Abstract
Purpose
Spinal cord injury (SCI) is associated with permanent neurological damage, and treatment thereof with a single modality often does not provide sufficient therapeutic outcomes. Therefore, a strategy that combines two or more techniques might show better therapeutic effects.
Materials and Methods
In this study, we designed a combined treatment strategy based on neural stem cells (NSCs) introduced via a neuronal cell type-inducible transgene expression system (NSE::) controlled by a neuron-specific enolase (NSE) promoter to maximize therapeutic efficiency and neuronal differentiation. The luciferase gene was chosen to confirm whether this combined system was working properly prior to using a therapeutic gene. The luciferase expression levels of NSCs introduced via the neuronal cell type-inducible luciferase expression system (NSE::Luci) or via a general luciferase expressing system (SV::Luci) were measured and compared in vitro and in vivo.
Results
NSCs introduced via the neuronal cell type-inducible luciferase expressing system (NSE::Luci-NSCs) showed a high level of luciferase expression, compared to NSCs introduced via a general luciferase expressing system (SV::Luci-NSCs). Interestingly, the luciferase expression level of NSE::Luci-NSCs increased greatly after differentiation into neurons.
Spinal cord injury (SCI) is often associated with permanent disability as a result of sensory or motor functional defects caused by neuronal death and demyelination after primary physical damage.1 Treatment with a single modality is unlikely to result in complete recovery. Therefore, combined treatment might be effective for improving therapeutic efficacy. In the field of gene therapy, therapeutic gene expression controlled by hypoxia-specific or tissue-specific gene expression systems offers a possibility for efficient and safe gene therapy.23456
In the stem cell therapy field, transplantation with neural stem cells (NSCs) showed therapeutic potential in animal models with incurable disorders, including SCI.78 Interestingly, it has been reported that stem cells can be used as a gene delivery system9 and that stem cell-mediated gene therapy results in a better therapeutic outcome and more stable therapeutic gene delivery than single therapy with stem cells or therapeutic genes alone.1011121314
Considering that most stem cell therapies for SCI have used NSCs or neural precursor cells, which have high neuronal differentiation potentials, we chose a neuronal cell type-inducible transgene expression system (NSE::) for transgene overexpression in NSCs. We assumed that a combined treatment strategy based on NSCs and an transgene expression system controlled by a neuron-specific enolase (NSE) promoter would increase therapeutic efficiency. Finally, we demonstrated that it is suitable with NSCs for the establishment of a gene and NSC therapy platform.
To confirm whether the NSE was suitable with NSCs for this combined treatment strategy, both a neuronal cell-type-inducible luciferase expression system (NSE::Luci) and a general luciferase expression system (SV::Luci) were made by pGL3 luciferase reporter vectors. SV::Luci was purchased from Promega (Madison, WI, USA, Cat.no E1761). Neuron-specific enolase (NSE) promoter was provided by Prof. Lee at Hanyang University, Seoul, Korea. The NSE region was inserted between NheI and BglII sites of the pGL3-basic vector (Promega, Cat.no E1751). The construction designs of the plasmid are shown in Fig. 1.
We used mouse NSCs in this study. NSCs (CRL-2925, ATCC, Manassas, VA, USA) were cultured in dulbecco's modified eagle medium (DMEM)/F12 and supplemented with 10% fetal bovine serum (FBS; Thermo Scientific, Waltham, MA, USA) and 1% penicillin and streptomycin (Invitrogen, Carlsbad, CA, USA). Approximately 4×105 cells were plated in six-well culture dishes (4×104 cells/cm2) and incubated at 37℃ in a humidified atmosphere containing 5% CO2.
For the other experiment, we used the human embryonic kidney 293 cell (293FT) provided from Prof. Kang at Yonsei University. Cells were cultured in DMEM supplemented with 10% FBS, 1% penicillin and streptomycin, 1X non-essential amino acid (NEAA; Invitrogen), and 1X sodium pyruvate (Invitrogen). Approximately 6×105 cells were plated in six-well culture dishes (6×104 cells/cm2) and incubated at 37℃ in a humidified atmosphere containing 5% CO2.
Transfection was performed using Lipofectamine 2000 (Invitrogen) as previously described.9 Briefly, NSE::Luci, SV::Luci, or SV::DsRed (Control) plasmid (each 4 µg) were individually mixed with serum-free and antibiotic-free DMEM/F12 medium. Lipofectamine (10 µL) also was mixed with serum-free and antibiotic-free DMEM/F12 medium. Plasmid DNA solution was mixed with the lipofectamine-DMEM mixture and then incubated for 20 min at room temperature (RT). The DNA-lipofectamine mixture was added to NSCs or 293FT cells, and the cells were incubated at 37℃ in a humidified atmosphere containing 5% CO2.
