Abstract
Purpose
The aim of this study was to assess the feasibility and efficacy of hypofractionated simultaneous integrated boost-intensity modulated radiotherapy (SIB-IMRT) using three-layered planning target volumes (PTV) for malignant gliomas.
Materials and Methods
We conducted a retrospective analysis of 12 patients (WHO grade IV-10; III-2) postoperatively treated with SIB-IMRT with concurrent temozolomide. Three-layered PTVs were contoured based on gadolinium-enhanced magnetic resonance imaging as follows; high risk PTV (H-PTV) as the area of surgical bed including residual gross tumor with a 0.5 cm margin; low risk PTV (L-PTV) as the area surrounding the high risk PTV with 1.5 cm margin; moderate risk PTV (M-PTV) as a line at one-third the distance from high risk PTV to low risk PTV. Total dose to high risk PTV was 70 Gy in 8 and 62.5 Gy in 4 patients.
Results
The median follow-up time was 52 months in surviving patients. The 2- and 5-year overall survival (OS) rates were 66.6% and 47.6%, respectively. The 2- and 5-year progression-free survival (PFS) rates were 57.1% and 45.7%, respectively. The median OS and PFS were 48 and 31 months, respectively. Six patients (50%) progressed: in-field only in one, out-field or disseminated in 4, and both in one patient. All patients completed planned treatments without a toxicity-related gap. Asymptomatic radiation necrosis was observed in 4 patients at post-radiotherapy 9-31 months.
Currently, the standard radiotherapy for malignant glioma is to deliver a total of 60 Gy to the surgical bed or residual tumor with conventional fractionation using 3-dimensional conformal radiotherapy. However, the majority of tumors recur or progress at the primary lesions.1 Although a number of trials have attempted to evaluate the benefit of dose escalation, most of them have failed to prove a benefit for conventional radiotherapy with more than 60 Gy.2-4 Simultaneous integrated boost (SIB)-intensity modulated radiotherapy (IMRT) has emerged as a novel treatment method and has been shown to provide dosimetric benefits for protecting adjacent normal tissues.5,6 Using this method, overall treatment time can be shortened and a biologically effective dose (BED) can be escalated by hypofractionation, both of which are important for overcoming radioresistance.7
At our institution, we have begun to offer hypofractionated SIB-IMRT as an option to patients with malignant glioma since 2004. Since malignant gliomas typically show a gradient of infiltrating tumor cells that decreases as one moves away from the mass on microscopy,8,9 we used three-layered planning target volumes (PTV) to deliver a sufficient dose to the peritumoral area. We conducted this study retrospectively to assess the feasibility and efficacy of hypofractionated SIB-IMRT using three-layered PTV for malignant gliomas.
This study was approved by the Institutional Review Board of our institution (approval number: 4-2012-0938).
This study included 12 patients with histologically confirmed malignant gliomas (10 glioblastomas, 2 anaplastic astrocytomas) who were treated by SIB-IMRT postoperatively between January 2004 and December 2007. All patients provided informed consent prior to undergoing radiotherapy. The median follow-up periods for all the patients and the surviving patients were 36 months (range, 8-65 months) and 52 months (range, 21-65 months), respectively. Table 1 summarizes their clinical characteristics. The median age of the patients was 40.5 years (range, 21-70 years). The numbers of patients who underwent gross total resection, subtotal resection and partial resection were nine, two and one, respectively. Retrospective O6-methylguanine-DNA methyltrasferase (MGMT) promoter methylation analysis was available in 10 patients: 8 patients were unmethylated and 2 patients were methylated. In all patients, postoperative brain magnetic resonance imaging (MRI) was performed within 48 h after surgery to distinguish residual tumor from postoperative change. Radiotherapy was begun within 2-3 weeks after surgery in principle.
Target volume definitions and order of delineation were as follows. First, H-PTV was set by drawing a 0.5 cm margin to the postoperative surgical margin and the residual gross tumor, and then L-PTV was set by expanding the H-PTV 3-dimensionally to provide a 1.5 cm margin. Lastly, M-PTV was drawn at the point close to a third of the way to H-PTV along the shortest distance between H-PTV and L-PTV. Organs at risk, such as the eyes, lenses, optic chiasm, optic nerves, brain stem, and scalp were also delineated.
In all patients, daily doses of 2.5, 2.15 and 1.8 Gy were prescribed to H-PTV, M-PTV and L-PTV, respectively. In our institution, two radiation oncologists treated brain tumors, and the dose schemes administered thereby were different between the two. The first scheme was administered in 8 patients; 28 fractions of irradiation were administered at the total doses of 70 Gy, 62 Gy and 50.4 Gy, respectively. The other schedule was performed in 4 patients; irradiation was performed 25 times with the total doses of 62.5 Gy, 53.8 Gy and 45 Gy, respectively. When the total prescribed doses were converted to a BED with α/β ratio=3, 8 patients (67%) received ≥125 BEDGy3 and the remaining 4 (33%) patients received 114.6 BEDGy3. Post-operative radiotherapy was initiated 14-39 days (median 16 days) after surgery. The start of radiotherapy was delayed in 2 patients, who were operated on at another hospital and referred to our hospital for radiotherapy. Median overall treatment time was 40.5 days (range, 37-51 days). The treatment period exceeded 6 weeks in 4 patients because of failure with medical equipment in 2 patients, leukocytopenia in one patient, and a poor general condition in one patient. Two patients underwent linac-based IMRT, and 9 underwent helical tomotherapy. Linac-based IMRT was planned with the Corvus® (Nomos Corp., Pittsburg, PA, USA) system, and helical tomotherapy with the Hi-Art® (Tomotherapy Inc., Madison, WI, USA) planning station.
