bed dose was 64.8 Gy including median 45.0 Gy of WPRT. Acute toxicities were assessed according to RTOG criteria.
Results
Overall intestinal toxicity was lower in TOMOSIB group than 3DCRT group (p=0.008). When it was divided into rectum and non-rectum intestine (NRI), TOMOSIB showed borderline superiority only in NRI toxicity (p=0.047). For the urinary toxicity, there was no significant difference between two groups (p=0.796). On dosimetric analysis for the rectum and bladder, dose delivered to 80% (p<0.001) and volume receiving 25-40 Gy (p<0.001) were remarkably higher in 3DCRT. For the NRI, only maximum dose showed significant results between two groups (p<0.001).
Conclusion
Intestinal toxicity should be verified with more detailed anatomic categorization such as rectum and NRI. TOMOSIB could not reduce urinary toxicity because of inevitably high dose exposure to the prostatic urethra. Current dosimetry system did not properly reflect intestinal/urinary toxicity, and suitable dosimetric guidelines are needed in TOMOSIB.
In the curative treatment of prostate cancer, whole-pelvis radiation therapy (WPRT) is prone to intestinal/urinary toxicities compared with prostate-only radiation. Although acute intestinal/urinary toxicity has recently decreased in WPRT by using the intensity-modulation technique,1,2,3,4 the intestine, bladder, and other organs are still vulnerable to high-dose radiation because of their distensible properties.5 There are also subtle differences in patient setup and dose delivery method according to institutional protocol, which can aggravate these kinds of problems.
Tomotherapy delivers more uniform dose to the target volume and protects normal tissue through multi-leaf collimators that can be used at any angle with 360-degree rotation.6 Its mega-voltage computed tomography (MVCT) system is used for correcting interfractional deviation which originated from internal organ motion or patient setup error. Daily MVCT imaging allows patients to be assessed for interfractional changes of the intestine (especially rectum) or bladder in prostate cancer radiotherapy. Thus, high-dose irradiation using simultaneous integrated boost (SIB) can be facilitated by tomotherapy.
Hypofractionation with SIB can shorten the overall treatment time and increase the local control rate. However, it also has limitations in avoiding acute toxicity problems even with intensity-modulated radiation therapy (IMRT) technique. As there is no definite guideline for fraction size and treatment duration, the validity of SIB remains to be discussed more in the era of IMRT. In this study, we intended to infer the usefulness of tomotherapy-based SIB (TOMOSIB) in terms of acute intestinal/urinary toxicity, by comparing it with 3-dimensional conformal radiotherapy (3DCRT), in cases of WPRT for prostate cancer.
This study was approved by the Institutional Review Board of our institution (approval number: YWMR-13-5-064).
Medical records and dosimetric data of 38 patients who received WPRT between Jan 2007 and Dec 2012 were retrospectively reviewed. All pathologic diagnoses were adenocarcinoma, and median age was 68 years (range, 50-79 years). The aim of radiotherapy was definitive treatment for 10 patients (26.3%) and salvage for the other 28 patients (73.7%). Hormonal agents were administered to 20 patients (52.6%) before radiation. Twelve patients (31.6%) were treated with TOMOSIB. Patient characteristics related to clinical factors are shown in Table 1. All patients had a performance status of ECOG 0 or 1 and none had previous pelvic irradiation.
All patients were scanned with planning computed tomography (CT) using a 3-5 mm slice thickness including the pelvis and lower abdomen. The patients were initially positioned on the treatment couch using external markings. During simulation, patients lay supine with their hands on the anterior chest or arm holder. For TOMOSIB patients, body fixation devices such as Wing Board or Vac-Lok Cusion (CIVCO Medical Solutions, Kalona, IA, USA) were supplemented. After scanning, CT datasets and structures were transferred to a planning workstation using Digital Imaging and Communications in Medicine system. In order to reproduce this condition during daily radiotherapy, all patients were instructed to have their urinary bladder filled 1-2 hours before scanning. An air balloon of 60 mL volume was inserted into the rectum of TOMOSIB patients.