For neural differentiation, NSCs were cultured in presence of retinoic acid (RA; Sigma, St. Louis, MO, USA) for 7 days. RA was dissolved in dimethyl sulfoxide (DMSO) (working solution, 10 mM) and diluted with culture media (final concentration, 0.01 µM, 0.1 µM, and 1 µM). Briefly, NSCs were seeded on poly-l-lysine (2 µg/cm2, Sigma)-coated glass coverslips at a density of 2×104 cells/cm2. After 24-h incubation, the medium was replaced with a complete medium with or without RA and then changed every 2 days. Neural differentiation was carried out for 7 days.
All protocols were approved by the Animal Care and Use Committee of the Medical Research Institute at Yonsei University College of Medicine. All experiments were conducted in accordance with international guidelines on the ethical use of animals, and the number of animals used was minimized. For anesthesia, ICR mice (male, 6 weeks, 30 g, OrientBio, Seongnam, Gyeonggi-do, Korea) were intraperitoneally injected with ketamine (100 mg/kg, Yuhan, Seoul, Korea) and rompun (10 mg/kg, Bayer Korea, Seoul, Korea). Laminectomy was performed at thoracic level 11. SCI was carried out using self-closing forceps (compression injury for 10 sec). Animals were divided into two groups, those treated with SV::Luci-NSCs and those treated with NSE::Luci-NSCs. NSCs (5×105 cells/2 µL) were directly injected into the spinal cord using a microinjection pump (injection rate, 1 µL/min). After transplantation, the exposure site was closed using suture wound clips. Cyclosporin A (10 mg/kg, Chong Kun Dang, Seoul, Korea) and cefazolin (20 mg/kg, Yuhan) were intraperitoneally injected daily.
The In Vivo Imaging System (IVIS) Spectrum (Xenogen, Alameda, CA, USA) was used to detect luciferase expression. For in vivo imaging, mice were anesthetized with ketamine (100 mg/kg) and rompun (10 mg/kg), and then the substrate D-luciferin (150 mg/kg) was injected intraperitoneally. Twenty minutes after injection, bioluminescence images were captured for 10 min. Regions of interest (ROIs) were analyzed, and total quantification of bioluminescence was quantified using Living Image® (Xenogen) software. For in vitro imaging, the substrate D-luciferin (150 µg/mL) was added to the SV::DsRed-NSCs (Control), SV::Luci-NSCs, or NSE::Luci-NSCs in medium, followed by mounting and bioluminescence detection for 2-3 min.
A luciferase assay was performed 24-48 hrs after gene transfection or cell transplantation, and each sample (cells or tissues) were lysed with Pro-prep™ (INTRON Biotechnology, Gyeonggi, Korea). The concentration of protein in lysates was measured using a bicinchoninic acid protein assay kit (Pierce, Iselin, NJ, USA), following the manufacturer's protocol. Luciferase expression was measured using a luminometer for 10 s. The level of luciferase expression was expressed in relative light units (RLU) per mg of protein.
Differentiated cells were fixed in 4% paraformaldehyde, pH 7.4 (Merck, Darmstadt, Germany), for 10-15 min at RT. Samples were washed with phosphate buffered saline (PBS, Thermo) three times. Blocking was performed with 10% normal donkey serum in PBS containing 0.3% Triton X-100 for 60 min at RT. Primary antibodies against beta-III-Tubulin (TUJ1), microtubule-associated protein 2 (MAP2), neural cell adhesion molecule (NCAM), and neurofilament (NF) were incubated overnight at 4℃. Samples were washed three times with PBS, and secondary antibodies were added and incubated for 1 h at RT. Samples were washed three times with PBS, and 4',6'-diamino-2-phenylinodole (DAPI; Vector Labs, Burlingame, CA, USA) was added. Samples were covered with glass coverslips. A confocal laser scanning microscope (LSM 700, Zeiss, Oberkochen, Germany) was used for analysis.
Statistical analysis was performed using Prism 6 (Graphpad, San Diego, CA, USA). All data were evaluated using Student's t-test and a one-way analysis of variance (ANOVA). All data are expressed as the mean±standard error of the mean. All p-values<0.05 were considered statistically significant.
NSE::Luci was constructed using NSE promoter and pGL3-basic plasmid (Fig. 1). To confirm whether this neuronal cell-type-inducible gene overexpression system is suitable for NSCs, we investigated the expression level of luciferase in NSCs transfected with SV::DsRed (Control), SV::Luci plasmid, and NSE::Luci plasmid. Based on the results of IVIS and the luciferase assay, we confirmed that luciferase expression significantly increased in NSE::Luci-NSCs, compared with SV::Luci-NSCs (Fig. 2). These results indicate that the neuronal cell type-inducible transgene overexpression system is suitable for NSCs. To confirm whether the transgene overexpression pattern can be sustained after hypoxic injury (mimicking tissue ischemia after SCI), we investigated the luciferase expression level of Control, SV::Luci-NSCs, and NSE::Luci-NSCs after hypoxic injury for 24 h. We confirmed that the luciferase expression level significantly increased in NSE::Luci-NSCs, compared with that in SV::Luci-NSCs (Fig. 2). These results indicate that combined therapy with NSCs and a neuronal cell type-inducible gene overexpression system can be applied to spinal cord injuries that cause tissue ischemia.
To confirm whether the NSE was selectively working in neuronal lineage cells, we examined the luciferase expression level in 293FT cells, non-neuronal cell, transfected with SV::DsRed (Control), SV::Luci, or NSE::Luci plasmid. We confirmed that the luciferase expression level in 293FT transfected with NSE::Luci plasmid was significantly lower than 293FT transfected with SV::Luci plasmid (Fig. 3). This result means that the efficacy of the neuronal cell type-inducible gene expression system depends on neuronal lineage cell type.
