Abstract
Purpose
The aim of this study was to investigate whether pathologic changes in zonula occludens-1 (ZO-1) are induced by interleukin-13 (IL-13) in the experimental minimal-change nephrotic syndrome (MCNS) model and to determine whether montelukast, a leukotriene receptor antagonist, has an effect on ZO-1 restoration in cultured human podocytes.
Materials and Methods
Human podocytes cultured on bovine serum albumin-coated plates were treated with different doses of IL-13 and montelukast and then examined for distribution using confocal microscopy and for ZO-1 protein levels using Western blotting.
Results
ZO-1 was internalized and shown to accumulate in the cytoplasm of human podocytes in an IL-13 dose-dependent manner. High doses (50 and 100 ng/mL) of IL-13 decreased the levels of ZO-1 protein at 12 and 24 h (both p<0.01; n=3), which were significantly reversed by a high dose (0.5 µM) montelukast treatment (p<0.01; n=3).
Although minimal-change nephrotic syndrome (MCNS) is the most common cause of nephrotic syndrome during childhood, the various proteins involved in the morphological and functional changes of cytoskeletal proteins in MCNS are still elusive.1 The hallmark of MCNS pathology is the foot process (FP) effacement of podocytes. Physiologically, FPs remain wide open to serve as a passage for glomerular filtrate and are held together by tenuous slit diaphragms (SDs) that connect the filtration slits.2 Glomerular SDs, a major selective barrier for proteins, reside in the cell-to-cell contact sites between neighboring podocytes, which are thought to be modified adherens junctions that consist of an increasing number of proteins, including nephrin, CD2-associated protein (CD2AP), Neph-1, -2, and -3, podocin, and zonula occludens-1 (ZO-1).3
Recent studies have identified that increased interleukin-13 (IL-13) expression can lead to podocyte injury and induce a minimal-change-like nephropathy.4,5,6 Notably, Lai, et al.7 reported that overexpression of IL-13 caused downregulation of nephrin, podocin, and dystroglycan-all of which are important molecules in maintaining SD integrity- and a concurrent upregulation of B7-1 as well as MCNS in a rat experiment model. Although receptors for IL-13 such as IL-13Rα1 and IL-13Rα2 have also been demonstrated to exist in cultured podocytes,8 the precise role of IL-13 is still not clear in the pathogenesis of MCNS, and there has been no report on the effects of IL-13 on cultured human podocytes in vitro.
While ZO-1 was originally identified as a tight junction component, this molecule was also found to be localized at adherens junctions in podocytes.9,10,11,12 ZO-1 is a cytosolic scaffold that connects the cadherin-catenin complex and actin-based cytoskeletons.9 It is thought to play a key role in adherens junctions through its interaction with various adherens-junction proteins and the formation of multimolecular complexes.10 Therefore, we hypothesized that IL-13 may play an important role in the development of proteinuria in MCNS by exerting a direct effect on ZO-1 in human podocytes.
Leukotrienes are proinflammatory arachidonic acid metabolites produced by leukocytes, eosinophils, mast cells, and macrophages via 5-lipoxygenase.13,14 Leukotrienes are involved in inflammatory cell recruitment, bronchoconstriction, vasodilatation, increased microvascular permeability, exudation of macromolecules, and edema; these interactions are well-described in the pathophysiology of asthma.14,15,16 Although not studied extensively yet in the field of nephrology, IL-13 is known to influence leukotriene levels.17 By inhibiting the proinflammatory effects of leukotriene, leukotriene receptor antagonist (LTRA) montelukast may be useful in the treatment of MCNS. IL-13-1112C/T polymorphism and the haplotype of IL-13 polymorphisms are also associated with LTRA drug responsiveness and useful as a target for modulation of LTRA drug responsiveness.17 Therefore, the aim of our study was to investigate the effects of IL-13 on ZO-1 and to determine whether LTRA could be effective in stabilizing the ZO-1 proteins in an experimental model of MCNS induced by IL-13.
Human conditionally immortalized podocytes (AB8/23), primarily cloned from human glomerular cultures, were characterized and generously provided by Dr. Moin A. Saleem (University of Bristol, Bristol, UK). Human podocytes were maintained in RPMI 1640 (WelGENE Inc., Daegu, South Korea) supplemented with 10% heat-inactivated fetal bovine serum (FBS), Insulin-Transferrin-Selenium-Pyruvate Supplement (ITSP; WelGENE Inc.), and antibiotics. Fresh media was supplied once every 2 days.
To stimulate human podocyte proliferation, cells were cultivated at 33℃ (permissive conditions) in a culture medium supplemented with human recombinant ITSP to induce expression of temperature-sensitive large T antigens. To induce differentiation, podocytes were maintained at 37℃ (non-permissive conditions) for at least 2 weeks, and for subcultures, 0.05% trypsin was used to detach cells from the culture dishes.18
