Abstract
Purpose
The authors have observed a failure to achieve secure fixation in elderly patients when inserting a half-pin at the anteromedial surface of the tibia. The purpose of this study was to compare two methods for inserting a half-pin at tibia diaphysis in elderly patients.
Materials and Methods
Twenty cadaveric tibias were divided into Group C or V. A half-pin was inserted into the tibias of Group C via the conventional method, from the anteromedial surface to the interosseous border of the tibia diaphysis, and into the tibias of Group V via the vertical method, from the anterior border to the posterior surface at the same level. The maximum insertion torque was measured during the bicortical insertion with a torque driver. The thickness of the cortex was measured by micro-computed tomography. The relationship between the thickness of the cortex engaged and the insertion torque was investigated.
Results
The maximum insertion torque and the thickness of the cortex were significantly higher in Group V than Group C. Both groups exhibited a statistically significant linear correlation between torque and thickness by Spearman's rank correlation analysis.
Conclusion
Half-pins inserted by the vertical method achieved purchase of more cortex than those inserted by the conventional method. Considering that cortical thickness and insertion torque in Group V were significantly greater than those in Group C, we suggest that the vertical method of half-pin insertion may be an alternative to the conventional method in elderly patients.
When using an external fixator to treat an open fracture with severe tissue damage, solid fixation and its maintenance are imperative to promoting bone healing, regardless of whether the fixator is used as a temporary damage control frame or as a definitive treatment frame. However, loosening of a screw or pin is a common problem in external fixation, and this loosening can cause instability at the pin-cortex interface, as well as pin site infection.1,2,3,4,5 Osteoporosis, a frequent comorbidity in elderly patients, increases the likelihood of loosening.6 Although osteoporosis is characterized by thinner cross-linking within trabecular bone and follows a decrease in cancellous bone mineral density, cortical bone density also decreases.7 The cortical thickness of the diaphysis decreases and the medullary canal width increases; these changes can affect the fixation capacity of screws or pins because fewer threads are able to purchase the cortex.1
When fixing a tibia shaft fracture with half-pins and an external fixator, the screws are generally inserted at the anteromedial surface of the tibia and pass through the interosseous border of the tibia.8 However, the authors have observed a failure to achieve secure fixation in elderly patients when inserting a half-pin at this site. The cross-section of the tibia diaphysis is triangular, and the anterior border is generally thicker than other medial or interosseous borders (Fig. 1). Thus, the authors hypothesized that a pin inserted at the anterior border through the posterior surface of the tibia could purchase the thickest portion of cross-section and increase fixation stability, especially in osteoporotic bone. In practice, when a pin did not seem to have "purchased the cortex solidly" on insertion at the anteromedial surface of the tibia diaphysis in an elderly patient, the authors switched the insertion site from the anteromedial surface to anterior border of the tibia. Then, the authors could sense that the pins were solidly inserted and fixed. However, few studies have reported on the relationship between fixation stability and the length of the tibia diaphysis cross-section or the thickness of the cortex engaged by the pin.
The purpose of this study was to compare the insertion torque of half-pins achieved by the conventional method (inserted from the anteromedial surface to the interosseous border) with that achieved by the vertical method (from the anterior border to the posterior surface). Furthermore, the authors compared the cortical thickness engaged in each method using micro computed tomography (micro CT) and assessed the relationship between engaged cortical thickness and insertion torque. The authors hypothesized that the vertical method would achieve higher insertion torque (greater purchase of the cortex) and the cortical thickness at the anterior border and posterior surface would be greater than that at the anteromedial surface and interosseous border.
Since no information is available on the insertion torque of Schanz screws (Synthes, 5.0×120 mm; thread length=40 mm, Oberdorf, Switzerland) (Fig. 2) at the tibia, we needed to determine where the half-pin should be inserted at the mid-shaft of the tibia to compare the insertion torque by insertion site. A pilot study revealed that even on the same medial surface or anterior border of the tibia, the insertion torque and the amount of cortical thickness engaged differed according to the insertion site or level. Thus, regardless of method, the author decided to insert the screw at the same level of the mid-shaft of the tibia in each specimen. The level of the insertion site was 2 cm below the midpoint of the tibia lengthwise, a point which is also near the mid-portion of the diaphysis. Following the conventional method (Group C), the screw was inserted across the near and far cortices at the mid-portion of the anteromedial surface and perpendicular thereto, passing through the interosseous border of the tibia. In the vertical method (Group V), the screw was inserted across both cortices perpendicular to the anterior border of the tibia, also passing perpendicular to the posterior surface (Fig. 3).