We performed a comparative planning study in order to determine the dosimetric benefits of a three-layered target volume plan (3LP), compared to a simple two-layered target volume plan (2LP) in terms of accounting for gradual decreases of invasion into surrounding normal brain tissue by glioma cells. 3LP was eventually applied to the patients. In 2LP, M-PTV was removed from prescriptions while maintaining the same prescription and dose constraints for other PTVs and normal tissues for rational comparison. We assumed that all of the 12 patients were prescribed 70 Gy, 60.2 Gy and 50.4 Gy with 28 fractions for H, M and L-PTV, respectively, although 4 of the 12 patients actually received 25 fractions. The results of the two plans were compared via a radiation dose profile, differential dose volume histogram (DVH), and 3D-dose distribution using Mathmatica® (Wolfram Research, Champaign, IL, USA).
All of the patients received temozolomide (TMZ)-based chemotherapy, which was administered concurrently with radiotherapy in 11 of the 12 patients and sequentially after radiotherapy in 1 patient. The drug was administered at 75 mg/m2 daily during radiotherapy, and 150-200 mg/m2 daily after radiotherapy. The cyclic administration of TMZ was performed for 4-24 cycles (median 11.5 cycles).
Toxicity was graded according to the toxicity criteria of the Radiation Therapy Oncology Group/European Organization for Research and Treatment of Cancer. In all patients, complete blood cell counts were taken weekly during radiotherapy. MRI was obtained 4 weeks after the completion of radiotherapy and every 3 to 4 months thereafter to check for progression. In-field progression was defined as recurrence within the target volumes. Out-field recurrence was defined as recurrence outside of the L-PTV. Dissemination was defined as recurrence of multiple foci outside the target volume, including leptomeningeal seeding. In cases of patients who underwent total removal of a tumor, recurrence was regarded as progression. Pseudoprogression was defined as a transient increase in contrast enhancement within the radiation field, detected before 3 months post-radiotherapy and resolving or stabilizing after 1-2 months.
Progression-free survival (PFS) and overall survival (OS) were calculated from the day of surgery and estimated by the Kaplan-Meier method. The significance of prognostic factors was analyzed by the log-rank test.
Hematologic toxicities during the treatment period included the following: grade I anemia in 2, grade II anemia in one, grade II thrombocytopenia in 2, and grade III neutropenia in 2 patients. Grade I central nervous system toxicity was observed in 2 patients and grade II in 3 patients. Four patients experienced decreased appetite. Three patients showed pseudoprogression. Radiation necrosis (RN) occurred in 4 patients at post-radiotherapy 9, 11, 14 and 31 months without any symptom. Fig. 1 demonstrates the serial MRI and helical tomotherapy plan of a patient with RN detected at post-radiotherapy 11 months. Intracranial hemorrhage developed in one patient at post-radiotherapy 4 years with sudden signs of increased intracranial pressure (IICP), while the correlation between this and radiotherapy was unclear. The patient recovered after surgical removal of the hematoma.
The median follow-up periods for all of the patients and the surviving patients were 28 months (range 10-65 months) and 52 months (range 21-65), respectively. The 2-, 3- and 5-year OS rates were 66.6%, 57.1% and 47.6%, respectively. The 2-, 3- and 5-year PFS rates were 57.1%, 45.7% and 45.7%, respectively (Fig. 2). The median OS was 48 months (95% CI: 29-67 months). Six patients experienced progression, and the median PFS was 32 months. Five of the six patients with progression died of the disease. The other patient, who is still alive, experienced tumor recurrence as leptomeningeal seeding at post-radiotherapy 21 months and received craniospinal irradiation and TMZ-based salvage treatment. Five of the six patients without progression have remained in a disease-free state up to the last follow-up, while the other patient died of acute pneumonia.
Significant prognostic factors shown to be related to better OS on univariate analysis included Karnofsky performance status (KPS) 90 or 100 (p=0.005) and a total dose ≥125 BEDGy3 (p=0.032). All patients with MGMT methylation were alive, but the difference in OS was not significant (p=0.167). Patients without pseudoprogression (p=0.237) and with radiation necrosis (p=0.220) tended to show better OS. For PFS, KPS 90 or 100 (p=0.037) and without pseudoprogression (p=0.025) were shown to be significant factors on univariate analysis. Patients who received a total dose ≥125 BEDGy3 (p=0.094) and with radiation necrosis (p=0.189) tended to exhibit better PFS, but these were not statistically significant (Table 2).
In total, six cases of progression were noted. One was in-field (in H-PTV), one was in-field (in L-PTV) with dissemination, one was out-field, one was out-field with dissemination, and two involved dissemination.
Of the 8 patients prescribed at 70 Gy (BED 128 Gy3), there were no in-field failures, but out-field failures were observed in 3 patients. The failure patterns were as follows: skin recurrence caused by histologically confirmed surgical seeding; multifocal recurrence occurred out-field of the ipsilateral and contra-lateral brain with leptomeningeal seeding; and out-field recurrence followed by disseminated disease. On the contrary, for the 4 patients prescribed at 62.5 Gy (BED 114 Gy3), recurrence occurred in 3 patients. One patient among them experienced in-field failure at the L-PTV 12 months after radiotherapy with concomitant leptomeningeal seeding. The extent of disease was very wide and tumor invaded the optic chiasm. In another patient, leptomeningeal seeding occurred in the lateral ventricle outside the radiotherapy (RT) field and in the parietal area at 21 months after treatment of a tumor of left temporal origin. The other patient died at 15 months of in-field failure that occurred in H-PTV at post-RT 8 months.
Fig. 3 shows the 3D dose distribution of the target volumes and dose profiles on the axial plane for one representative patient. The decrease in dose from the margin of H-PTV to L-PTV was much slower in 3LP than in 2LP, in which the dose decreased sharply as soon as it passed out of H-TPV. Between the two planning methods, differential DVHs were similar for H-PTV, but clearly different for M-PTV and L-PTV. While L-PTV in 2LP had a sharp peak at 50.4 Gy, that in 3LP showed a gentle slope, suggesting a gradual decrease of radiation dose from the border of H-PTV to L-PTV. In 3LP and 2LP for the 15 patients, the D95% of H-PTV were 69.7±0.5 Gy and 69.6±0.6 Gy, respectively, and those of L-PTV were 52.1±1.1 Gy and 50.0±0.8 Gy, respectively, showing little difference between the two plans; however, those of M-PTV were 60.3±1.9 Gy and 54.9±1.7 Gy, respectively, showing a dose increase of around 6 Gy (10%) in 3LP. The D95% of M-PTV in 3LP was close to the dose prescribed for M-PTV of the three-layered target. V60 Gy and V50 Gy were about 22% and 37% higher, respectively, in 3LP than in 2LP. However, the difference in dose distribution for surrounding normal tissues between the two plans was less than 3%, a negligible level.