To confirm the differentiation potency of NSCs, neural differentiation was induced by RA treatment. Differentiated cells cultured in the presence of 1 µM RA for 7 d were stained for neuronal specific markers, such as TUJ1, MAP2, NCAM, and NF. We confirmed that differentiated cells were positive for all neuronal markers (Fig. 4), meaning that NSCs used in this experiment were efficiently differentiated into neurons.
To confirm whether the luciferase expression pattern of the NSE::Luci-NSCs can be increased after neuronal differentiation (1 µM RA), compared with NSC stage (0 µM RA), we investigated the luciferase expression level at the stage of NSCs and differentiated neurons after transfection with NSE::Luci or SV::Luci plasmids. We confirmed that the luciferase expression level of NSCs transfected with NSE::Luci plasmid was much higher after neuronal differentiation (Fig. 5A). At the neuronal differentiation condition (1 µM RA), the luciferase expression level of NSE::Luci-NSCs was significantly higher than that of SV::Luci-NSCs (Fig. 5B).
These results mean that the transgene expression by NSE promoter was induced by neuronal differentiation. Thus, these findings indicate that the neuronal cell-type-inducible transgene overexpression system appropriates with NSCs, which have a neuronal differentiation potency for combined treatment strategies.
To confirm whether NSE::Luci-NSCs show a consistent high expression pattern in vivo, both SV::Luci-NSCs and NSE::Luci-NSCs were transplanted into the spinal cord, and an IVIS analysis and luciferase assay was carried out 24-48 h after transplantation. Similar to our results in vitro, the luciferase expression level was significantly higher in NSE::Luci-NSCs than in SV::Luci-NSCs (Fig. 6). These results indicate that a combined treatment strategy based on a neuronal cell type-inducible transgene expression system and NSCs can be applied to SCI.
As one of the pathologies that appear after SCI, an ischemic environment in the spinal cord is caused by the destruction of blood vessels; this ischemic condition lasts from several days to several weeks. The survival rate of stem cells transplanted into the ischemic environment would normally rapidly decline. For the protection of transplanted stem cells and the injured spinal cord, we designed a gene and stem cell therapy platform based on NSCs and a neuronal cell type-inducible gene expression system.
Gene overexpression by NSE promoter is already known to work in mature neurons. However, we confirmed that the luciferase expression level of NSE::Luci-NSCs was significantly higher than the luciferase expression level of SV::Luci-NSCs, and the luciferase expression level of 293FT cells (used as a mean non-neuronal cell type) transfected with NSE::Luci plasmid was significantly lower than that of 293FT cells transfected with SV::Luci plasmid. This result means that transgene overexpression by NSE promoter is already working at the NSC stage. A previous study investigated the difference of gene overexpression based on NSE promoter between neurons and non-neuronal cells, such as HEK293 and HeLa cells; however, the study did not examine the difference of gene overexpression at the NSC stage.4 Thus, we carefully suggest that neuron-specific promoter may function extensively in neuronal lineage cells including NSCs.
Additionally, we confirmed that NSE::Luci-NSCs showed a higher luciferase expression level than the SV::Luci-NSCs under normoxia and hypoxia, and the luciferase expression level of NSE::Luci-NSCs was much higher after differentiation into neurons. All of these features can be used advantageously, if the luciferase used in this study were to be replaced by other therapeutic genes.
In our previous study, we confirmed that a NSCs transfected with a hypoxia-inducible gene expression system should be maintained for at least 1 day under hypoxic conditions for transgene overexpression.9 However, the gene expression level of NSCs transfected with a neuronal cell type-inducible gene overexpression system was sufficiently greater in normoxia, namely before transplantation (saving time, as there is no need to wait 1 day). Thus, the high amount of secreted therapeutic protein within 1 day may be useful for improving the survival of transplanted stem cells, as well as protecting the injured spinal cord from exposure to an ischemic environment. Subsequently, the high amount of secreted therapeutic protein from transplanted NSCs would be sustained under the ischemic environment and would also increase further neuronal differentiation.
Thus, these findings suggest that a neuronal cell type-inducible gene expression system is suitable for use with NSCs for expressing a high amount of therapeutic genes, and such gene and NSC therapy based on the present systems described here appear to be very promising as potential treatments for SCI.