The clinical target volume (CTV) was classified as a three-step (low-, intermediate-, and high-risk CTV) according to each irradiated dose level. Low-risk CTV was defined as pelvic lymph node area such as regional, internal iliac, external iliac, obturator, and distal common iliac lymph nodes. Presacral nodes were not routinely included. Seminal vesicle and prostate circumference was assigned to intermediate- and high-risk CTV, respectively. For the patients who received radical prostatectomy previously, the location of surgical clips was used as a reference point to contour surgical bed of prostate gland (high-risk CTV) or seminal vesicle (intermediate-risk CTV). They were contoured on each axial CT image with 0.5 to 1.0-cm radial expansion around each anatomic structure. The volume of bladder and rectum was also defined including their wall and inner space as a single structure, respectively. Rectum contouring was extended from the anus to the rectosigmoid flexure. Non-rectum intestine (NRI) was delineated to encompass small bowel and colon from the upper margin of the rectum to the interspace between the 4-5th lumbar spine.
A dose of 45 Gy was administered to low-risk CTV with 4-field box technique. After 45 Gy, a dose of 9 Gy was added to intermediate-risk CTV followed by high-risk CTV dose of 16.2 Gy and 10.8 Gy for definitive and postoperative irradiation, respectively. All local boosts to intermediate- and high-risk CTV were implemented with 5 to 7 portals. The irradiated dose was adjusted according to each patient's disease status. As a result, median irradiated dose was 64.8 Gy (range, 59.4-75.6 Gy) to high-risk CTV and 45.0 Gy (range, 41.4-45.0 Gy) to low-risk CTV. The treatment schedule was 5 fractions per week with a daily dose of 1.8 Gy. The Pinnacle system (ADAC Laboratories, Milpitas, CA, USA) was used for treatment planning. Radiation was delivered using a 10 to 15-MV photon beam and multi-leaf collimators with 0.7 to 1.0-cm expansion around each CTV.
TOMOSIB was planned by IMRT mode. The prescribed doses in definitive radiotherapy were 50.4 Gy, 60.2 Gy, and 70.0 Gy for low-, intermediate-, and high-risk CTV, respectively, with 28 fractions. Those of postoperative irradiation were 51.0 Gy, 54.0 Gy, and 66.0 Gy for low-, intermediate-, and high-risk CTV, respectively, with 30 fractions. Due to individual dose adjustment, actual median irradiated dose was 66.6 Gy (range, 66.0-75.0 Gy) for high-risk CTV and 51.0 Gy (range, 50.4-54.0 Gy) for low-risk CTV. Dose to rectum, bladder, NRI, and femoral heads was constrained by mean dose of 27 Gy, 25 Gy, 25 Gy, and 30 Gy, respectively. The treatment schedule was 5 fractions per week with a daily dose of 2.2-2.5 Gy for high-risk CTV. Inverse treatment planning, which is based on iterative least squares minimization of an objective function, as described by Shepard, et al.,7 was applied using TomoTherapy Hi-Art System version 2.0 (TomoTherapy Incorporated, Madison, WI, USA). Daily MVCT was scanned prior to each treatment session for the image-guided verification.
During radiotherapy, acute intestinal/urinary toxicity was estimated on a weekly basis according to RTOG scoring system.8 Toxicity assessment was dependent mainly on patients' subjective complaints, and the maximum toxicity incidence was compared between TOMOSIB and 3DCRT. We focused on the incidence, not on the duration or recurrence of each symptom, in order to exclude the influence of medicines used for symptom palliation. Pretreatment symptoms were also excluded to reflect radiation toxicity as purely as possible.
Correlations between clinical factors and acute intestinal/urinary toxicity were evaluated by chi-square test. Student t-test was used for dosimetric comparison of each normal organ between TOMOSIB and 3DCRT. Statistical significance was defined at the p<0.05 level. The SPSS (Version 20.0, SPSS Inc., Chicago, IL, USA) program was used for analysis.
Most treatment processes were tolerable, and none of the 38 patients experienced interruption due to radiation-induced toxicity. Only one 54-year-old patient, who received 3DCRT, suffered from underlying internal hemorrhoid and anal fissure. This patient received conservative surgical care after 45 Gy.
Twenty-five patients (65.8%) experienced acute intestinal toxicity and 15 patients (39.5%) suffered from Grade 2 toxicity. No Grade 3-5 toxicity was observed. Among 15 patients who had Grade 2 intestinal toxicity, 7 exhibited defecation-related symptoms such as diarrhea or tenesmus, 3 showed severe anal pain requiring analgesics, and 4 complained of rectal bleeding. One patient suffered from both frequent defecation and rectal bleeding.
