Abstract
Purpose
Although the proteasome inhibitor known as bortezomib can modulate the inflammatory process through the nuclear factor-kappa B signaling pathway, the immunomodulatory effect of pre-incubated bortezomib has not been fully evaluated for inflammation by infectious agents. Therefore, we evaluated the effect of bortezomib on the expression of inflammatory cytokines and mediators in macrophage cell lines and on survival in a murine peritonitis sepsis model.
Materials and Methods
Bortezomib was applied 1 hr before lipopolysaccharide (LPS) stimulation in RAW 264.7 cells. The cecal ligation and puncture (CLP) experiments were performed in C57BL/6J mice.
Results
Pre-incubation with bortezomib (25 nM or 50 nM) prior to LPS (50 ng/mL or 100 ng/mL) stimulation significantly recovered the number of viable RAW 264.7 cells compared to those samples without pre-incubation. Bortezomib decreased various inflammatory cytokines as well as nitric oxide production in LPS-stimulated cells. The 7-day survival rate in mice that had received bortezomib at 0.01 mg/kg concentration 1 hr prior to CLP was significantly higher than in the mice that had only received a normal saline solution of 1 mL 1 hr prior to CLP. In addition, the administration of bortezomib at 0.01 mg/kg concentration 1 hr before CLP resulted in a significant decrease in inflammation of the lung parenchyma. Collectively, pretreatment with bortezomib showed an increase in the survival rate and changes in the levels of inflammatory mediators.
The proteasome inhibitors that halt the non-lysosomal degradation of cellular proteins through the ubiquitin-proteasome system (UPS) can modulate excessive inflammation and immune responses as well as regulate cytokine expressions produced by various stimuli.1,2,3,4 The potential role of proteasome inhibitors as a therapeutic drug has been evaluated in chronic inflammatory diseases, such as rheumatoid arthritis and inflammatory bowel disease.5,6,7 The potent anti-inflammatory effects of proteasome inhibitors have been chiefly attributed to reducing the activation of nuclear factor-kappa B (NF-κB).8,9
Bortezomib is a synthetic peptide boronate that functions by inhibiting the chymotrypsin-like activity of the 26S proteasome complex in UPS. Specifically, it inhibits this activity by forming highly selective and reversible covalent bonds with threonine in mammalian cells.9,10 In 2003, bortezomib was the first proteasome inhibitor approved by the USA Food and Drug Administration for treating multiple myeloma.9 This protease inhibitor has been successfully used mainly as a cytostatic anticancer drug for the treatment of refractory and relapsed multiple myeloma.11
Severe sepsis including septic shock represents a state of profound and uncontrolled inflammation, which results from the activation of NF-κB through intracellular signaling pathways such as Toll-like receptors.12 Many clinical trials have been performed to modulate the excessive inflammatory response in Gram-negative sepsis cases mediated by lipopolysaccharide (LPS), but no immunomodulatory drug has been shown to improve mortality in severe sepsis patients until now.13 The development of new therapeutic drugs and modalities as targets or tailored-therapies is essential to reducing the high mortality of severe sepsis.
With their role as immune modulators, proteasome inhibitors may be candidates for new therapeutic targets in the treatment of severe sepsis.14 Two previous studies using a mouse cecal ligation and puncture (CLP) model revealed that MG-132 and lactacystin (quercetin and mevinolin), in combination with the role of antibiotics as proteasome inhibitors, decreased inflammatory response and prolonged survival.15,16 However, there are no known reports discussing whether bortezomib, the only drug with clinical approval among the generated proteasome inhibitors, can have beneficial effects in cases of severe sepsis. Therefore, this study evaluated the effect of bortezomib on the expression of inflammatory cytokines and mediators in in vitro LPS-induced macrophage cell lines and on survival in a murine peritonitis sepsis model induced by CLP. The ultimate goal of this study is to present the possibility of bortezomib as a new drug for the management of severe sepsis.
The murine-macrophage-like cell line RAW 264.7, which is most commonly used in LPS-treated sepsis in in vitro experiments, was prepared.16 The RAW 264.7 cells were purchased from the Korean Cell Line Bank, Seoul, Korea and maintained at 37℃ in liquid growth media composed of Dulbecco's Modified Eagle Medium (DMEM), 10% fetal bovine serum (FBS), and penicillin (100 unit/mL) and streptomycin (100 µg/mL) (WelGENE Inc., Daegu, Korea) for all the experiments. The media included RAW 264.7 cells that were cultured in a 37℃ incubator with 5% CO2 and 95% ambient air and substituted for new compositions twice a week.
In all in vitro experiments, the RAW 264.7 cells were seeded onto the plate on day 1, and liquid growth media was changed from 10% FBS DMEM to 1% FBS DMEM on day 2. On day 3, LPS was applied at various concentrations to the growing RAW 264.7 cells 1 hr after the application of bortezomib at various concentrations. All experimental processes were repeated three times, using the same protocol each time.
Lipopolysaccharide from Escherichia coli, serotype 055:B5 was purchased from Sigma Chemical (St. Louis, MO, USA). Bortezomib (Velcade® inj, 3.5 mg/V composed of a mixture of 3.5 mg bortezomib and 35 mg mannitol) was obtained from Millennium Pharmaceuticals, Inc. (Cambridge, MA, USA). Bortezomib (1 mg) was dissolved in sterile 0.9% normal saline at a concentrations of 1 mg/mL and was then stored at -20℃ until use. For these studies, the prepared 100 µM stock solution was re-suspended in 0.9% saline.