Malignant glioma is known to involve a dismal prognosis despite the availability of multi-modality approaches of surgery, radiotherapy and TMZ-based chemotherapy.10 This study reports promising preliminary treatment outcomes and relatively high incidence of RN after SIB-IMRT using three-layered target volumes.
In this study, we applied an intermediate hypofractionation scheme (2.5 Gy per fraction to high-risk area) instead of the conventional fractionation. Since glioblastoma cells exhibit a low ratio on in vitro survival curves11 and all radiation dose escalations up to 90 Gy with conventional fractionation develop in-field recurrence,12 we hypothesized that optimal fractionation should go beyond the fraction size of 1.8-2.0 Gy. In a previous study by the Narayana group,13 who used IMRT of conventional fractionation, 95% of relapses were still local. In our study, there was no in-field failure among patients whose H-PTV was irradiated at 70 Gy (BED 128 Gy3) with 2.5 Gy per fraction. We used 2.5 Gy rather than a larger fraction size in order to reduce the risk of complications and to maximize the benefits of fractionation through a treatment period of around 5 weeks. In the cases of the IMRT trial by Floyd, et al.14 that prescribed a total dose of 50 Gy (133 BEDGy3) with 5 Gy per fraction and the trial by Iuchi, et al.15 that prescribed a total dose of 48-68 Gy (260 BEDGy3) with 6-8.5 Gy per fraction, the incidences of RN requiring surgical intervention were 15% (3 of 20 patients) and 12% (3 of 25 patients), respectively. In our study, 4 of 12 patients (33%) experienced RN. They were all asymptomatic and the necrosis occurred mostly near the H-PTV area. One possible reason for this relatively high incidence of RN is the concurrent use of TMZ. Since both the first and the 5-year follow-up report of concurrent TMZ and conventional RT16,17 and a single institutional experience of hypofractionated RT with concurrent TMZ did not mention RN specifically,18 little is known about the incidence of RN after concurrent TMZ and hypofractionated RT. Despite a small patient number of our study, we think that this could be useful information.
We used α/β ratio of 3 Gy because malignant gliomas are considered as a late responding tissue like neural tissue due to its relative radioresistance,14 and there are experimental and clinical data which have reported α/β ratio of 3 Gy for malignant glioma cells.19,20 Furthermore, dose to the perilesional tissue has to be concerned to estimate the risk of RN for surrounding normal brain tissue which is included in the target volume.21
All patients in this study underwent resection and 93% received total or subtotal resection. This might have contributed to the improved survival in our study. Also, it could a possible explanation for no symptom of our patients with RN. It is possible that the focal IICP from a small degree of radiation necrosis can be dispersed through surgical defects caused by preceding surgery, and therefore, does not cause clinical symptoms.
Some authors have reported that a delayed start of radiotherapy lowers survival rates.22-24 In our patients, the median length of time between surgery and postoperative radiotherapy was 16 days. Except 2 patients who had surgery at another hospital and who were referred to our hospital for radiotherapy, and one patient with anaplastic glioma, all of the remaining 9 patients began postoperative radiotherapy within 2-3 weeks. Suzuki, et al.25 reported dismal prognoses despite the use of same fractionation scheme reported in this study. Their study, however, differed from this study in two ways: a two-layered target volume was used and the median time from operation to RT was 23 days, a week longer than ours.
In this study, we used three-layered target volumes. In comparison with 2LP, 3LP could achieve more differentiated fractional doses accounting for the gradual decrease in invasion of surrounding normal brain tissue malignant glioma cells from the gross tumor or surgical resection margin. As proven by clonogenic assay, which is a key experiment in radiation biology, cell colonies survive in proportion to the number of plated cells if different numbers of cells are plated in cell culture dishes and are irradiated.26 This supports the fact that different radiation doses should be prescribed according to the number or density of cells.
An another interesting finding of this study is a relative increase in multifocal or disseminated recurrence in spite of the increased local control through SIB-IMRT. In malignant glioma, multifocality has been reported to be around 10-20%; however, our study showed that it may increase up to 50% in long-term follow-up, even with temozolomide-based chemotherapy. This suggests that if local control and survival are improved, a risk of dissemination may increase.
Since this report is a single institutional, retrospective study of small number of patients, the result of our study is hard to be generalized to all malignant glioma patients. In addition, our study is a preliminary report of single treatment technique and not a comparison study, therefore, it is difficult to draw a conclusion involving superiority of the technique. Despite such limitations, the encouraging outcomes in this report warrant further investigation and future clinical studies of IMRT for malignant gliomas.
In summary, hypofractionated SIB-IMRT for malignant glioma seems to be tolerable and improving local control in patients who underwent resection. Since the incidence of RN is relatively high in these patients with concurrent TMZ, this technique might be safer and helpful for the patients with contraindications for TMZ.
Figures and Tables
Fig. 1
A patient who experienced non-symptomatic radiation necrosis 11 months after treatment. He underwent near total tumor removal and helical tomotherapy based on the three-layered target volumes with dose prescriptions of 70 Gy, 60.2 Gy, and 50 Gy. He is alive after more than 5 years without evidence of disease progression. RT, radiotherapy.