The proportion of Grade 2 or higher intestinal toxicity was relatively lower in TOMOSIB group than in 3DCRT group (p=0.008) (Table 2). When intestinal toxicity was analyzed separately for rectum and NRI, there was no significant difference in acute rectal toxicity between two groups (p=0.191) (Table 3). TOMOSIB displayed borderline superiority only in NRI toxicity (p=0.047). Other clinical factors, such as age, radical prostatectomy, androgen deprivation therapy (ADT), and pretreatment urinary symptom, were not associated with Grade 2 or higher intestinal toxicity. Postoperative irradiation was not different from definitive radiotherapy for intestine, rectum, and NRI toxicity. All toxicities of Grade 2 or higher appeared between the 3rd and the 7th week during radiotherapy. At the 5th week of radiotherapy, when the WPRT dose levels of both groups were almost the same (around 45 Gy), the cumulative incidence of Grade 2 or higher toxicity was 0.0% (0 patient) in TOMOSIB group and 30.8% (8 patients) in 3DCRT group (Fig. 1). More specifically, it was 11.5% (3 patients) for the rectum and 19.2% (5 patients) for the NRI in 3DCRT group (Fig. 2).
Thirty-three patients (86.8%) revealed acute urinary toxicity and 21 patients (55.3%) suffered from Grade 2 or higher toxicity including 1 patient (2.6%) of Grade 3 toxicity. No Grade 4-5 toxicity was observed. Among 21 patients (55.3%) who had Grade 2 or higher toxicity, 18 experienced frequency-related symptoms and the others showed painful voiding.
The ratio of Grade 2 or higher toxicity did not differ significantly between TOMOSIB and 3DCRT groups (p=0.796) (Table 2). Age, radical prostatectomy, and pretreatment symptom were not associated with Grade 2 or higher toxicity. Postoperative irradiation also was not different from definitive radiotherapy in the ratio of higher urinary toxicity (p=0.153). Only ADT seemed to be associated (p=0.012). Fourteen (77.8%) out of 18 patients, who were treated with ADT, revealed severe toxicity. All toxicities of Grade 2 or higher appeared between the 2nd and 8th week during radiotherapy (Fig. 3). At the 5th week of radiotherapy, the cumulative incidence of Grade 2 or higher toxicity was 41.7% (5 patients) in TOMOSIB group and 26.9% (7 patients) in 3DCRT group.
In all TOMOSIB plans, dose distributions for each CTV and normal organ were generally acceptable. However, the dose homogeneity for CTV was limited compared with that of 3DCRT plan (Fig. 4). When dosimetric assessment was confined to the dose level at the time of full irradiation for the pelvic lymph node area, most parameters for normal organs were better in TOMOSIB than in 3DCRT. For the rectum, the mean dose, dose delivered to 80% volume, and the volume receiving 15-45 Gy were lower in TOMOSIB than in 3DCRT. For the bladder, dose delivered to 80% volume and the volume receiving 25-45 Gy were lower in TOMOSIB than in 3DCRT. For the NRI, overall dosimetric data did not show significant difference between TOMOSIB and 3DCRT, except maximum dose. The dose-volume histogram (DVH) data for concerned normal organs are shown in Table 4. In terms of treatment aim, dose-volume relationship between definitive and postoperative irradiation was not definitely different for the rectum, bladder, and NRI (Table 5).
The main objective of this study was to compare the acute intestinal/urinary toxicities of TOMOSIB with those of 3DCRT during WPRT. Generally, TOMOSIB was more effective than 3DCRT in alleviating acute intestinal toxicity. However, this was not obvious when the intestine was divided into rectum and NRI. Acute urinary toxicity was not reduced by TOMOSIB despite better bladder dose distribution. Dosimetric values for rectum and bladder were not correlated with acute intestinal/urinary toxicities. These data suggest that current dosimetric analysis for TOMOSIB should be reassessed and more careful approaches are needed in terms of radiation toxicity.