The Proteasome-Glo™ Chymotrypsin-like Cell-Based Assay Kit (Promega Corporation, Madison, WI, USA) was used to measure the proteasome inhibition activity of bortezomib. This assay used a luminogenic proteasome substrate containing succinyl-leucine-leucine-valine-tyrosine-aminoleuciferin in a buffer optimized for cell permeabilization, proteasome and luciferase activity.17,18 Adding a single Proteasome-Glo™ Cell-Based Reagent in an "add-mix-measure" format resulted in proteasomal cleavage of the substrate and generation of a luminescent signal produced by the luciferase reaction. Following the cleavage by the proteasome, the substrate for luciferase (aminoluciferin) was released, allowing the luciferase reaction to proceed and produce light. Ten minutes after adding various substrates, the luminescence was measured using a luminometer and expressed in relative luminescent units.17,18
The quantitative index of RAW 264.7 cell viability after treatment with LPS and bortezomib of various concentrations was determined by the Cell Counting Kit-8 (CCK-8) colorimetric assay. The CCK-8 assay used highly water-soluble tetrazolium monosodium salt and WST (water soluble tetrazolium salt)-8 [2-(2-methoxy-4-nitrophenyl)-3-(4-nitrophenyl)-5-(2,4-disulfophenyl)-2H-tetrazolium, monosodium salt]. The detection sensitivity of CCK-8 was higher than that of other tetrazolium salts such as MTT, XTT, MTS, or WST-1.19,20 RAW 264.7 cells were plated in 96-well microtiter plates at a density of 8×103 cells per well. Following this, the live cell count was assayed using CCK-8 (Dojindo Molecular Technologies, Inc., Rockville, MD, USA), according to the manufacturer's protocol. In brief, 10 µL of CCK-8 solution was added to each well, and the plates were incubated in a CO2 incubator for 4 hr. Thereafter, 10 µL of 1% w/v sodium dodecyl sulfate solution was added to each well, and the plates were stored in darkness at room temperature. Finally, the absorbance (optical density) was measured at 450 nm
Total cellular RNA was extracted using a commercial easy-spin™ (DNA free) total RNA extraction kit, which minimizes the contamination of genomic DNA by combining the advantages of solution and column types (iNtRON Biotechnology Inc., Seoul, Korea).21 For the reverse transcription-polymerase chain reaction (RT-PCR) study, approximately 6×105 RAW 264.7 cells were seeded in 60 mm dishes on day 1. On day 3, the RAW 264.7 cells were harvested after 4 hr of LPS treatment. Subsequently, RNA preparation and RT-PCR were performed for various inflammatory cytokines [tumor necrosis factor-alpha (TNF-α), interferon-gamma (IFN-γ), interleukin (IL)-1β, IL-6, and IL-10] and intercellular adhesion molecule-1 (ICAM-1). The first-strand cDNA was synthesized using Moloney Murine Leukemia Virus reverse transcriptase,22 oligo dT, and specific primers of 50 pmole from 2 µg of total RNA (BIONEER Inc., Seoul, Korea). Specific murine primers, as well as amplification cycles and annealing temperatures for semi-quantitative RT-PCR experiments used in this study, are summarized in Table 1. The expression of mouse glyceraldehyde 3-phosphate dehydrogenase was monitored in all semi-quantitative RT-PCR experiments as the internal control. The semi-quantitation of the RT-PCR product was performed using the Multi Gauge version 3.0 program (Fujifilm, Tokyo, Japan).
A nitric oxide (NO) detection kit (iNtRON Biotechnology, Seoul, Korea) based on a diazotization assay (Griess method)23 was used to analyze the effect of bortezomib on the production of NO after stimulation with LPS. This kit can accurately detect the concentration of NO- by indirectly measuring nitrite (NO2-), which is the by-product of NO transformation in living cells and is based on the colorimetric change that occurs when naphthylethylenediamine is added to the by-product of the reaction between sulfanilamide and nitrite. The limit of detection is 2.0 µM nitrite. The process for determining the reference curve with the nitrite standard solution was performed in advance to ensure accurate nitrite amounts. The absorbance was measured within 20-30 minutes using a plate reader with a filter in the range of 520-560 nm. On the third day of RAW 264.7 cell growth, the supernatant of the cell line growth media, which was applied by bortezomib (0 or 50 nM) and LPS (0 or 100 ng/mL) with a 1 hr interval, was used to detect NO 16 and 24 hr post-administration of bortezomib and LPS to the RAW 264.7 cell line.
C57BL/6J mice were provided a standard laboratory diet and water ad libitum and treated in accordance with the guidelines and regulations for the Care and Use of Laboratory Animals of Yonsei University, Seoul, Korea, and the Institute of Laboratory Animal Resources Commission on Life Science National Research Council, USA. The mice were 7-8 weeks of age, weighing 25-30 g at the beginning of the experiments. In this study, bortezomib and normal saline were both administrated intraperitoneally. This animal study has been approved by the Institutional Animal Care and Use Committee of Yonsei University Health System.
The negative control mice had neither received surgery nor treatment (n=5) and had received 1 mL of normal saline 1 hr prior to the sham surgery (n=5). The positive controls for the study were mice that had received 1 mL of normal saline 1 hr before CLP surgery (n=8). To evaluate the impact of bortezomib doses on survival, each group received bortezomib at a concentration of either 0.01 mg/kg (n=8) or 0.1 mg/kg (n=8) 1 hr before CLP surgery and was compared to the positive control group. To evaluate the effect of delayed administration of bortezomib on survival, the mice (n=8) that had received bortezomib at a 0.01 mg/kg concentration 24 hr after CLP surgery were compared with the positive control group. The mice were assessed for survival up to 7 days following surgery, and mortality rates were compared between groups using survival analysis.