To imitate MCNS-like conditions, cells were incubated with various concentrations of IL-13 (Peprotech Inc., Rocky Hill, NJ, USA) during the indicated time periods (6, 12, and 24 h). IL-13 was administered in 3, 5, 10, 30, 50, and 100 ng/mL doses into 0.5% RPMI with montelukast (Sigma-Aldrich Inc., St. Louis, MO, USA) at 37℃.
Human podocytes that were grown on type I collagen-coated glass cover slips were incubated at 37℃ for 2 h and fixed in 4% paraformaldehyde for 20 min. The cells were then permeabilized in 0.1% tritonX-100 for 10 min, blocked with 10% FBS for 30 min, washed three times for 5 min in phosphate buffered saline (PBS), and labeled with monoclonal rabbit anti-ZO-1 antibody (Invitrogen, Eugene, OR, USA). Phalloidin-FITC (Sigma-Aldrich Inc.) was utilized to stain F-actin. Primary antibody-bound specimens were incubated with 1:1000 (v/v) Alexa 594 for red conjugates and Alexa 488 for green (Invitrogen), respective of secondary anti-rabbit IgG, at room temperature for 40 min and at 37℃ for 20 min without CO2. Nuclei were stained with 4'-6-diamidino-2-phenylindole (DAPI) (1:1000) for 20 min in PBS. Coverslips were mounted in aqueous mountant and viewed with a fluorescence microscope (Leica TCS SP2 AOBS, Mannheim, Germany).
Confluent cell layers were incubated with additives for various time durations, and proteins were extracted using a protein extraction solution PRO-PREP (Intron Biotechnology, Seongnam, Gyeonggi, South Korea) containing phenylmethylsulfonyl fluoride, ethylenediamine tetraacetic acid, pepstatin A, leupeptin, and aprotinin; protein concentrations were then determined as previously described.19 To perform Western blotting for ZO-1, 30 µg of boiled extracts were resolved on 10% SDS-PAGE gels and transferred to polyvinylidene difluoride (PVDF) membranes (Millipore Corp., Medford, MA, USA).
The membranes were then washed with methanol and blocked in 5% fat-free milk before incubation with monoclonal rabbit anti-ZO-1 (Invitrogen). Anti-β-tubulin antibody (Santa Cruz Biotechnology Inc., Santa Cruz, CA, USA) was used as a loading control. After incubation with horseradish peroxidase-conjugated secondary antibodies (Santa Cruz Biotechnology), protein bands were detected using the enhanced chemiluminescence (ECL) detection system (WEST-ZOL® plus; Amersham Biotech Ltd., Bucks, UK). Density values are expressed as percentages of the control. Data on densitometric analysis of the ZO-1/β-tubulin ratio are expressed as mean±standard deviation.
Podocytes were double-stained for ZO-1 and F-actin, and the cell nuclei were stained with DAPI. ZO-1 in human podocytes was highly expressed within the podocyte in the cytoplasmic aspect of the FP membrane, adjacent to the insertion of the SD, and colocalized with β-catenin (Fig. 1A). Staining for ZO-1 was most intense in the cytoplasmic surface of the podocyte FP. ZO-1 and F-actin did not overlap and were located at different sites in the confocal images. From low to high IL-13 concentrations, ZO-1 staining became blurry, which indicated a relocalization of ZO-1 away from the peripheral cell membrane. In the human podocytes under IL-13 conditions, ZO-1 was internalized into the cytoplasm from the peripheral cell membrane as IL-13 concentrations increased (Fig. 1A). These distributional changes were also observed in F-actin, particularly at the higher IL-13 concentrations (Fig. 1A). Moreover, in high-resolution microscopy, ZO-1 was distributed to the cell contact areas under physiologic conditions without IL-13 yet was redistributed and accumulated into the cytoplasm around the nucleus during a 6-hour incubation period as IL-13 increased from 0 to 30 ng/mL (Fig. 1B). These results suggest that IL-13 may have a substantial impact on the redistribution and rearrangement of ZO-1 molecules and may also disrupt the cytoskeletal connections between F-actin and α-catenin-β-catenin complex in a concentration-dependent manner (Fig. 1A). The internalized ZO-1 proteins were restored to the periphery by treatment with a high dose of 0.5 µM montelukast (Fig. 1B).
A major ZO-1 protein band was found at 205 kDa, and ZO-1 levels were compared with β-tubulin. In human podocytes, density values for ZO-1 protein tended to decrease with IL-13 treatment in a dose-dependent manner at 12 and 24 h. The highest dose (100 ng/mL) of IL-13 significantly decreased the amount of ZO-1 protein by 37.5% at 12 h and by 39% at 24 h (both p<0.01; n=3) (Fig. 2). Similarly, a dose of 50 ng/mL IL-13 significantly decreased the amount of ZO-1 protein by 31.0% at 12 h and by 34.9% at 24 h (both p<0.01; n=3) (Fig. 2). A dose of 10 ng/mL of IL-13 also decreased ZO-1 protein levels to a greater degree at 24 h (35.1%; p<0.05) than at 12 h (Fig. 2). The ZO-1 protein levels that had been reduced by 30 ng/mL IL-13 were restored by a high dose of 0.5 µM montelukast (p<0.01; n=3); however, the reduced levels were not affected by only 0.2 µM of montelukast (Fig. 3). These results suggest that IL-13 may induce a minimal-change-like nephropathy through a reduction in ZO-1 molecules, which can be reversed by a high dose of the LTRA montelukast.