WPRT is recommended for the treatment of locally advanced prostate cancer considering high probability of lymph node metastasis.9,10,11 Roach, et al.12,13 suggested a formula to predict the risk of lymph node involvement in clinically localized prostate cancer using prostate-specific antigen and Gleason score. In the RTOG 9413 trial, patients treated with WPRT experienced a 4-year progression-free survival of 54.2% compared to 47.0% in those with prostate-only radiation (p=0.020), when 1323 patients with an estimated risk of lymph node involvement of 15% were randomized to both groups.9 However, WPRT generally leads to more severe intestinal/urinary toxicity than prostate-only radiotherapy. This problem cannot be solved even with IMRT technique. Deville, et al.14 reported that whole-pelvis IMRT increased acute gastrointestinal toxicity in comparison with prostate-only IMRT. In addition, SIB can aggravate this problem. Thus, current SIB technique needs to be investigated. We analyzed TOMOSIB in terms of acute intestinal/urinary toxicity by comparing it with 3DCRT.
For acute intestinal toxicity, TOMOSIB exhibited relatively good results despite its higher dose level than that of 3DCRT. When the NRI was analyzed separately from intestine, the toxicity gap between two groups decreased. However, TOMOSIB still seemed to be satisfactory for the rectal toxicity despite relatively high dose exposure. This could be confirmed by relatively few cases of Grade 2 or higher toxicity and better dosimetric results (Table 4).
The effect of tomotherapy itself needs to be ascertained with that of rectal ballooning. Correlations between rectal dose exposure and gastrointestinal toxicity were reported in previous studies along with the usefulness of balloon insertion.15,16,17,18,19,20 According to Storey, et al.,15 rectal volume over 70 Gy is significantly related to its toxicity. Michalski, et al.17 showed that acute bowel symptoms are associated with the percentage of the rectum receiving a reference dose or greater through multivariate analysis of 219 patients (p=0.012). This kind of ballooning effect has also been mentioned by Cho, et al.18 for the SIB treatment planned with IMRT method, where the toxicity reduction was not as remarkable as for 3DCRT. In our study, all TOMOSIB patients were treated with balloon inserted. Therefore, low incidence of Grade 2 or higher intestinal toxicity in TOMOSIB cannot always be considered as the influence of tomotherapy itself.
The intestinal/urinary toxicity results were not always consistent with its dosimetric values. For example, the NRI dosimetry data were not significantly different between TOMOSIB and 3DCRT, except maximum dose, despite its toxicity difference. One of the reasons may be non-standardized contouring system for NRI. For urinary system, the disagreement pattern between toxicity and dosimetry was the same as for the NRI. This was the same in other IMRT studies for urinary toxicity.21,22,23 One possible reason is the high dose exposure to the bladder area which was overlapped directly with high-risk CTV. Subjective bladder filling can be another factor for high dose exposure because of its irregular distension.
Most of all, hypofractionated schedule of TOMOSIB with higher dose level could lead to unsatisfactory outcome in urinary toxicity, which can be estimated in terms of urethral and prostate doses. According to Keiler, et al.,21 tomotherapy could not provide satisfactory genitourinary toxicity results in the post-prostatectomy subset because of inevitable high dose exposure to urethral and prostate areas. Di Muzio, et al.1 reported the excellent genitourinary toxicity results in a phase I-II study on hypofractionated TOMOSIB. However, this may not be compared with our study because only half of the 60 patients received WPRT. In our study, TOMOSIB showed higher maximum toxicity incidence from the 1st to the 5th week during radiotherapy even though most bladder dosimetric parameters were lower than those of 3DCRT, except the maximum dose. According to DVHs of TOMOSIB, the prostatic circumferential area showed relatively poor dose homogeneity, which means much more irradiation to the prostatic urethra than initially planned dose (Fig. 4).
Usually, postoperative irradiation appears to be more vulnerable in terms of intestinal/urinary toxicity than definitive radiotherapy, because positional change of each normal organ after surgical resection can increase the possibility of overlapping with CTV dose area. In our study, however, the postoperative irradiation did not show significant toxicity difference from definitive radiotherapy for the rectum, bladder, and NRI. Dose-volume relationship was almost the same pattern as clinical outcome. Only in the low-dose section of NRI DVH, the volume of postoperative irradiation had a tendency to be relatively large (Table 5). Therefore, surgical influence can be considered slight in overall dose distribution and toxicity.