Fig. 3
Comparative planning study between three-(3LP) and two-layered plans (2LP). (A and B) Three-dimensional dose distributions in 3LP and 2LP. (C) A coronal two-dimensional dose distribution along the red line in three-dimensional dose distribution. Points a and b mark the outer margins of H-PTV and L-PTV, respectively. The elliptical purple area from points a to b shows the area of key dose differences between 3LP and 2LP. (D) Differential dose volume histogram for H, M, L-PTVs in two plans. PTV, planning target volumes.
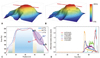
References
1. Lee SW, Fraass BA, Marsh LH, Herbort K, Gebarski SS, Martel MK, et al. Patterns of failure following high-dose 3-D conformal radiotherapy for high-grade astrocytomas: a quantitative dosimetric study. Int J Radiat Oncol Biol Phys. 1999; 43:79–88.


2. Selker RG, Shapiro WR, Burger P, Blackwood MS, Arena VC, Gilder JC, et al. The Brain Tumor Cooperative Group NIH Trial 87-01: a randomized comparison of surgery, external radiotherapy, and carmustine versus surgery, interstitial radiotherapy boost, external radiation therapy, and carmustine. Neurosurgery. 2002; 51:343–355.


3. Prados MD, Wara WM, Sneed PK, McDermott M, Chang SM, Rabbitt J, et al. Phase III trial of accelerated hyperfractionation with or without difluromethylornithine (DFMO) versus standard fractionated radiotherapy with or without DFMO for newly diagnosed patients with glioblastoma multiforme. Int J Radiat Oncol Biol Phys. 2001; 49:71–77.