The main goal of this study was to determine whether pathological changes in ZO-1 protein levels could be induced by IL-13. We demonstrated a redistribution and reduction in ZO-1 proteins from human podocytes treated with IL-13. The exposure of ZO-1 molecules to IL-13 caused ZO-1 to move and accumulate internally toward the cytoplasmic actin filaments, suggesting that the observed redistribution and reduction in ZO-1 proteins could be involved in the pathogenesis of MCNS. These results are similar to our previous studies that found that ZO-1 proteins in podocytes were also affected by diabetic conditions, causing hyperpermeability at early stages.20,21
Recent studies have shown strong evidence that proteinuria in MCNS is associated with cytokines and T cell disorders that result in glomerular podocyte dysfunction,4,5,22 as well as B7-1 (CD80) that is expressed on the surface of B cells. An increase in IL-13 production by CD3+, CD4+, and CD8+ T cells was shown to mediate steroid-sensitive nephrotic syndrome in relapse.4,5 Of note, Lai, et al.7 demonstrated that IL-13-transfected rats developed a minimal-change-like glomerulopathy, as characterized by significant worsening of albuminuria over time, generalized swelling, low serum albumin, and hypercholesterolemia. In IL-13-transfected rats, light microscopy showed an absence of significant glomerular changes; however, electron microscopy revealed up to 80% effacement of podocyte FPs, which progressed the nephrotic syndrome.7 Although the nephrotic-range proteinuria was selective in IL-13-transfected rats, no relationship between serum IL-13 and urinary albumin excretion or serum albumin levels was observed.7 Thus, our study attempted to define the pathogenic relationship between IL-13 and ZO-1 protein in the development of podocyte FP effacement with nephrotic-range proteinuria, especially in human podocytes.
The proteinuric conditions in MCNS are usually associated with ultrastructural changes in podocytes, with fusion, widening, retraction, and gradual simplification of the highly specialized interdigitating FPs, which are also accompanied by alterations in the permselectivity of the SD glomerular filtration barrier and the linking of adaptor proteins, including nephrin, podocin, CD2AP, catenins, and ZO-1.22,23,24 Maintenance of podocyte FP structure is pivotal for accurate functioning of the glomerular filtration barrier. The FP effacement results from the detachment of podocytes from the glomerular basement membrane (GBM), and FP retraction leads to disintegration of the cytoskeletal structure and linking adaptor proteins, abnormal movement of the FP over the GBM, and reconstruction of the SD.3,23 Permselectivity of the glomerular filtration barrier, which is composed of a charge-selective barrier and a size-selective barrier, also plays a key role in restricting passage of plasma proteins across the GBM and SD.25,26
ZO is a member of the membrane-associated guanylate kinase homologue family of proteins characterized by their PSD-95/discs-large/Zonula occludens-1 (PDZ) domain; these proteins are critical regulators of tight and adherens junction assembly.27 ZO connects several types of SD proteins through its PDZ domain to the actin cytoskeleton. Although ZO-1 was originally identified as a tight junction component, ZO-1 migrates from its apical location down to the level of the slit membrane at the capillary loop stage of renal development, where it is observed in a punctuate pattern along the filtration slits.28 There are three ZO proteins, ZO-1, -2, and -3, which are multi-domain polypeptides.10 Of these three molecules, ZO-1 has been proposed to be a scaffolding protein between transmembrane and cytoplasmic proteins and possibly forms a link between actin and the cadherin-catenin complex.27 ZO-1 may also participate in signaling events through tyrosine phosphorylation29 and binds directly to α-catenin and actin located on the FPs at the insertions of the SD.28,30 ZO-1 forms a gasket that seals off the intercellular spaces and restricts the movement of proteins, water and solutes along the paracellular pathway at tight junctions, maintaining the polarized distribution of membrane proteins.27 However, the functions of ZO-1 between podocyte FPs are not to serve as a seal but to firmly attach FPs to one another and to stabilize the FP layer against the high filtration pressure at the SD, a modified adherens junction rather than a derivative of the tight junction.