The mice were anesthetized with an intraperitoneal (IP) injection of a combination of 10 mg/kg (0.004 mL/10 g) of xylazine (2% Rompun inj®, Bayer Korea. Ltd., Seoul, Korea) and a 30 mg/kg (0.006 mL/10 g) solution of a 1:1 mixture of tiletamine and zolazepam (Zoletil™ 250 mg/5 mL, Virbac Korea, Seoul, Korea). The cecum was exteriorized through a midline abdominal incision approximately 1 cm in length. For the induction of mid-grade murine peritonitis sepsis, the cecum was ligated at half the distance between the distal pole and the base of the cecum with size 5.0 monofilament.24 The ante-mesenteric side of the cecum was punctured through and through using a 23-gauge needle. A scant amount of luminal content was expressed through both puncture sites to ensure patency. The cecum was returned to the abdominal cavity, and the fascia and skin incision were closed using size 6.0 monofilament and surgical staples, respectively. Topical 1% lidocaine and bacitracin were applied to the surgical site post-operatively. All animals received a single intramuscular injection of trovafloxacin (Pfizer Inc., New York, NY, USA) at a dose of 20 mg/kg and subcutaneous fluid resuscitation with 1.0 mL of normal saline immediately post-operatively. The mice were then returned to their individual cages and rewarmed using heat lamps until they regained normal posture and mobility.25 Sham-operated animals underwent the same procedure without ligation or puncture of the cecum.
All mice that were still alive 7 days after surgery were anesthetized with an IP injection of a combination of 10 mg/kg (0.004 mL/10 g) of xylazine (2% Rompun inj®, Bayer Korea Ltd., Seoul, Korea) and a 30 mg/kg (0.006 mL/10 g) solution of a 1:1 mixture of tiletamine and zolazepam (Zoletil™ 250 mg/5 mL, Virbac Korea, Seoul, Korea). The lung tissue was harvested from all mice that were alive 7 days after surgery and immediately frozen in -70℃ LN2 (liquid nitrogen) containers until homogenization. All harvested lung tissues were stained with hematoxyline and eosin and reviewed by a pathology specialist. The same pathology specialist, who was unaware of the treatment assignment for these histologic samples, evaluated the semiquantitative assessment of lung inflammation using the pulmonary inflammatory score.17 The pulmonary inflammatory score was graded from 0 to 3 using the following definition: 0=normal; 1=mild inflammation involving the peribronchial or perivascular area; 2=moderate inflammation involving less than 50% of the parenchyma, and 3=severe inflammation involving more than 50% of the parenchyma.17
Each in vitro experiment was replicated three times, and the mean values of these results were used for the statistical quantification analysis. Results were expressed as mean±standard error of the mean. The statistical differences between the mean values of the two groups were determined using the independent sample Student's t-test and the non-parametric Mann-Whitney U test. Survival analyses were performed using the Kaplan-Meier curves. All statistical analyses were performed using the Statistics Package for Social Science (SPSS 18.0 for Windows; SPSS Inc., Chicago, IL, USA). All p-values were two-tailed, and p<0.05 was considered to be statistically significant.
The concentrations of viable RAW 264.7 cells were significantly decreased after treatment with 50 and 100 ng/mL of LPS alone, without pre-incubation with bortezomib (the arrows in Fig. 1). When the cells were pre-incubated with bortezomib 1 hr prior to treatment with 50 and 100 ng/mL of LPS, both 25 and 50 nM concentrations were shown to significantly recover the concentrations of viable RAW 264.7 cells compared with no pre-incubation. However, pre-incubating the samples with higher concentrations of bortezomib (75 and 100 nM) significantly decreased the concentrations of viable RAW 264.7 cells in the samples that had received either the 50 or 100 ng/mL LPS solutions (Fig. 1).
An evaluation was performed to determine whether proteasome activity was effectively inhibited by 25 and 50 nM bortezomib, which significantly increased cell viability against the effect of LPS (Fig. 1). Bortezomib at both the 25 and 50 nM concentrations significantly decreased the proteasome activity compared with the cells that were not treated with bortezomib and LPS, irrespective of post-treatment with 100 ng/mL of LPS. Moreover, when the higher concentration (50 nM) of bortezomib was used, the proteasome activity was reduced more than when the lower dose (25 nM) of bortezomib was used (Fig. 2).
The expression of inflammatory cytokines and adhesion molecules at the cellular RNA level was evaluated using RT-PCR and the semi-quantitation method in order to reveal the effects of bortezomib on inflammatory cytokine and adhesion molecule levels in the cells stimulated by LPS. Bortezomib at 0, 25, and 50 nM concentrations was used to pre-treat cells 1 hr prior to stimulation with LPS at 0, 50, and 100 ng/mL concentrations in various combinations. TNF-α, IL-1β, and ICAM-1 were not expressed in the RAW 264.7 cells, which had received neither bortezomib nor LPS treatment. In addition, these three molecules were also not expressed when the cells were only treated with bortezomib (at either 25 or 50 nM concentrations), without LPS stimulation. IL-6 and IL-10 were expressed in the pure RAW 264.7 cells but not significantly detected in the cells treated only with bortezomib (25 or 50 nM). However, IFN-γ was expressed without a significant difference, irrespective of the concentrations of bortezomib and LPS used (Fig. 3A). Therefore, we did not perform the semi-quantitation test for IFN-γ.