4. Souhami L, Seiferheld W, Brachman D, Podgorsak EB, Werner-Wasik M, Lustig R, et al. Randomized comparison of stereotactic radiosurgery followed by conventional radiotherapy with carmustine to conventional radiotherapy with carmustine for patients with glioblastoma multiforme: report of Radiation Therapy Oncology Group 93-05 protocol. Int J Radiat Oncol Biol Phys. 2004; 60:853–860.


5. MacDonald SM, Ahmad S, Kachris S, Vogds BJ, DeRouen M, Gittleman AE, et al. Intensity modulated radiation therapy versus three-dimensional conformal radiation therapy for the treatment of high grade glioma: a dosimetric comparison. J Appl Clin Med Phys. 2007; 8:47–60.


6. Chan MF, Schupak K, Burman C, Chui CS, Ling CC. Comparison of intensity-modulated radiotherapy with three-dimensional conformal radiation therapy planning for glioblastoma multiforme. Med Dosim. 2003; 28:261–265.


7. Hall EJ, Giaccia AJ. Radiobiology for the radiologist. 7th ed. Philadelphia: Wolters Kluwer Health/Lippincott Williams & Wilkins;2012.
8. Apuzzo MLJ. AANS Publications Committee. Malignant cerebral glioma. Park Ridge, Ill: American Association of Neurological Surgeons;1990.
9. Phillips TL, Hoppe R, Roach M, Leibel SA. Leibel and Phillips textbook of radiation oncology. 3rd ed. Philadelphia: Elsevier/Saunders;2010.
10. Stupp R, Dietrich PY, Ostermann Kraljevic S, Pica A, Maillard I, Maeder P, et al. Promising survival for patients with newly diagnosed glioblastoma multiforme treated with concomitant radiation plus temozolomide followed by adjuvant temozolomide. J Clin Oncol. 2002; 20:1375–1382.


