Abstract
Purpose
The use of graft tissue fixation using bioabsorbable interference screws (BISs) in anterior cruciate ligament (ACL) reconstruction offers various advantages, but limited pullout strength. Therefore, additional tibial fixation is essential for aggressive rehabilitation. We hypothesized that additional graft tissue fixation using bioabsorbable suture anchors (BSA) would provide sufficient pull-out strength.
Materials and Methods
Twenty four fresh frozen porcine distal femur and patellar tendon preparations were used. All specimens were divided into three groups based on additional fixation methods: A, isolated BIS; B, BIS and BSA; and C, BIS and post cortical screw. Tensile testing was carried out under an axial load. Ultimate failure load and ultimate failure load after cyclic loading were recorded.
Results
The ultimate failure loads after load to failure testing were 166.8 N in group A, 536.4 N in group B, and 438 N in group C; meanwhile, the ultimate failure loads after load to failure testing with cyclic loading were 140 N in group A, 466.5 N in group B, and 400 N in group C. Stiffness after load to failure testing was 16.5 N/mm in group A, 33.5 N/mm in group B, and 40 N/mm in group C. An additional BSA fixation resulted in a significantly higher ultimate failure load and stiffness than isolated BIS fixation, similar to post screw fixation.
The prevalence of anterior cruciate ligament (ACL) injury is increasing due to life style changes and more frequent outdoor activity. Reportedly, ACL reconstruction as an operative treatment offers a success rate of 70-90%.1,2 Early fixation of grafted tendons during early rehabilitation therapy is one of the most important factors of ACL reconstruction. Tibial fixation is also an important factor that can lead to early failure, as it is known to be a weak point during the early stage of biological fixation after ACL reconstruction. Grafts used during ACL reconstruction include bone-patellar tendon-bone and hamstring tendon grafts; however, their use is now less favored due to complications arising from the grafted site. Currently, free soft tissue grafts are more widely accepted in ACL reconstruction. Most hamstring graft tibial fixation techniques utilize interference screws, especially bioabsorbable interference screws (BISs), for increased fixation with less damage to the soft tissues.3 However, when interference screws are used for tibial graft fixation, the graft sometimes loses its tension, so that a hybrid fixation technique (with more than one method of fixation) must be applied. Biomechanical studies showed that interference screws combined with additional indirect distal hamstring tendon fixation techniques can withstand much higher tearing forces than one type of fixation alone.4,5,6,7 However, additional metallic fixation devices (e.g., spike washers, post screws, and metal buttons) cause irritation of the soft tissue around the tibial cortical area, thus causing limitations such as metal removal.8,9 Therefore, we hypothesized that using BISs simultaneously with additional bioabsorbable suture anchor (BSA) devices could augment early fixation. The purpose of this study was to investigate the effectiveness of BSA devices by comparing them to metallic post cortical screws in this biomechanical study.
Cadaveric knee joints are not easily accessible in our country. Instead, we chose to use porcine knee joints in our study, which are anatomically similar and have similar tibial strength. Twenty four fresh frozen porcine distal femur and patellar tendon preparations were used for the experiments. We harvested distal femurs and patellar tendons from 6-month-old experimental pigs, weighing approximately 100 kg. After obtaining the samples, we divided them in half on a sagittal plane using a saw. Each sample, comprising a femur and a patellar tendon, was frozen at -70℃ until the study began, and was thawed for 12 hours at 4℃ and for another 4 hours at room temperature just before the experiments. The patellar tendon samples (5 cm in length and 8 mm in thickness) were harvested from the center of the tendon, and were sutured using a continuously interlocking suture method with a Krackow whip stitch, forming four loops at each end of the tendon (Fig. 1).
A 10-mm tunnel was created and an 8-mm strip of patellar tendon was fixed using a 9×25 mm BIS (BioScrew®; Linvatec Corp., Largo, FL, USA) on the midline of the sagittal plane from the intercondylar notch. According to the hybrid fixation technique, we also used a BSA or a cortical screw as additional fixative methods respectively in the following three groups: group A received BIS fixation only (Fig. 2A); group B was fixed with a 6×16.5 mm Duet™ Suture Anchor (Linvatec Corp., Largo, FL, USA) after BIS fixation (Figs. 2B and 3); and group C was fixed with a 3.5 mm cortical screw after BIS fixation (Fig. 2C). FiberWire® (Arthrex, Naples, FL, USA) was connected to the patellar tendon and each end was ligated. The tendon was then fixed to the clamp of an 858 Table Top System® (MTS Systems Corporation, Eden Prairie, MN, USA) machine with the femoral tunnel parallel to the force of traction for simple and cyclic loading stress tests (Fig. 4). Approximately 10 MPa was applied in order to fix the patellar tendons to the cyclic loading clamp.
An 8-mm patellar tendon strip was inserted into the 10-mm femoral tunnel and fixed with a 9×25 mm BioScrew® without additional fixation.