The results for the semi-quantitation are shown in Fig. 3B-F. The levels of TNF-α were shown to decrease with a statistically significant difference only in the cells pretreated with 50 nM of bortezomib before the application of LPS at both 50 and 100 ng/mL concentrations, compared with the cells that were not pretreated with bortezomib before stimulation by LPS at the same concentrations. Bortezomib at the lower dose of 25 nM did not have the same effect on TNF-α decline. IL-1β and IL-6 expression levels were significantly decreased in the cells that had been pretreated with bortezomib at either a 25 or 50 nM concentration before the application of LPS at either a 50 or 100 ng/mL concentration, compared with the cells that were not pretreated with bortezomib before the stimulation by LPS of the same concentrations. Bortezomib at the higher concentration of 50 nM showed a greater decrease in IL-1β than the 25 nM bortezomib concentration before stimulation by LPS at either the 50 or 100 ng/mL concentration. However, the decreases in IL-6 levels were similar, irrespective of the concentrations of bortezomib and LPS.
The expression levels of IL-10 were significantly decreased in the cells that were pretreated with either a 25 or 50 nM concentration of bortezomib before the application of LPS at a 50 ng/mL concentration, compared with the cells that had not been pretreated with bortezomib before stimulation by LPS using the same concentrations. In the cells that received the high concentration of LPS (100 ng/mL), pre-incubation using the higher concentration of bortezomib (50 nM) significantly decreased the expression of IL-10. With respect to ICAM-1, the cells stimulated by the higher concentration of LPS (100 ng/mL) expressed significantly decreased levels of ICAM-1 when pre-incubated with either 25 or 50 nM of bortezomib. However, the cells that had been stimulated by the lower dose of LPS (50 ng/mL) expressed significantly decreased levels of ICAM-1 when pre-incubated with only the lower dose of bortezomib (25 nM).
There was a six-fold increase in NO production in the RAW 264.7 cells both 16 and 24 hr post-treatment with the administration of only LPS at a 100 ng/mL concentration. When the cells were pre-treated with a 50 nM concentration of bortezomib prior to the application of LPS (100 ng/mL), NO levels were significantly lower than in cells treated with LPS alone but were higher than the negative control RAW 264.7 cells that had not been treated with bortezomib or LPS (Fig. 4).
In the negative control groups, all mice were alive until 7 days after surgery. The 7-day survival rate in mice that were pre-treated with bortezomib at a concentration of 0.01 mg/kg 1 hr before CLP surgery was significantly higher than in the positive control group (the mice that received normal saline at a 1 mL concentration 1 hr before CLP surgery) (p=0.006) (Fig. 5A). However, the 7-day survival rate between the mice that had received bortezomib at a 0.1 mg/kg concentration 1 hr before CLP surgery and the positive control was not significantly different (p=0.661) (Fig. 5B).
The delayed administration of bortezomib at a 0.01 mg/kg concentration 24 hr after CLP surgery produced a higher 7-day survival rate than in the positive control, with borderline statistical significance (p=0.096) (Fig. 5C).
In H&E staining, the lungs of mice that had received bortezomib at the 0.01 mg/kg concentration 1 hr before CLP surgery showed minimal infiltration of inflammatory cells in the pulmonary parenchyma. This observation was similar to that seen in the lungs of mice that had received the normal saline solution of 1 mL 1 hr before sham surgery (Fig. 6B and D). However, the inflammation in mice that received the bortezomib at a 0.1 mg/kg concentration 1 hr before CLP surgery was greater than in the mice that had received bortezomib at a 0.01 mg/kg concentration 1 hr before CLP surgery (Fig. 6D and E). The lung parenchyma in mice that had received bortezomib at the 0.01 mg/kg concentration 24 hr after CLP surgery revealed an increase in inflammatory cell infiltration compared to mice that received bortezomib at the 0.01 mg/kg concentration 1 hr before CLP surgery (Fig. 6D and F). However, decreased infiltration was revealed in mice that received bortezomib at the 0.1 mg/kg concentration 24 hr after CLP surgery compared to mice that received bortezomib at the 0.1 mg/kg concentration 1 hr before CLP surgery (Fig. 6E and F).
In the objective semi-quantitative analysis, the mice groups which had received bortezomib at a 0.01 mg/kg concentration both 1 hr before and 24 hr after CLP surgery (pulmonary inflammatory score of 1 and 1.5, respectively) had a significantly decreased mean pulmonary inflammatory score than that of the mice groups which had received normal saline at a 1 mL concentration 1 hr before CLP surgery (positive control group, Fig. 6C, pulmonary inflammatory score of 2.5) (1 vs. 2.5, p=0.015; 1.5 vs. 2.5, p=0.035, respectively).
The principal pro-inflammatory mediators in the pathophysiology of sepsis are TNF-α and IL-1β, which activate NF-κB by triggering a signaling pathway that leads to the phosphorylation and consequent degradation of the inhibitor κBα (IκBα).26,27 The degradation of IκBα exposes a nuclear localization signal on the NF-κB protein, which then moves into the nucleus and stimulates the transcription of specific genes. The overproduction of pro-inflammatory mediators enhances adhesion molecules and also leads to deleterious effects associated with multiple organ failure and shock.28