Downregulation of the glomerular gene and production of SD proteins such as nephrin, podocin, and dystroglycan has been reported in both experimental models of nephropathy, such as puromycin nephropathy and adriamycin nephropathy, and human kidney biopsies.31,32,33 In these studies, immunofluorescence staining showed reduced protein production and staining intensity for nephrin, podocin, and dystroglycan, with a shift from a linear pattern to a discontinuous and granular pattern, which returned to normal after steroid treatment. Moreover, certain reports showed that changes in the properties or production of ZO-1 may accompany renal diseases associated with proteinuria.34,35 However, very little is known about how the binding of proteins to ZO-1 is regulated or how cell-signaling pathways control adherens assembly and filtration barrier function, although many different pathways have been implicated.36 Thus, this study explored the production of ZO-1 and the effects of IL-13 and LTRA on ZO-1 restoration in cultured human podocytes. While Kurihara, et al.35 did not show a quantitative change in ZO-1 protein production in a puromycin aminonucleoside (PAN)-treated rat model, we found that ZO-1 proteins were redistributed and reduced in MCNS. In addition, IL-13 significantly decreased ZO-1 protein levels in human podocytes of MCNS, whereas ZO-1 protein production significantly increased in the rat models of PAN-induced nephrosis.37
The present study has several limitations: 1) we were unable to demonstrate all of the signaling pathways of IL-13 via IL-13 receptor (a heterodimer of IL-4Rα and IL-13Rα1) to ZO-1 proteins in podocytes, presumably cascading through tyrosine phosphorylation of ZO-1. 2) Although IL-13 is known to influence leukotriene levels and IL-13-1112C/T polymorphism and the haplotype of IL-13 polymorphisms are known to be significantly associated with LTRA drug responsiveness,17 the mechanism of montelukast in MCNS is not fully understood yet. 3) The efficacy of montelukast as a useful, optional add-on treatment in MCNS should be proven in in vivo experiments to reduce massive proteinuria and potential side effects from corticosteroids. Despite these limitations, the current study demonstrated the potential of LTRA on the reduced ZO-1 proteins in the MCNS model of human podocytes, whereas treatment with angiotensin-converting enzyme inhibitors ameliorated the proteinuria and restored the normal localization of ZO-1 at the SD in the spontaneously proteinuric Munich-Wistar-Froemter rats.38 Additional future studies are necessary to elucidate the exact mechanisms, efficacy, and proper dose of LTRA in the in vivo treatment of MCNS in the future.
In conclusion, our study may provide a base for understanding the ZO-1 molecule in human podocytes. High concentrations of IL-13 increased the disruption of glomerular filtration barrier in SD and FP effacement. ZO-1 proteins were redistributed and reduced in IL-13-treated human podocytes, which was significantly restored after treatment with an LTRA montelukast. Therefore, our findings further strengthen the hypothesis that IL-13 may alter the expression of ZO-1 proteins, resulting in proteinuria, and also provide an explanation for the plausible connection of Th2 cytokines, MCNS, and atopy.
Figures and Tables
Fig. 1
Distributional changes in ZO-1 by IL-13 in human podocytes. ZO-1 was distributed at the peripheral cell membrane and colocalized with β-catenin and actin filament at cell-to-cell contact junctions. High concentrations of IL-13 suppressed and disrupted the immunostaining and linearity of ZO-1 proteins, and accumulated ZO-1 proteins into the cytoplasm around nucleus (A), which improved by treatment with 0.5 µM montelukast (B). Magnification: 1000×; Scale bar=20 µm. ZO-1, zonula occludens-1; IL-13, interleukin-13.
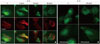
Fig. 2
Effects of IL-13 on ZO-1 protein levels in cultured human podocytes as assayed by Western blotting. ZO-1 levels significantly decreased at IL-13 concentrations of more than 50 ng/mL at 12 and 24 h incubations, compared with the control. Data on the densitometric analysis of the ZO-1/β-tubulin ratio are expressed as mean±SD. Control (100%): the value of (-). *p<0.05. †p<0.01. ZO-1, zonula occludens-1; IL-13, interleukin-13.
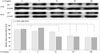
ACKNOWLEDGEMENTS
This research was supported by the Basic Science Research Program through the National Research Foundation of Korea (NRF) and funded by the Ministry of Education, Science and Technology (2011-0013789).
References
2. Kurihara H. [Molecular dynamics of proteins found exclusively in glomerular epithelial cells]. Rinsho Byori. 2000; 48:491–497.
3. Asanuma K, Mundel P. The role of podocytes in glomerular pathobiology. Clin Exp Nephrol. 2003; 7:255–259.