There have been some earlier reports about the negative influence of hormone therapy upon urinary toxicity in patients treated with radiotherapy, although the mechanism remains unclear.24,25,26 In our study, ADT seemed to be related to Grade 2 or higher urinary toxicity, but this cannot be regarded as the influence of ADT itself because of the small number of cases and diverse kinds of hormonal agents. The toxicity increase by ADT should be evaluated in more detail by homogeneous hormonal agents in future studies.
Ideally, late toxicity with long-term follow-up is the ultimate endpoint to be evaluated. However, only acute toxicity was analyzed in this study for the following reasons. First, acute toxicity can be an index for predicting the late toxicity. 24,27,28 Second, late toxicity assessment is limited due to palliated symptoms after conservative care or medication while acute toxicity is relatively free from this point. In addition, acute toxicity usually progresses to late toxicity in the same way for both gastrointestinal and genitourinary symptoms.28
This study has a limitation stemming from its small number of patients, apart from the heterogeneity of treatment such as definitive radiotherapy versus postoperative irradiation. These points should be improved with more systematic approach in future studies.
Taken together, acceptable acute intestinal toxicity results by TOMOSIB should be verified with more detailed anatomic categorization such as rectum and NRI. In estimating urinary toxicity, bladder dosimetry has some limitations, and prostatic urethra must be concerned. Consequently, more deliberate approach with proper dosimetric guidelines is suggested in TOMOSIB, especially for WPRT.
Figures and Tables
![]() | Fig. 1The cumulative incidence of the Grade 2 or higher intestinal toxicity from the 1st to the 8th week during radiotherapy (TOMOSIB vs. 3DCRT).TOMOSIB, tomotherapy-based simultaneous integrated boost; 3DCRT, 3-dimensional conformal radiotherapy. |
![]() | Fig. 2The cumulative incidence of the Grade 2 or higher toxicity for the rectum (A) and the NRI (B) from the 1st to the 8th week during radiotherapy (TOMOSIB vs. 3DCRT). NRI, non-rectum intestine; TOMOSIB, tomotherapy-based simultaneous integrated boost; 3DCRT, 3-dimensional conformal radiotherapy. |
![]() | Fig. 3The cumulative incidence of the Grade 2 or higher urinary toxicity from the 1st to the 8th week during radiotherapy (TOMOSIB vs. 3DCRT). TOMOSIB, tomotherapy-based simultaneous integrated boost; 3DCRT, 3-dimensional conformal radiotherapy. |
![]() | Fig. 4A typical example of dose-volume histogram at completion of whole-pelvis radiation for (A) TOMOSIB and (B) 3DCRT. CTV, clinical target volume; 3DCRT, 3-dimensional conformal radiotherapy; TOMOSIB, tomotherapy-based simultaneous integrated boost. |
Table 1
Patient Characteristics
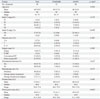
Table 2
Univariate Analysis for Acute Intestinal/Urinary Toxicity of Grade 2 or Higher
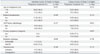
Table 3
Univariate Analysis for Acute Rectal/NRI Toxicity of Grade 2 or Higher
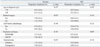
Table 4
Dosimetric Comparison for Rectum, Bladder, and NRI between TOMOSIB and 3DCRT When All Prescription Doses Were Irradiated to the Pelvic Lymph Node Area for Both Groups
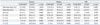
D80, dose delivered to 80% volume; Vn, volume percentage above nGy; NRI, non-rectum intestine; 3DCRT, 3-dimensional conformal radiotherapy; TOMOSIB, tomotherapy-based simultaneous integrated boost.
*For TOMOSIB, whole pelvic dose ranged from 50.4-54.0 Gy with simultaneous integrated boost to prostatic circumference.
†For 3DCRT, whole pelvic dose ranged from 41.4-45.0 Gy.
Table 5
Dosimetric Comparison for Rectum, Bladder, and NRI between Definitive and Postoperative Radiotherapy When All Prescription Doses Were Irradiated to the Pelvic Lymph Node Area for Both Groups
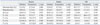
ACKNOWLEDGEMENTS
This work was supported by a research grant from Yonsei University Wonju College of Medicine (YUWCM 2013-56).
Notes
References
1. Di Muzio N, Fiorino C, Cozzarini C, Alongi F, Broggi S, Mangili P, et al. Phase I-II study of hypofractionated simultaneous integrated boost with tomotherapy for prostate cancer. Int J Radiat Oncol Biol Phys. 2009; 74:392–398.