In the pilot study, the effect size was 1.4 (mean difference: 70; standard deviation: 50), and 10 cases were needed to generate a statistical power of 80% with a two-sided α level of 0.05. Thus, ten fresh cadaveric tibias were used (age ranged from 65 to 84 years old; mean age, 73±6 years). None of the tibias exhibited gross deformity or had undergone previous surgical history. Specimens were stored frozen at -20℃ and thawed for 24 hours at room temperature prior to use. A 1-cm skin incision was made at the insertion site (2 cm below the midpoint of the tibia lengthwise) on the anteromedial surface of the diaphysis. Using a 3.5-mm drill bit, a pilot hole was created across the near and far cortices. A torque driver [FTD4000CN2-S (torque range, 50-400 cN·M), TOHNICHI MFG. Co., Tokyo, Japan] (Fig. 4) was used. The torque driver was assembled at the end of the 5.0-mm Schanz screw. A measurement of the maximum torque was made during the insertion of the screw into the pilot hole through the near cortex until it engaged the far cortex. Another 1-cm skin incision was made at midpoint of the tibia lengthwise (same level of the previous insertion on the anteromedial surface) on the anterior border of the tibia. Then, another pilot hole was created perpendicular to the anterior border. Maximum torque was measured in the same manner as with the conventional method. All procedures were performed by a single surgeon to maintain uniformity between experiments. Institutional Review Board approval with a waiver of informed consent was obtained.
All of specimens underwent micro CT analysis (NFR Polaris-G90MVC, Cardiac Gating Model, Jeonju, Korea). The cortical thickness at the point where the holes were created was measured and the cortical thickness (Group C, anteromedial surface and interosseous border; Group V, anterior border and posterior surface) for each group was calculated. Because creating the hole was performed prior to micro CT, the cortical thickness could not be measured on the image that included the holes. Instead, the image just proximal to the image where the holes started to appear was used (Fig. 5). To enhance the reliability in measuring the cortical thickness, two orthopaedic surgeons measured the values independently, and the intra-class correlation coefficient (ICC) for inter-observer reliability was calculated.
Statistical analysis was performed using IBM SPSS Statistics, Version 20 (IBM, New York, NY, USA). The Mann-Whitney test was used to compare the insertion torque and cortical thickness between two groups. To investigate the correlation between insertion torque and cortical thickness, Spearman's rank correlation analysis was used. The level of significance was set at p<0.05.
The value of the maximum insertion torque (mean and standard deviation) was 160.0±44.4 cN·M (range, 80-240 cN·M) in Group C and 251.7±71.9 cN·M (range, 110-360 cN·M) in Group V. There was a significant difference between the groups (p=0.001). The percentage difference between Groups C and V was 56.6±21.9% (range, 8.3-92.3%). The sum of cortical thickness (mean and standard deviation) was 4.3±1.8 mm (range, 2.4-7.8 mm; ICC=0.889) for Group C and 7.5±3.8 mm (range, 2.96-14.5 mm; ICC=0.867) for Group V. There was a significant difference between the groups (p=0.001). The percentage difference between Groups C and V was 67.8±36.3% (range, 12.5-132.1%) (Table 1).
Both groups exhibited a statistically significant linear correlation between insertion torque and cortical thickness by Spearman rank's correlation analysis (r=0.803, p<0.001, Group C; r=0.787, p<0.001, Group V).
The purposes of this study were to measure the maximum torque of the half-pin inserted at the tibia diaphysis by the conventional method (Group C) or the vertical method (Group V) and to investigate the relationship between the maximum torque and the cortical thickness at the insertion site. When applying a half-pin external fixator to the tibia, as most orthopaedic surgeons do in practice, Ziran, et al.8 indicated that the anteromedial surface is best suited for 5-mm pins, due to the absence of soft tissue and easy access at this site. Ziran, et al. also recommended that the pin be inserted perpendicular to the anteromedial surface of the tibia. This method may be convenient for surgeons because half-pins can be easily inserted perpendicular to the surface. In contrast, insertion of a pin perpendicular to the anterior border of the tibia diaphysis, as performed in this study, may be difficult, even while palpating the anterior border, considering the location of the anterolateral surface of the tibia. Also, there was a possibility for slippage of the drill tip on the anterior border (shaped apex) when making the pilot hole. Thus, before drilling, with the help of a drill guide, we made a small groove on the anterior border by tapping the drill with a mallet.