4. Yap HK, Cheung W, Murugasu B, Sim SK, Seah CC, Jordan SC. Th1 and Th2 cytokine mRNA profiles in childhood nephrotic syndrome: evidence for increased IL-13 mRNA expression in relapse. J Am Soc Nephrol. 1999; 10:529–537.
5. Cheung W, Wei CL, Seah CC, Jordan SC, Yap HK. Atopy, serum IgE, and interleukin-13 in steroid-responsive nephrotic syndrome. Pediatr Nephrol. 2004; 19:627–632.


6. Wei CL, Cheung W, Heng CK, Arty N, Chong SS, Lee BW, et al. Interleukin-13 genetic polymorphisms in Singapore Chinese children correlate with long-term outcome of minimal-change disease. Nephrol Dial Transplant. 2005; 20:728–734.


7. Lai KW, Wei CL, Tan LK, Tan PH, Chiang GS, Lee CG, et al. Overexpression of interleukin-13 induces minimal-change-like nephropathy in rats. J Am Soc Nephrol. 2007; 18:1476–1485.


8. Van Den Berg JG, Aten J, Chand MA, Claessen N, Dijkink L, Wijdenes J, et al. Interleukin-4 and interleukin-13 act on glomerular visceral epithelial cells. J Am Soc Nephrol. 2000; 11:413–422.


9. Nagafuchi A. Molecular architecture of adherens junctions. Curr Opin Cell Biol. 2001; 13:600–603.


10. Fanning AS, Anderson JM. Zonula occludens-1 and -2 are cytosolic scaffolds that regulate the assembly of cellular junctions. Ann N Y Acad Sci. 2009; 1165:113–120.


11. Fanning AS, Anderson JM. PDZ domains: fundamental building blocks in the organization of protein complexes at the plasma membrane. J Clin Invest. 1999; 103:767–772.


12. Van Itallie CM, Fanning AS, Bridges A, Anderson JM. ZO-1 stabilizes the tight junction solute barrier through coupling to the perijunctional cytoskeleton. Mol Biol Cell. 2009; 20:3930–3940.


13. Drazen JM, Israel E, O'Byrne PM. Treatment of asthma with drugs modifying the leukotriene pathway. N Engl J Med. 1999; 340:197–206.


15. Bisgaard H. Pathophysiology of the cysteinyl leukotrienes and effects of leukotriene receptor antagonists in asthma. Allergy. 2001; 56 Suppl 66:7–11.