The UPS is the major proteolytic mechanism for non-lysosomal degradation of cellular proteins.10 UPS plays a role in turnover and quality control of proteins,29 cell cycle and apoptosis,30,31 transcription and cell signaling,32-35 immune response and antigen presentation,36 and inflammation and development.37,38 Bortezomib, a peptide-boronic acid analog, has been shown to have potent inhibitory activity at the chymotrypsin-like subunit in the 20S core of proteasomes.39 It is the first drug of its kind that has received accelerated approval for the treatment of relapsed or refractory multiple myeloma.39 A significant decrease in the level of NF-κB, resulting in decreased production of inflammatory cytokines by the cell after treatment with bortezomib, has been noted by several studies.40,41 This effect results in the lack of degradation of the inhibitor of kappa-B light polypeptide gene enhancer (IκB) protein.39 In addition, protein processing and degradation is halted by the proteasome inhibitor, resulting in a decrease in cell adhesion molecule expression and anti-apoptotic protein expression and ultimately resulting in increased apoptosis.39 It was reported that bortezomib significantly inhibited acute lethal graft-versus-host disease induction in association with a significant decrease in TNF-α, IL-1, and IL-6.42 Additionally, bortezomib induced the selective depletion of human alloreactive T lymphocytes while decreasing the production of Th1 cytokines (IFN-γ and IL-2) in vitro.43
Anti-cytokine or anti-inflammatory treatments in severe sepsis should be tailored based on the patient's immune status. Severe septic patients may have an initial robust hyper-inflammatory response. Death may occur due to a hyper-inflammatory state, and anti-inflammatory treatments may improve the likelihood of survival.26 Numerous therapies that block pro-inflammatory cytokines (e.g., anti-TNF-α, TNF-α receptor, and IL-I receptor antagonists) have failed, perhaps because the approaches are narrowly focused and the pathways are redundant, or because cytokines are critical to host defense and their blockade is excessively immunosuppressive.44 Measurements of circulating concentrations of inflammatory mediators may prove to be useful in evaluating the stage of sepsis and in tailoring the administration of anti-inflammatory agents. However, anti-inflammatory agents used during the hypo-immune phase may worsen outcomes. When patients are shown to be in a hypo-immune state, inflammatory strategies that enhance the function of the innate or adaptive immune system may be efficacious.26
Because the proteasome complex regulates the LPS-induced signal transduction, and the proteasome inhibitors block the LPS-induced proteasome's chymotrypsin activity as well as macrophage TNF-α secretion and the expression of multiple inflammatory mediator genes, proteasome inhibitors may be an important therapeutic target in Gram-negative sepsis and septic shock.14,45 Safránek, et al. reported that CLP induced significantly increased plasma levels of IL-1, TNF-α, IL-6, and IL-10, but treatment with the proteasome inhibitor MG-132 resulted in less of an increase in IL-1, TNF-α, and IL-10 levels and prolonged survival.15 In a previous study, a proteasome inhibitor was administrated 3 hr before or after the induction of sepsis (CLP surgery).15 Several studies revealed that proteasome inhibition resulted in a decrease in several of the LPS-induced pro-inflammatory cytokines and adhesion molecules in vitro and prevented LPS-induced shock in mice. In most of these studies, the proteasome inhibitors were pretreated in macrophages or mice before the LPS challenge.14,15,45 However, the protective effect of proteasome inhibitors in sepsis may not be uniformly applied to various immune states or various time differences after the development of sepsis or multiple organ dysfunctions in human beings. The principal aim of the present study was to test whether the administration of the proteasome inhibitor bortezomib could modulate the expression of pro-inflammatory cytokines and mortality in a murine sepsis model induced by CLP surgery.
This study revealed that bortezomib decreased the levels of inflammatory cytokines TNF-α, IL-1β, IL-6, and IL-10 and ICAM-1 in LPS-stimulated cells of an in vitro Gram-negative sepsis experiment. In addition, pre-treatment with bortezomib decreased NO production. Our results suggest that bortezomib can reduce the uncontrolled exaggerated inflammatory process. The above data were supported by the cell viability assay and the measurement of proteasome activity. Bortezomib at both 25 and 50 nM concentrations significantly recovered the counts of viable cells in which the inflammatory process was affected by LPS.
In contrast, bortezomib at the higher concentrations of 75 and 100 nM decreased the cell viability of LPS-stimulated cells compared to cells that had not been pre-incubated with bortezomib. This effect may have been caused by the direct cellular toxicity of bortezomib. Proteasome inhibitors such as bortezomib are known to be direct inducers of apoptosis and inhibitors of cell proliferation in both tumor and normal cells.10 In addition, they can cause different cellular effects through the inhibition of proteasomes, based on the dose of the proteasome inhibitor used.46
In the bioluminescent proteasome assay, bortezomib at both 25 and 50 nM concentrations did not completely inhibit chymotrypsin-like activity. Therefore, these concentrations of bortezomib could not sufficiently recover the count of viable cells to similar levels as the cells that had not received LPS stimulation. However, because of the toxicity in higher doses of bortezomib, we evaluated the effect of bortezomib on the expression of inflammatory cytokine using the lower concentrations of 25 and 50 nM.