Nevertheless, as we hypothesized, the insertion torque achieved by the vertical method was significantly greater than that achieved by the conventional method; the percent difference in torque between the two methods was approximately 57%. Furthermore, the sum of the cortical thickness engaged by half-pins inserted via the vertical method was significantly greater than that for pins engaged via the conventional method; the percent difference in cortical thickness was approximately 68%. It is unclear whether these results can be applied to relatively young patients with good bone quality. However, in elderly patients with wide bone marrow canals and thinner cortex,1 these outcomes may be meaningful.
Despite recent advances in locked plating that allow for more solid fixation, it is still challenging to satisfactorily stabilize osteoporotic fractures in elderly patients. External fixation is unavoidable in cases of open fracture that require soft tissue management when internal fixations are not feasible. Although external fixation is regarded as a valuable modality of fracture treatment, owing to the advantages of minimal invasiveness, stability, and facilitation of joint mobility,9 these advantages are more difficult to realize in osteoporotic fractures. To avoid fixation failure at the bone and pin interface, which is a potential problem of external fixation, several important factors should be considered: patient-related factors, implant-related factors, and technique-related factors. Despite remarkable developments in implant design and techniques, patient-related factors, such as poor bone quality and thin cortex due to aging and osteoporosis, are still challenging to overcome. Age and osteoporosis can deteriorate bone quality and reduce cortical thickness, which can increase the chance of pin or screw loosening.10,11,12 Russo, et al.10 reported that age-related bone loss reduced cortical thickness and increased intracortical porosity even in long bones such as the tibia.
For elderly patients, the engagement of as much of the cortex as possible by a pin thread is necessary. As revealed in the current study, the amount of cortical thickness engaged and insertion torque resistance were directly related. Wikenheiser, et al.13 reported that thermal response and insertion torque resistance are related; however, this thermal damage can be minimized by either predrilling or lowering the speed of insertion.14 In this study, the half-pin was inserted by hand, so thermal damage might not be significant. However, there is a concern for "wobbling" during subsequent manual placement of half-pins.8,15 Ziran indicated that this wobbling might cause a small but meaningful conical deformation at the near cortex, which might reduce the initial stability.8 We were aware of this issue. However, in this study, we could not use a motorized power device, because we had to use the torque driver for measuring the insertion torque. This study has several limitations that should be addressed. First, this study only measured insertion torque resistance, which is only a single factor among many that determine initial stability. Second, the authors did not measure the pull-out strength, even though pin or screw loosening after external fixation may be more meaningful than the direct pull-out strength in practice. If subsequent pull-out strength had been measured, the authors could have investigated the relationship between subsequent pull-out strength and initial stability, and this study could have been substantial. Third, the authors could not measure the cortical bone mineral density due to technical issues; only cortical thickness was addressed. Thus, the severity of osteoporosis in each specimen was not determined. Fourth, we could not estimate the effect of previous pin insertion and its hole on subsequent pin insertion in terms of insertion torque, even though we thought it might be not significant. Fifth, this study did not address late pin loosening that also happens in osteoporotic bone.
In conclusion, a half-pin inserted by the vertical method achieved purchase of more cortex than that inserted by the conventional method. Considering that the cortical thickness and insertion torque in Group V were significantly greater than those of Group C in the current study, this new method may be of use as an alternative to the conventional method in elderly patients.
Figures and Tables
Fig. 1
Cross-section of the right tibia diaphysis. A, anterior border; I, interosseous border; M, medial border.
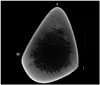
Fig. 3
Orientation of Schanz screws inserted by the conventional method and vertical method. C, conventional method, from the anteromedial surface to the interosseous border; V, vertical method, from the anterior border to the posterior surface.
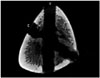
Fig. 5
Cortical thickness at the cross-section of the tibia diaphysis. A, anterior border of the tibia; M, medial border; I, interosseous border. Blue arrow: cortical thickness in Group C. Red arrow: cortical thickness in Group V.
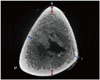
Table 1
The Maximum Insertion Torque and Sum of Cortical Thickness Engaged