In this study, we proposed a combined strategy using a neuronal cell type-inducible transgene overexpression system and NSCs for SCI treatment. We confirmed that the luciferase gene expression level of NSE::Luci-NSCs was significantly higher than that of SV::Luci-NSCs. This pattern was sustained after hypoxic injury and in vivo. Specifically, the luciferase gene expression level observed at the NSC stage was much greater after differentiation into neurons. We suggest that a neuronal cell type-inducible transgene overexpression system is suitable for NSCs; furthermore, a combined treatment strategy based on a neuronal cell type-inducible therapeutic gene overexpression system and NSCs may be a promising tool for the treatment of SCI.
Figures and Tables
Fig. 1
Plasmid construction. Plasmid construction of neuronal cell type-inducible (NSE::Luci) or general (SV::Luci) luciferase overexpression vector. The neuron-specific enolase (NSE) promoter region was inserted to pGL3-basic vector after digestion (NheI and BglII sites).
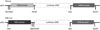
Fig. 2
Luciferase expression pattern of neural stem cells introduced with a neuronal cell type-inducible luciferase overexpression system (NSE::Luci-NSCs) in normoxia and hypoxia. Luciferase expression levels of Control, SV::Luci-NSCs, and NSE::Luci-NSCs examined by luciferase expression imaging at 24-48 h after transfection. (A) The result of luciferase expression imaging indicates that luciferase in NSE::Luci-NSCs group is highly over-expressed, compared to the other groups. The color scale bar indicates the luciferase expression level. (B) Quantitative analysis of luciferase expression imaging in (A). (C) Luciferase expression levels of Control, SV::Luci-NSCs, and NSE::Luci-NSCs examined by luciferase assay at 24-48 h after transfection. Luciferase expression of NSE::Luci-NSC is much higher than the other groups in normoxia and hypoxia. The number of the symbol indicates the value of n. Data are represented as mean±standard error of the mean. *p<0.05. ROI, regions of interest; NSC, neural stem cell; NSE, neuron-specific enolase; RLU, relative light units.
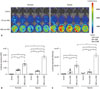
Fig. 3
Neuronal cell-type-inducible luciferase gene overexpression systems in non-neuronal cells. Luciferase expression levels of SV::Luci-293FT and NSE::Luci-293FT confirmed by luciferase assay at 48 h transfection. The number of the symbol indicates the value of n. Data are represented as mean±standard error of the mean. *p<0.05. NSE, neuron-specific enolase; RLU, relative light units.
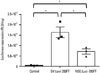
Fig. 4
Neuronal differentiation potency. Neuronal differentiation potency of NSCs was confirmed by fluorescence staining specific for each neuron. (A and B) Most of the differentiated cells are positive for neuron-specific markers, such as beta-III-tubulin, MAP2, and NCAM. (C) The thick axonal bundle was confirmed by staining with neurofilament and beta-III-tubulin. NSC, neural stem cell; MAP2, microtubule-associated protein 2; NCAM, neural cell adhesion molecule.
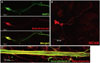
Fig. 5
High luciferase expression pattern after differentiation into neurons. (A) The luciferase expression level in neural stem cells and differentiated neurons was compared by luciferase assay. Luciferase expression is significantly greater in differentiated neurons (with RA) than in neural stem cells (without RA). (B) The luciferase expression level of NSE::Luci-NSCs after neuronal differentiation is significantly greater than that of SV::Luci-NSCs after differentiation. The number of the symbol indicates the value of n. Data are represented as mean±standard error of the mean. *p<0.05. RA, retinoic acid; NSC, neural stem cell; NSE, neuron-specific enolase; RLU, relative light units.
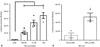
Fig. 6
Luciferase expression pattern of neuronal cell-type-inducible luciferase gene overexpressing neural stem cells after transplantation. (A) Luciferase expression pattern of SV::Luci-NSCs and NSE::Luci-NSCs confirmed by IVIS 24-48 h after cell transplantation. NSE::Luci-NSCs transplanted into the spinal cord show a high luciferase expression, compared to SV::Luci-NSCs, after transplantation. The color scale indicates the luciferase expression level, not the cell numbers in this study. (B) Luciferase expression pattern of SV::Luci-NSCs and NSE::Luci-NSCs confirmed by luciferase assay 24-48 h after cell transplantation. NSE::Luci-NSCs transplanted into the spinal cord consistently show a high luciferase expression pattern, compared to SV::Luci-NSCs, after transplantation. The number of the symbol indicates the value of n. Data are represented as mean±standard error of the mean. *p<0.05. NSE, neuron-specific enolase; NSC, neural stem cell; RLU, relative light units; IVIS, In Vivo Imaging System.
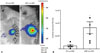
ACKNOWLEDGEMENTS
This study was supported by a grant from the Korea Health Technology R&D Project, Ministry of Health & Welfare, Republic of Korea (A120254); a National Research Foundation of Korea Grant funded by the Korean Government (2012-0001560); and faculty research grants from Yonsei University College of Medicine for 2014 (No. 6-2014-0035).
References
1. Hagg T, Oudega M. Degenerative and spontaneous regenerative processes after spinal cord injury. J Neurotrauma. 2006; 23:264–280.