One limitation of this study was that the histopathologic examination to compare the degree of pulmonary inflammation was only performed in the harvested lung tissue 7 days after sepsis development. Therefore, further study is warranted to confirm the degree of organ failure in liver, kidney, lung, and spleen tissues immediately and shortly after the development of sepsis. Furthermore, studies are needed on combination treatments with bortezomib and other immunomodulating drugs including statin and macrolide or antibiotics, as well as other doses and time administrations. Such studies might reveal that the delayed administration of bortezomib after sepsis pathogenesis initiation has a significant therapeutic implication in clinical settings, because most severe sepsis patients present to the hospital after the initiation of an advanced hyper-immune septic process.
We revealed that the pre-incubation of bortezomib 1 hr before LPS administration decreased important pro-inflammatory cytokine levels, and pre-treatment with bortezomib 1 hr before CLP surgery ameliorated survival in the mouse peritonitis sepsis model. In conclusion, our study suggests the possibility of pre-treatment with bortezomib as a new therapeutic modality in severe sepsis.
Figures and Tables
Fig. 1
The concentrations of viable RAW 264.7 cells measured using the CCK-8 assay to evaluate the effect of pre-incubation with bortezomib 1 hr before LPS treatment on the cell proliferation. On experiment day 3, LPS at 50 ng/mL (A) and 100 ng/mL (B) was administered to the growing RAW 264.7 cell lines of 8×103 cells/mL at 1 hr after the application of bortezomib with various concentrations from 0 to 100 nM. Arrows (↓) indicate the reference values in samples that had received only LPS application with 50 and 100 ng/mL, without pre-incubation with bortezomib. *Statistical significance with p-values less than 0.05 when compared with reference values (LPS alone, arrows). CCK-8, Cell Counting Kit-8; LPS, lipopolysaccharide; OD, optical density.
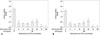
Fig. 2
The chymotrypsin-like activity of proteasomes measured by the bioluminescent proteasome assay. RAW 264.7 cells (arrow) that were not treated with bortezomib and lipopolysaccharide (100 ng/mL) were used as the reference control to determine statistical significance (*p<0.01). †Means statistically significant with a p-value less than 0.01 when compared with bortezomib at a 25 nM concentration (asterisk). The results are reported as mean±standard error of the mean of three independent experiments in triplicate. RLU, relative luminescent units; LPS, lipopolysaccharide; Bor, bortezomib.
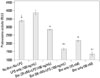
Fig. 3
The expression levels of inflammatory cytokines and intercellular adhesion molecules based on the administration of bortezomib and LPS at various concentrations. (A) The RT-PCR products expressed by agarose gel electrophoresis. (B-F) The relative expression rate of RT-PCR products by internal control using mouse GAPDH in each cytokine and ICAM-1. These semi-quantitation was performed using the Multi Guage Version 3.0 program. *Statistical significance with p-values less than 0.01. **Statistical significance with p-values less than 0.001. †,‡Reference values for statistical comparison. GAPDH, glyceraldehyde 3-phosphate dehydrogenase; TNF-α, tumor necrosis factor-alpha; IFN-α, interferon-gamma; IL, interleukin; ICAM, intercellular adhesion molecule; LPS, lipopolysaccharide.
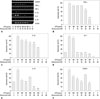
Fig. 4
The measurement of nitric oxide concentrations using the diazotization assay (Griess method) in order to analyze the effect of bortezomib on nitric oxide production after LPS stimulation. On the third day of RAW 264.7 cell growth, the supernatant of the cell line growth media received bortezomib (0 or 50 nM) and then LPS (0 or 100 ng/mL) with a 1 hr interval. These samples were used for the nitric oxide detection assay 16 and 24 hr after administration of bortezomib and/or LPS to the cell lines. *p-values less than 0.001 compared with the LPS-only treatment group for the 16 and 24 hr time periods. LPS, lipopolysaccharide; Bor, bortezomib.
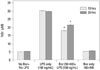
Fig. 5
Comparison of 7-day mortalities after CLP surgery by the Kaplan-Meier survival curve. Bortezomib and normal saline (at 1 mL) were always injected intraperitoneally. CLP, cecal ligation and puncture; NS, normal saline; Bor, bortezomib.
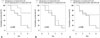
Fig. 6
Histologic findings of lung tissue after H&E staining (×200). All lung tissues were harvested from the mice that were alive at 7 days after the surgery. (A) Normal mice (negative control). (B) Normal saline 1 mL injection IP at 1 hr before sham surgery (negative control). (C) Normal saline 1 mL injection IP at 1 hr before CLP surgery (positive control). (D) Bortezomib 0.01 mg/kg injection IP at 1 hr before CLP surgery. (E) Bortezomib 0.1 mg/kg injection, IP at 1 hr before CLP surgery. (F) Bortezomib 0.01 mg/kg injection, IP at 24 hr after CLP surgery. IP, intraperitoneal; CLP, cecal ligation and puncture.
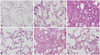
Table 1
Primers and Methods for Reverse-Transcription Polymerase Chain Reaction Used in This Study
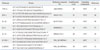
ACKNOWLEDGEMENTS
This study was supported by a faculty research grant of Yonsei University College of Medicine for 2010 (6-2010-0073).
References
1. Mao X, Pan X, Cheng T, Zhang X. Therapeutic potential of the proteasome inhibitor Bortezomib on titanium particle-induced inflammation in a murine model. Inflammation. 2012; 35:905–912.