Group C | Group V | p value | |
---|---|---|---|
Maximum insertion torque (cN·M) | 160.0±44.4 | 251.7±71.9 | 0.001 |
Cortical thickness (mm) | 4.3±1.8 (ICC=0.889) | 7.5±3.8 (ICC=0.867) | 0.001 |
ICC, intra-class correlation coefficient.
Group C, conventional method, engaging both cortices from the anteromedial surface to the interosseous border of the tibia diaphysis; Group V, vertical method, engaging both cortices from the anterior border to the posterior surface of the tibia diaphysis. The values present the mean and standard deviation.
ACKNOWLEDGEMENTS
The authors thank Man-Seo Cho for his assistance with the figures and research. This study was supported by a faculty research grant from the Yonsei University College of Medicine (6-2013-0046).
References
1. Giannoudis PV, Schneider E. Principles of fixation of osteoporotic fractures. J Bone Joint Surg Br. 2006; 88:1272–1278.


2. Donaldson FE, Pankaj P, Simpson AH. Bone properties affect loosening of half-pin external fixators at the pin-bone interface. Injury. 2012; 43:1764–1770.


3. Hyldahl C, Pearson S, Tepic S, Perren SM. Induction and prevention of pin loosening in external fixation: an in vivo study on sheep tibiae. J Orthop Trauma. 1991; 5:485–492.
4. Donaldson FE, Pankaj P, Simpson AH. Investigation of factors affecting loosening of Ilizarov ring-wire external fixator systems at the bone-wire interface. J Orthop Res. 2012; 30:726–732.


5. Parameswaran AD, Roberts CS, Seligson D, Voor M. Pin tract infection with contemporary external fixation: how much of a problem? J Orthop Trauma. 2003; 17:503–507.


6. Koval KJ, Meek R, Schemitsch E, Liporace F, Strauss E, Zuckerman JD. An AOA critical issue. Geriatric trauma: young ideas. J Bone Joint Surg Am. 2003; 85-A:1380–1388.
7. Seebeck J, Goldhahn J, Morlock MM, Schneider E. Mechanical behavior of screws in normal and osteoporotic bone. Osteoporos Int. 2005; 16:Suppl 2. S107–S111.


8. Ziran BH, Smith WR, Anglen JO, Tornetta P 3rd. External fixation: how to make it work. J Bone Joint Surg Am. 2007; 89:1620–1632.
9. Hoang-Kim A, Scott J, Micera G, Orsini R, Moroni A. Functional assessment in patients with osteoporotic wrist fractures treated with external fixation: a review of randomized trials. Arch Orthop Trauma Surg. 2009; 129:105–111.


10. Russo CR, Lauretani F, Seeman E, Bartali B, Bandinelli S, Di Iorio A, et al. Structural adaptations to bone loss in aging men and women. Bone. 2006; 38:112–118.


11. Currey JD. Changes in the impact energy absorption of bone with age. J Biomech. 1979; 12:459–469.


12. Donaldson FE, Pankaj P, Cooper DM, Thomas CD, Clement JG, Simpson AH. Relating age and micro-architecture with apparent-level elastic constants: a micro-finite element study of female cortical bone from the anterior femoral midshaft. Proc Inst Mech Eng H. 2011; 225:585–596.


13. Wikenheiser MA, Markel MD, Lewallen DG, Chao EY. Thermal response and torque resistance of five cortical half-pins under simulated insertion technique. J Orthop Res. 1995; 13:615–619.


14. Hutchinson DT, Bachus KN, Higgenbotham T. External fixation of the distal radius: to predrill or not to predrill. J Hand Surg Am. 2000; 25:1064–1068.


15. Oliphant BW, Kim H, Osgood GM, Golden RD, Hawks MA, Hsieh AH, et al. Predrilling does not improve the pullout strength of external fixator pins: a biomechanical study. J Orthop Trauma. 2013; 27:e25–e30.