2. Arcangeli S, Saracino B, Petrongari MG, Gomellini S, Marzi S, Landoni V, et al. Analysis of toxicity in patients with high risk prostate cancer treated with intensity-modulated pelvic radiation therapy and simultaneous integrated dose escalation to prostate area. Radiother Oncol. 2007; 84:148–155.


3. Muren LP, Wasbø E, Helle SI, Hysing LB, Karlsdottir A, Odland OH, et al. Intensity-modulated radiotherapy of pelvic lymph nodes in locally advanced prostate cancer: planning procedures and early experiences. Int J Radiat Oncol Biol Phys. 2008; 71:1034–1041.


4. Cozzarini C, Fiorino C, Di Muzio N, Alongi F, Broggi S, Cattaneo M, et al. Significant reduction of acute toxicity following pelvic irradiation with helical tomotherapy in patients with localized prostate cancer. Radiother Oncol. 2007; 84:164–170.


5. Chang JS, Yoon HI, Cha HJ, Chung Y, Cho Y, Keum KC, et al. Bladder filling variations during concurrent chemotherapy and pelvic radiotherapy in rectal cancer patients: early experience of bladder volume assessment using ultrasound scanner. Radiat Oncol J. 2013; 31:41–47.


6. Ruchala KJ, Olivera GH, Schloesser EA, Mackie TR. Megavoltage CT on a tomotherapy system. Phys Med Biol. 1999; 44:2597–2621.


7. Shepard DM, Olivera GH, Reckwerdt PJ, Mackie TR. Iterative approaches to dose optimization in tomotherapy. Phys Med Biol. 2000; 45:69–90.


8. Cox JD, Stetz J, Pajak TF. Toxicity criteria of the Radiation Therapy Oncology Group (RTOG) and the European Organization for Research and Treatment of Cancer (EORTC). Int J Radiat Oncol Biol Phys. 1995; 31:1341–1346.


9. Roach M 3rd, DeSilvio M, Lawton C, Uhl V, Machtay M, Seider MJ, et al. Phase III trial comparing whole-pelvic versus prostate-only radiotherapy and neoadjuvant versus adjuvant combined androgen suppression: Radiation Therapy Oncology Group 9413. J Clin Oncol. 2003; 21:1904–1911.


10. Seaward SA, Weinberg V, Lewis P, Leigh B, Phillips TL, Roach M 3rd. Improved freedom from PSA failure with whole pelvic irradiation for high-risk prostate cancer. Int J Radiat Oncol Biol Phys. 1998; 42:1055–1062.


11. Seaward SA, Weinberg V, Lewis P, Leigh B, Phillips TL, Roach M 3rd. Identification of a high-risk clinically localized prostate cancer subgroup receiving maximum benefit from whole-pelvic irradiation. Cancer J Sci Am. 1998; 4:370–377.
12. Roach M 3rd. Re: The use of prostate specific antigen, clinical stage and Gleason score to predict pathological stage in men with localized prostate cancer. J Urol. 1993; 150:1923–1924.