2. Koca SS, Ozgen M, Dagli F, Tuzcu M, Ozercan IH, Sahin K, et al. Proteasome inhibition prevents development of experimental dermal fibrosis. Inflammation. 2012; 35:810–817.


3. Yannaki E, Papadopoulou A, Athanasiou E, Kaloyannidis P, Paraskeva A, Bougiouklis D, et al. The proteasome inhibitor bortezomib drastically affects inflammation and bone disease in adjuvant-induced arthritis in rats. Arthritis Rheum. 2010; 62:3277–3288.


4. Blanco B, Sánchez-Abarca LI, Caballero-Velázquez T, Santamaría C, Inogés S, Pérez-Simón JA. Depletion of alloreactive T-cells in vitro using the proteasome inhibitor bortezomib preserves the immune response against pathogens. Leuk Res. 2011; 35:1412–1415.


5. Brun J. Proteasome inhibition as a novel therapy in treating rheumatoid arthritis. Med Hypotheses. 2008; 71:65–72.


6. Inoue S, Nakase H, Matsuura M, Mikami S, Ueno S, Uza N, et al. The effect of proteasome inhibitor MG132 on experimental inflammatory bowel disease. Clin Exp Immunol. 2009; 156:172–182.


7. Lee SW, Kim JH, Park YB, Lee SK. Bortezomib attenuates murine collagen-induced arthritis. Ann Rheum Dis. 2009; 68:1761–1767.


8. Palombella VJ, Rando OJ, Goldberg AL, Maniatis T. The ubiquitin-proteasome pathway is required for processing the NF-kappa B1 precursor protein and the activation of NF-kappa B. Cell. 1994; 78:773–785.


10. Meiners S, Ludwig A, Stangl V, Stangl K. Proteasome inhibitors: poisons and remedies. Med Res Rev. 2008; 28:309–327.


11. Moehler T, Goldschmidt H. Therapy of relapsed and refractory multiple myeloma. Recent Results Cancer Res. 2011; 183:239–271.


12. Tsujimoto H, Ono S, Efron PA, Scumpia PO, Moldawer LL, Mochizuki H. Role of Toll-like receptors in the development of sepsis. Shock. 2008; 29:315–321.


13. Christaki E, Anyfanti P, Opal SM. Immunomodulatory therapy for sepsis: an update. Expert Rev Anti Infect Ther. 2011; 9:1013–1033.


14. Qureshi N, Perera PY, Shen J, Zhang G, Lenschat A, Splitter G, et al. The proteasome as a lipopolysaccharide-binding protein in macrophages: differential effects of proteasome inhibition on lipopolysaccharide-induced signaling events. J Immunol. 2003; 171:1515–1525.


15. Safránek R, Ishibashi N, Oka Y, Ozasa H, Shirouzu K, Holecek M. Modulation of inflammatory response in sepsis by proteasome inhibition. Int J Exp Pathol. 2006; 87:369–372.


16. Reis J, Tan X, Yang R, Rockwell CE, Papasian CJ, Vogel SN, et al. A combination of proteasome inhibitors and antibiotics prevents lethality in a septic shock model. Innate Immun. 2008; 14:319–329.


17. Jeong SJ, Lim BJ, Park S, Choi D, Kim HW, Ku NS, et al. The effect of sRAGE-Fc fusion protein attenuates inflammation and decreases mortality in a murine cecal ligation and puncture model. Inflamm Res. 2012; 61:1211–1218.


18. O'Brien MA, Moravec RA, Riss TL, Bulleit RF. Homogeneous, bioluminescent proteasome assays. Methods Mol Biol. 2008; 414:163–181.
19. Ishiyama M, Miyazono Y, Sasamoto K, Ohkura Y, Ueno K. A highly water-soluble disulfonated tetrazolium salt as a chromogenic indicator for NADH as well as cell viability. Talanta. 1997; 44:1299–1305.


20. Isobe I, Michikawa M, Yanagisawa K. Enhancement of MTT, a tetrazolium salt, exocytosis by amyloid beta-protein and chloroquine in cultured rat astrocytes. Neurosci Lett. 1999; 266:129–132.


21. Lee JW, Choi CH, Choi JJ, Park YA, Kim SJ, Hwang SY, et al. Altered MicroRNA expression in cervical carcinomas. Clin Cancer Res. 2008; 14:2535–2542.


22. Kotewicz ML, D'Alessio JM, Driftmier KM, Blodgett KP, Gerard GF. Cloning and overexpression of Moloney murine leukemia virus reverse transcriptase in Escherichia coli. Gene. 1985; 35:249–258.


23. Tsikas D. Analysis of nitrite and nitrate in biological fluids by assays based on the Griess reaction: appraisal of the Griess reaction in the L-arginine/nitric oxide area of research. J Chromatogr B Analyt Technol Biomed Life Sci. 2007; 851:51–70.


24. Rittirsch D, Huber-Lang MS, Flierl MA, Ward PA. Immunodesign of experimental sepsis by cecal ligation and puncture. Nat Protoc. 2009; 4:31–36.