Four samples from each group were used for load to failure tests using the 858 Table Top System®. Fixed grafted tendons were maintained at a loading speed of 50 mm/min in the direction of the femoral tunnel. The ultimate failure load was measured and recorded for analysis (Fig. 5).
In four samples from each group, a load of 50 N was applied for 30 seconds to prevent any effects of a sudden load. A cyclic loading test was then performed consisting of 100 cycles from 50-250 N with 1 Hz frequency. The direction of force was parallel to the traction force. Load, graft stiffness, failure load, failure mode, and sliding of the graft from the fixation were recorded and analyzed. After the cyclic loading test, a simple loading test was reapplied to the grafted tendon, and the ultimate failure load and stiffness were measured. We analyzed rupture of the graft, graft slippage and breakage, and pull-out of the additional fixation (Fig. 6).
Statistical analysis was performed with SPSS software (Version 11, SPSS Inc., Chicago, IL, USA). Comparison was carried out using Kruskal-Wallis test, and Mann-Whitney U test was performed to detect differences between the three groups. Differences with a significance level of p<0.05 were considered as statistically significant.
The ultimate failure mode of tensile testing before cyclic loading consisted of a pull-out pattern in all three groups. The ultimate failure load of tensile testing before cyclic loading was an average of 166.8±15.2 N (range, 120.5-190.5 N) for group A, 536.4±47.9 N (range, 487.7-700 N) for group B, and 438.0±46.2 N (range, 360.7-561.9 N) for group C [Kruskal-Wallis test p-value (pK)=0.01]. The ultimate failure load of group A was significantly lower than those of groups B and C, while the ultimate failure loads of groups B and C were not significantly different [Mann-Whitney U test p value (pM)=0.02] (Table 1).
The ultimate failure mode of tensile testing after cyclic loading comprised a pull-out pattern in all three groups. The ultimate failure loads during tensile testing after a cyclic loading of 100 cycles with a 50 N preload for 30 sec were on average 140.0±13.6 N (range, 72.1-188.6 N) for group A, 466.5±37.6 N (range, 330.0-520.0 N) for group B, and 400.0±50.8 N (range, 350.0-480.0 N) for group C. The ultimate failure load after cyclic loading of group A was significantly lower than those for groups B and C, and the failure loads for groups B and C were not significantly different (Table 1). There were no graft failures in the ligament portion for all three groups; all failures demonstrated graft slippage in the tunnel without BIS pull-out. There was one case of screw pull-out in group B (additional BSA) and one case of screw pull-out in group C (additional cortical fixation screw). When stiffness was examined, groups B and C had similar results, while group A had significantly lower stiffness (Table 2).
Implant fixation during ACL reconstruction is weakest early after surgery, and fixation of the tibia is relatively weaker than that of the femur.4,10,11,12 Scheffler, et al.13 reported that the main reasons for this include the relatively weaker bone density of the tibia tunnel when compared to the femur and excessive loading on the implants during knee joint flexion. Several reports have demonstrated clinically significant results when using BISs for tibia fixation.14 In contrast, Yoo, et al.7 reported that a BIS with an additional cortical screw and spike washer for fixation of the distal hamstring tendon to the tibia results in a higher load at failure and stiffness when compared to either a single BIS or cortical screw and spike washer fixation alone. However, metallic tibial fixation (with a spike washer or staple) leads to postoperative soft tissue irritation and kneeling pain, which can result in hardware removal.8,9 This study was designed to investigate whether additional soft tissue graft fixation using a BSA generates as much pull-out strength as metallic devices for early rehabilitation, since the use of BSA devices could prevent complications, such as soft tissue irritation.
Suture anchors have proven to be safe and of use in several fields for additive fixation to the articular capsule, ligaments, and tendons, and are an alternative to staples and metallic screws in orthopedic surgery.15 Bioabsorbable screws, which are a more desirable alternative to metallic fixation, are advantageous in that they reduce the complications associated with the use of metal, which may dissolve in the human body. They also exhibit excellent biodynamical strength due to their poly L-lactic acid content, which is derived from polylactic acid. However, these screws were originally associated with problems, such as fixation failure; this was due to their polyglycolic acid content.16,17 Weiler, et al.18 investigated the additional fixation effect of EndoPearl (Livatec, Largo, FL, USA) applied to interference screws in soft tissue implants, such as distal femurs and hamstring tendons. Endopearl has a much greater tensile strength than interference screws. They demonstrated a similar maximum failure power between biodegradable screws and fully-threaded cortical screws on tensile strength testing, which was approximately three times more intense than when additive fixation was not performed. This result was similar to that reported by Yoo, et al.7 In a biodynamical experiment they showed that cortical screws and hybrid fixation added to a washer exhibit a maximum failure power twice that of previous method used. In addition, a simple tensile strength test also demonstrated similar maximum failure power. Their report supported the idea that additive fixation for the tibia in ACL reconstruction is critically important.