16. Forbes TA, Lunn AJ. Montelukast: a novel therapeutic option in eosinophilic peritonitis. Pediatr Nephrol. 2014; 29:1279–1282.


17. Kang MJ, Lee SY, Kim HB, Yu J, Kim BJ, Choi WA, et al. Association of IL-13 polymorphisms with leukotriene receptor antagonist drug responsiveness in Korean children with exercise-induced bronchoconstriction. Pharmacogenet Genomics. 2008; 18:551–558.


18. Saleem MA, O'Hare MJ, Reiser J, Coward RJ, Inward CD, Farren T, et al. A conditionally immortalized human podocyte cell line demonstrating nephrin and podocin expression. J Am Soc Nephrol. 2002; 13:630–638.


19. Ha TS, Song CJ, Lee JH. Effects of advanced glycosylation endproducts on perlecan core protein of glomerular epithelium. Pediatr Nephrol. 2004; 19:1219–1224.


20. Ha TS. High-glucose and advanced glycosylation end products increased podocyte permeability via PI3-K/Akt signaling. J Mol Med (Berl). 2010; 88:391–400.


21. Ha TS, Choi JY, Park HY, Lee JS. Ginseng total saponin improves podocyte hyperpermeability induced by high glucose and advanced glycosylation endproducts. J Korean Med Sci. 2011; 26:1316–1321.


23. Mundel P, Shankland SJ. Podocyte biology and response to injury. J Am Soc Nephrol. 2002; 13:3005–3015.


24. Reiser J, Kriz W, Kretzler M, Mundel P. The glomerular slit diaphragm is a modified adherens junction. J Am Soc Nephrol. 2000; 11:1–8.


25. Comper WD, Glasgow EF. Charge selectivity in kidney ultrafiltration. Kidney Int. 1995; 47:1242–1251.


26. Goode NP, Shires M, Davison AM. The glomerular basement membrane charge-selectivity barrier: an oversimplified concept? Nephrol Dial Transplant. 1996; 11:1714–1716.


27. Hartsock A, Nelson WJ. Adherens and tight junctions: structure, function and connections to the actin cytoskeleton. Biochim Biophys Acta. 2008; 1778:660–669.


28. Schnabel E, Anderson JM, Farquhar MG. The tight junction protein ZO-1 is concentrated along slit diaphragms of the glomerular epithelium. J Cell Biol. 1990; 111:1255–1263.


29. Kurihara H, Anderson JM, Farquhar MG. Increased Tyr phosphorylation of ZO-1 during modification of tight junctions between glomerular foot processes. Am J Physiol. 1995; 268:F514–F524.


30. Itoh M, Furuse M, Morita K, Kubota K, Saitou M, Tsukita S. Direct binding of three tight junction-associated MAGUKs, ZO-1, ZO-2, and ZO-3, with the COOH termini of claudins. J Cell Biol. 1999; 147:1351–1363.


31. Luimula P, Ahola H, Wang SX, Solin ML, Aaltonen P, Tikkanen I, et al. Nephrin in experimental glomerular disease. Kidney Int. 2000; 58:1461–1468.


32. Luimula P, Sandström N, Novikov D, Holthöfer H. Podocyte-associated molecules in puromycin aminonucleoside nephrosis of the rat. Lab Invest. 2002; 82:713–718.


33. Raats CJ, van den Born J, Bakker MA, Oppers-Walgreen B, Pisa BJ, Dijkman HB, et al. Expression of agrin, dystroglycan, and utrophin in normal renal tissue and in experimental glomerulopathies. Am J Pathol. 2000; 156:1749–1765.


34. Kawachi H, Kurihara H, Topham PS, Brown D, Shia MA, Orikasa M, et al. Slit diaphragm-reactive nephritogenic MAb 5-1-6 alters expression of ZO-1 in rat podocytes. Am J Physiol. 1997; 273:F984–F993.
35. Kurihara H, Anderson JM, Kerjaschki D, Farquhar MG. The altered glomerular filtration slits seen in puromycin aminonucleoside nephrosis and protamine sulfate-treated rats contain the tight junction protein ZO-1. Am J Pathol. 1992; 141:805–816.
36. Matter K, Balda MS. Signalling to and from tight junctions. Nat Rev Mol Cell Biol. 2003; 4:225–236.