25. Lutterloh EC, Opal SM, Pittman DD, Keith JC Jr, Tan XY, Clancy BM, et al. Inhibition of the RAGE products increases survival in experimental models of severe sepsis and systemic infection. Crit Care. 2007; 11:R122.


26. Hotchkiss RS, Karl IE. The pathophysiology and treatment of sepsis. N Engl J Med. 2003; 348:138–150.


27. Alberts B, Johnson A, Lewis J, Raff M, Roberts K, Walter P. Molecular Biology of the Cell. 4th ed. New York: Garland Science;2002.
28. Thijs A, Thijs LG. Pathogenesis of renal failure in sepsis. Kidney Int Suppl. 1998; 66:S34–S37.
29. Goldberg AL. Protein degradation and protection against misfolded or damaged proteins. Nature. 2003; 426:895–899.


30. Naujokat C, Hoffmann S. Role and function of the 26S proteasome in proliferation and apoptosis. Lab Invest. 2002; 82:965–980.


31. Jesenberger V, Jentsch S. Deadly encounter: ubiquitin meets apoptosis. Nat Rev Mol Cell Biol. 2002; 3:112–121.


32. Wang T. The 26S proteasome system in the signaling pathways of TGF-beta superfamily. Front Biosci. 2003; 8:d1109–d1127.


33. Wojcikiewicz RJ. Regulated ubiquitination of proteins in GPCR-initiated signaling pathways. Trends Pharmacol Sci. 2004; 25:35–41.


34. Muratani M, Tansey WP. How the ubiquitin-proteasome system controls transcription. Nat Rev Mol Cell Biol. 2003; 4:192–201.


35. Fuchs SY. The role of ubiquitin-proteasome pathway in oncogenic signaling. Cancer Biol Ther. 2002; 1:337–341.
36. Kloetzel PM, Ossendorp F. Proteasome and peptidase function in MHC-class-I-mediated antigen presentation. Curr Opin Immunol. 2004; 16:76–81.


37. Bowerman B, Kurz T. Degrade to create: developmental requirements for ubiquitin-mediated proteolysis during early C. elegans embryogenesis. Development. 2006; 133:773–784.


38. Elliott PJ, Zollner TM, Boehncke WH. Proteasome inhibition: a new anti-inflammatory strategy. J Mol Med (Berl). 2003; 81:235–245.


39. Mattingly LH, Gault RA, Murphy WJ. Use of systemic proteasome inhibition as an immune-modulating agent in disease. Endocr Metab Immune Disord Drug Targets. 2007; 7:29–34.


40. Amiri KI, Horton LW, LaFleur BJ, Sosman JA, Richmond A. Augmenting chemosensitivity of malignant melanoma tumors via proteasome inhibition: implication for bortezomib (VELCADE, PS-341) as a therapeutic agent for malignant melanoma. Cancer Res. 2004; 64:4912–4918.


41. Fujioka S, Schmidt C, Sclabas GM, Li Z, Pelicano H, Peng B, et al. Stabilization of p53 is a novel mechanism for proapoptotic function of NF-kappaB. J Biol Chem. 2004; 279:27549–27559.


42. Sun K, Wilkins DE, Anver MR, Sayers TJ, Panoskaltsis-Mortari A, Blazar BR, et al. Differential effects of proteasome inhibition by bortezomib on murine acute graft-versus-host disease (GVHD): delayed administration of bortezomib results in increased GVHD-dependent gastrointestinal toxicity. Blood. 2005; 106:3293–3299.


43. Blanco B, Pérez-Simón JA, Sánchez-Abarca LI, Carvajal-Vergara X, Mateos J, Vidriales B, et al. Bortezomib induces selective depletion of alloreactive T lymphocytes and decreases the production of Th1 cytokines. Blood. 2006; 107:3575–3583.


45. Shen J, Reis J, Morrison DC, Papasian C, Raghavakaimal S, Kolbert C, et al. Key inflammatory signaling pathways are regulated by the proteasome. Shock. 2006; 25:472–484.


46. Meiners S, Ludwig A, Lorenz M, Dreger H, Baumann G, Stangl V, et al. Nontoxic proteasome inhibition activates a protective antioxidant defense response in endothelial cells. Free Radic Biol Med. 2006; 40:2232–2241.


47. Hassan SW, Doody KM, Hardy S, Uetani N, Cournoyer D, Tremblay ML. Increased susceptibility to dextran sulfate sodium induced colitis in the T cell protein tyrosine phosphatase heterozygous mouse. PLoS One. 2010; 5:e8868.


48. Gray PW, Goeddel DV. Cloning and expression of murine immune interferon cDNA. Proc Natl Acad Sci U S A. 1983; 80:5842–5846.


49. Shaftel SS, Kyrkanides S, Olschowka JA, Miller JN, Johnson RE, O'Banion MK. Sustained hippocampal IL-1 beta overexpression mediates chronic neuroinflammation and ameliorates Alzheimer plaque pathology. J Clin Invest. 2007; 117:1595–1604.


50. Moore KW, Vieira P, Fiorentino DF, Trounstine ML, Khan TA, Mosmann TR. Homology of cytokine synthesis inhibitory factor (IL-10) to the Epstein-Barr virus gene BCRFI. Science. 1990; 248:1230–1234.