Suggs, et al.19 analyzed the graft strength and kinetics of the knee joint after ACL reconstruction using a 3-dimensional computer simulation program. They stated that graft strength has a direct effect on the biokinetics of the knee joint. It is also important to choose both an appropriate graft material and a suitable fixation location for the revitalization of normal knee biokinetics. In the current study, groups B and C, which received additional fixation, showed a higher strength score than group A. These results indicated that additional fixation of the tibial graft helps in preventing failure of reconstruction. Taking the results of Suggs, et al.19 into consideration, the current study also supported that additional fixation may be important in recovery of the knee joint to its normal state.
By analyzing the failure patterns resulting from maximum damage strength, we showed that true rupture of the grafts and pull-out of interference screws is not prevalent, while slippage of the grafts was seen in the femoral tunnel. Partial pull-out occurred in approximately 50% of each case in the groups with additional fixation. From these results, we discerned that single fixation of interference screws alone is not strong enough for ACL reconstruction, and additional fixation is recommended for early accelerated rehabilitation therapy.
In studies with porcine bones, Nurmi, et al.20 reported that the trabecular bone density of the porcine tibia was significantly higher than that of the human tibia, as assessed by peripheral quantitative computed tomography scanning. The authors concluded that porcine tibias do not provide a reasonable surrogate for human cadaveric tibias, when evaluating ACL reconstruction. The bone mineral density of human proximal tibias in younger active populations has been reported to range from 1.09-1.30 g/cm2.5,21,22,23 Sim, et al.24 reported that the bone density of the porcine femur is on average 1.498±0.141 g/cm2 (1.381-1.750 g/cm2), and it was considered that the porcine femur provided sufficient bony support.
Limitations of this study are that first, we performed this study with porcine bone and ligaments due to the limited availability of human cadavers. Even if the porcine femur has sufficient bone density comparable to that of a human, we believe that human cadavers are better substitutes for this type of study. Second, the biomechanical experiments of this study cannot reflect overall normal kinetics of human knee. The tensile force was applied to the grafts in the same single direction as the femoral tunnel. This direction of force does not correctly reflect the kinetics of the human ACL, which provides resistance against anterior-posterior and rotational forces. Thirdly, the effect of bioabsorbable suture anchor is affected by the relationship between the bony shape and the tunnel location. For this reason, we maintained the same settings and other conditions, such as bone preparation, tunnel location, and tunnel inclination, except the fixation method. We considered that only a difference in fixation methods was an independent factor of this experimental study. Although this study has biomechanical flaws, we believe that it is meaningful comparison of fixation strength between bioabsorbable interference screw and additional fixation, such as metallic screw and bioabsorbable suture anchors, under controlled conditions.
In conclusion, additional fixation using a BSA or a post cortical screw provided sufficient pull-out strength for the tibial side during ACL reconstruction. In simple and cyclic loading tests, the BSA technique for additional fixation showed similar fixation strength to the cortical screw technique. Both techniques had superior fixation strength when compared to the group with no additional fixation. In ACL reconstruction, additional fixation using BSA devices for fixation of grafted tendons to the tibial side provides good fixation strength similar to that of metallic cortical screws in early accelerated rehabilitation.
Figures and Tables
Fig. 2
Additional fixation devices used for this study. (A) 9×25 mm BioScrew®. (B) 6×16.5 mm Duet™. (C) 3.5 mm cortical screw.
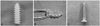
Fig. 3
(A) Bioabsorbable suture anchor (Duet™). (B) Separated suture anchor tip and FiberWire® (Arthrex) connecting the tendon. (C) Reassembled bioabsorbable suture anchor.
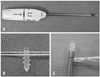
Fig. 4
The 858 Table Top System® testing apparatus is shown with a porcine femur and graft. The free end of the patellar tendon graft was fixed to the inferior clamp of the machine with FiberWire®.
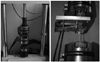
Fig. 5
Load elongation curve of the load to failure test (Elongation=EU-E0). UFL, ultimate failure load.
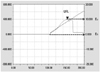
References
1. Dragoo JL, Braun HJ, Durham JL, Chen MR, Harris AH. Incidence and risk factors for injuries to the anterior cruciate ligament in National Collegiate Athletic Association football: data from the 2004-2005 through 2008-2009 National Collegiate Athletic Association Injury Surveillance System. Am J Sports Med. 2012; 40:990–995.