11. Haas-Kogan DA, Yount G, Haas M, Levi D, Kogan SS, Hu L, et al. p53-dependent G1 arrest and p53-independent apoptosis influence the radiobiologic response of glioblastoma. Int J Radiat Oncol Biol Phys. 1996; 36:95–103.


12. Chan JL, Lee SW, Fraass BA, Normolle DP, Greenberg HS, Junck LR, et al. Survival and failure patterns of high-grade gliomas after three-dimensional conformal radiotherapy. J Clin Oncol. 2002; 20:1635–1642.


13. Narayana A, Yamada J, Berry S, Shah P, Hunt M, Gutin PH, et al. Intensity-modulated radiotherapy in high-grade gliomas: clinical and dosimetric results. Int J Radiat Oncol Biol Phys. 2006; 64:892–897.


14. Floyd NS, Woo SY, Teh BS, Prado C, Mai WY, Trask T, et al. Hypofractionated intensity-modulated radiotherapy for primary glioblastoma multiforme. Int J Radiat Oncol Biol Phys. 2004; 58:721–726.


15. Iuchi T, Hatano K, Narita Y, Kodama T, Yamaki T, Osato K. Hypofractionated high-dose irradiation for the treatment of malignant astrocytomas using simultaneous integrated boost technique by IMRT. Int J Radiat Oncol Biol Phys. 2006; 64:1317–1324.


16. Stupp R, Mason WP, van den, Weller M, Fisher B, Taphoorn MJ, et al. Radiotherapy plus concomitant and adjuvant temozolomide for glioblastoma. N Engl J Med. 2005; 352:987–996.


17. Stupp R, Hegi ME, Mason WP, van den Bent MJ, Taphoorn MJ, Janzer RC, et al. Effects of radiotherapy with concomitant and adjuvant temozolomide versus radiotherapy alone on survival in glioblastoma in a randomised phase III study: 5-year analysis of the EORTC-NCIC trial. Lancet Oncol. 2009; 10:459–466.


18. Cao JQ, Fisher BJ, Bauman GS, Megyesi JF, Watling CJ, Macdonald DR. Hypofractionated radiotherapy with or without concurrent temozolomide in elderly patients with glioblastoma multiforme: a review of ten-year single institutional experience. J Neurooncol. 2012; 107:395–405.


19. Yang X, Darling JL, McMillan TJ, Peacock JH, Steel GG. Radiosensitivity, recovery and dose-rate effect in three human glioma cell lines. Radiother Oncol. 1990; 19:49–56.


20. Shibamoto Y, Nishimura Y, Tsutsui K, Sasai K, Takahashi M, Abe M. Comparison of accelerated hyperfractionated radiotherapy and conventional radiotherapy for supratentorial malignant glioma. Jpn J Clin Oncol. 1997; 27:31–36.


21. Romanelli P, Conti A, Pontoriero A, Ricciardi GK, Tomasello F, De Renzis C, et al. Role of stereotactic radiosurgery and fractionated stereotactic radiotherapy for the treatment of recurrent glioblastoma multiforme. Neurosurg Focus. 2009; 27:E8.


22. Irwin C, Hunn M, Purdie G, Hamilton D. Delay in radiotherapy shortens survival in patients with high grade glioma. J Neurooncol. 2007; 85:339–343.


23. Burnet NG, Jena R, Jefferies SJ, Stenning SP, Kirkby NF. Mathematical modelling of survival of glioblastoma patients suggests a role for radiotherapy dose escalation and predicts poorer outcome after delay to start treatment. Clin Oncol (R Coll Radiol). 2006; 18:93–103.


24. Kim YS, Kim SH, Cho J, Kim JW, Chang JH, Kim DS, et al. MGMT gene promoter methylation as a potent prognostic factor in glioblastoma treated with temozolomide-based chemoradiotherapy: a single-institution study. Int J Radiat Oncol Biol Phys. 2012; 84:661–667.