2. Lee M, Lee ES, Kim YS, Choi BH, Park SR, Park HS, et al. Ischemic injury-specific gene expression in the rat spinal cord injury model using hypoxia-inducible system. Spine (Phila Pa 1976). 2005; 30:2729–2734.


3. Jin H, Liu ML, Kim HA, Lee M, An S, Oh J, et al. Role of the oxygen-dependent degradation domain in a hypoxia-inducible gene expression system in vascular endothelial growth factor gene therapy. Spine (Phila Pa 1976). 2009; 34:E952–E958.


4. Navarro V, Millecamps S, Geoffroy MC, Robert JJ, Valin A, Mallet J, et al. Efficient gene transfer and long-term expression in neurons using a recombinant adenovirus with a neuron-specific promoter. Gene Ther. 1999; 6:1884–1892.


5. Tsuchiya R, Yoshiki F, Kudo Y, Morita M. Cell type-selective expression of green fluorescent protein and the calcium indicating protein, yellow cameleon, in rat cortical primary cultures. Brain Res. 2002; 956:221–229.


6. Kügler S, Kilic E, Bähr M. Human synapsin 1 gene promoter confers highly neuron-specific long-term transgene expression from an adenoviral vector in the adult rat brain depending on the transduced area. Gene Ther. 2003; 10:337–347.


7. Iwai H, Nori S, Nishimura S, Yasuda A, Takano M, Tsuji O, et al. Transplantation of neural stem/progenitor cells at different locations in mice with spinal cord injury. Cell Transplant. 2014; 23:1451–1464.


8. Lu P, Wang Y, Graham L, McHale K, Gao M, Wu D, et al. Long-distance growth and connectivity of neural stem cells after severe spinal cord injury. Cell. 2012; 150:1264–1273.


9. Liu ML, Oh JS, An SS, Pennant WA, Kim HJ, Gwak SJ, et al. Controlled nonviral gene delivery and expression using stable neural stem cell line transfected with a hypoxia-inducible gene expression system. J Gene Med. 2010; 12:990–1001.


10. Kim HM, Hwang DH, Lee JE, Kim SU, Kim BG. Ex vivo VEGF delivery by neural stem cells enhances proliferation of glial progenitors, angiogenesis, and tissue sparing after spinal cord injury. PLoS One. 2009; 4:e4987.


11. Kim HJ, Oh JS, An SS, Pennant WA, Gwak SJ, Kim AN, et al. Hypoxia-specific GM-CSF-overexpressing neural stem cells improve graft survival and functional recovery in spinal cord injury. Gene Ther. 2012; 19:513–521.


12. Oh JS, An SS, Gwak SJ, Pennant WA, Kim KN, Yoon DH, et al. Hypoxia-specific VEGF-expressing neural stem cells in spinal cord injury model. Neuroreport. 2012; 23:174–178.