13. Roach M 3rd, Marquez C, Yuo HS, Narayan P, Coleman L, Nseyo UO, et al. Predicting the risk of lymph node involvement using the pre-treatment prostate specific antigen and Gleason score in men with clinically localized prostate cancer. Int J Radiat Oncol Biol Phys. 1994; 28:33–37.


14. Deville C, Both S, Hwang WT, Tochner Z, Vapiwala N. Clinical toxicities and dosimetric parameters after whole-pelvis versus prostate-only intensity-modulated radiation therapy for prostate cancer. Int J Radiat Oncol Biol Phys. 2010; 78:763–772.


15. Storey MR, Pollack A, Zagars G, Smith L, Antolak J, Rosen I. Complications from radiotherapy dose escalation in prostate cancer: preliminary results of a randomized trial. Int J Radiat Oncol Biol Phys. 2000; 48:635–642.


16. Peeters ST, Heemsbergen WD, van Putten WL, Slot A, Tabak H, Mens JW, et al. Acute and late complications after radiotherapy for prostate cancer: results of a multicenter randomized trial comparing 68 Gy to 78 Gy. Int J Radiat Oncol Biol Phys. 2005; 61:1019–1034.


17. Michalski JM, Winter K, Purdy JA, Parliament M, Wong H, Perez CA, et al. Toxicity after three-dimensional radiotherapy for prostate cancer on RTOG 9406 dose Level V. Int J Radiat Oncol Biol Phys. 2005; 62:706–713.


18. Cho JH, Lee CG, Kang DR, Kim J, Lee S, Suh CO, et al. Positional reproducibility and effects of a rectal balloon in prostate cancer radiotherapy. J Korean Med Sci. 2009; 24:894–903.


19. Bastasch MD, Teh BS, Mai WY, McGary JE, Grant WH 3rd, Butler EB. Tolerance of endorectal balloon in 396 patients treated with intensity-modulated radiation therapy (IMRT) for prostate cancer. Am J Clin Oncol. 2006; 29:8–11.


20. Ronson BB, Yonemoto LT, Rossi CJ, Slater JM, Slater JD. Patient tolerance of rectal balloons in conformal radiation treatment of prostate cancer. Int J Radiat Oncol Biol Phys. 2006; 64:1367–1370.


21. Keiler L, Dobbins D, Kulasekere R, Einstein D. Tomotherapy for prostate adenocarcinoma: a report on acute toxicity. Radiother Oncol. 2007; 84:171–176.


22. Zelefsky MJ, Chan H, Hunt M, Yamada Y, Shippy AM, Amols H. Long-term outcome of high dose intensity modulated radiation therapy for patients with clinically localized prostate cancer. J Urol. 2006; 176(4 Pt 1):1415–1419.


23. Teh BS, Mai WY, Augspurger ME, Uhl BM, McGary J, Dong L, et al. Intensity modulated radiation therapy (IMRT) following prostatectomy: more favorable acute genitourinary toxicity profile compared to primary IMRT for prostate cancer. Int J Radiat Oncol Biol Phys. 2001; 49:465–472.


24. Yoshimura R, Iwata M, Shibuya H, Sakai Y, Kihara K. Acute and late genitourinary toxicity of conformal radiotherapy for prostate cancer. Radiat Med. 2006; 24:553–559.


25. Ishikawa H, Tsuji H, Kamada T, Hirasawa N, Yanagi T, Mizoe JE, et al. Adverse effects of androgen deprivation therapy on persistent genitourinary complications after carbon ion radiotherapy for prostate cancer. Int J Radiat Oncol Biol Phys. 2008; 72:78–84.


26. Peeters ST, Hoogeman MS, Heemsbergen WD, Slot A, Tabak H, Koper PC, et al. Volume and hormonal effects for acute side effects of rectum and bladder during conformal radiotherapy for prostate cancer. Int J Radiat Oncol Biol Phys. 2005; 63:1142–1152.