2. Almqvist KF, Willaert P, De Brabandere S, Criel K, Verdonk R. A long-term study of anterior cruciate ligament allograft reconstruction. Knee Surg Sports Traumatol Arthrosc. 2009; 17:818–822.


3. Brand JC Jr, Nyland J, Caborn DN, Johnson DL. Soft-tissue interference fixation: bioabsorbable screw versus metal screw. Arthroscopy. 2005; 21:911–916.


4. Giurea M, Zorilla P, Amis AA, Aichroth P. Comparative pull-out and cyclic-loading strength tests of anchorage of hamstring tendon grafts in anterior cruciate ligament reconstruction. Am J Sports Med. 1999; 27:621–625.


5. Magen HE, Howell SM, Hull ML. Structural properties of six tibial fixation methods for anterior cruciate ligament soft tissue grafts. Am J Sports Med. 1999; 27:35–43.


6. Tetsumura S, Fujita A, Nakajima M, Abe M. Biomechanical comparison of different fixation methods on the tibial side in anterior cruciate ligament reconstruction: a biomechanical study in porcine tibial bone. J Orthop Sci. 2006; 11:278–282.


7. Yoo JC, Ahn JH, Kim JH, Kim BK, Choi KW, Bae TS, et al. Biomechanical testing of hybrid hamstring graft tibial fixation in anterior cruciate ligament reconstruction. Knee. 2006; 13:455–459.


8. Hill PF, Russell VJ, Salmon LJ, Pinczewski LA. The influence of supplementary tibial fixation on laxity measurements after anterior cruciate ligament reconstruction with hamstring tendons in female patients. Am J Sports Med. 2005; 33:94–101.


9. Jansson KA, Linko E, Sandelin J, Harilainen A. A prospective randomized study of patellar versus hamstring tendon autografts for anterior cruciate ligament reconstruction. Am J Sports Med. 2003; 31:12–18.


10. Beynnon BD, Johnson RJ, Abate JA, Fleming BC, Nichols CE. Treatment of anterior cruciate ligament injuries, part 2. Am J Sports Med. 2005; 33:1751–1767.


11. Clark R, Olsen RE, Larson BJ, Goble EM, Farrer RP. Cross-pin femoral fixation: a new technique for hamstring anterior cruciate ligament reconstruction of the knee. Arthroscopy. 1998; 14:258–267.


12. Howell SM, Deutsch ML. Comparison of endoscopic and two-incision techniques for reconstructing a torn anterior cruciate ligament using hamstring tendons. Arthroscopy. 1999; 15:594–606.


13. Scheffler SU, Südkamp NP, Göckenjan A, Hoffmann RF, Weiler A. Biomechanical comparison of hamstring and patellar tendon graft anterior cruciate ligament reconstruction techniques: the impact of fixation level and fixation method under cyclic loading. Arthroscopy. 2002; 18:304–315.


14. Ma CB, Francis K, Towers J, Irrgang J, Fu FH, Harner CH. Hamstring anterior cruciate ligament reconstruction: a comparison of bioabsorbable interference screw and endobutton-post fixation. Arthroscopy. 2004; 20:122–128.


15. Bushnell BD, Byram IR, Weinhold PS, Creighton RA. The use of suture anchors in repair of the ruptured patellar tendon: a biomechanical study. Am J Sports Med. 2006; 34:1492–1499.


16. Benedetto KP, Fellinger M, Lim TE, Passler JM, Schoen JL, Willems WJ. A new bioabsorbable interference screw: preliminary results of a prospective, multicenter, randomized clinical trial. Arthroscopy. 2000; 16:41–48.


17. Sassmannshausen G, Carr CF. Transcutaneous migration of a tibial bioabsorbable interference screw after anterior cruciate ligament reconstruction. Arthroscopy. 2003; 19:E133–E136.


18. Weiler A, Richter M, Schmidmaier G, Kandziora F, Südkamp NP. The EndoPearl device increases fixation strength and eliminates construct slippage of hamstring tendon grafts with interference screw fixation. Arthroscopy. 2001; 17:353–359.


19. Suggs J, Wang C, Li G. The effect of graft stiffness on knee joint biomechanics after ACL reconstruction--a 3D computational simulation. Clin Biomech (Bristol, Avon). 2003; 18:35–43.


20. Nurmi JT, Sievänen H, Kannus P, Järvinen M, Järvinen TL. Porcine tibia is a poor substitute for human cadaver tibia for evaluating interference screw fixation. Am J Sports Med. 2004; 32:765–771.


21. Asahina S, Muneta T, Ishibashi T, Yamamoto H. Effects of knee flexion angle at graft fixation on the outcome of anterior cruciate ligament reconstruction. Arthroscopy. 1996; 12:70–75.


22. Leppälä J, Kannus P, Natri A, Pasanen M, Sievänen H, Vuori I, et al. Effect of anterior cruciate ligament injury of the knee on bone mineral density of the spine and affected lower extremity: a prospective one-year follow-Up study. Calcif Tissue Int. 1999; 64:357–363.

