Abstract
Purpose
Functional mitral regurgitation (FMR) and myocardial dyssynchrony commonly occur in patients with dilated cardiomyopathy (DCM). The aim of this study was to elucidate changes in FMR in relation to those in left ventricular (LV) dyssynchrony as well as geometric parameters of the mitral valve (MV) in DCM patients during dobutamine infusion.
Materials and Methods
Twenty-nine DCM patients (M:F=15:14; age: 62±15 yrs) with FMR underwent echocardiography at baseline and during peak dose (30 or 40 ug/min) of dobutamine infusion. Using 2D echocardiography, LV end-diastolic volume, end-systolic volume (LVESV), ejection fraction (EF), and effective regurgitant orifice area (ERO) were estimated. Dyssynchrony indices (DIs), defined as the standard deviation of time interval-to-peak myocardial systolic contraction of eight LV segments, were measured. Using the multi-planar reconstructive mode from commercially available 3D image analysis software, MV tenting area (MVTa) was measured. All geometrical measurements were corrected (c) by the height of each patient.
Results
During dobutamine infusion, EF (28±8% vs. 39±11%, p=0.001) improved along with significant decrease in cLVESV (80.1±35.2 mm3/m vs. 60.4±31.1 mm3/m, p=0.001); cMVTa (1.28±0.48 cm2/m vs. 0.79±0.33 cm2/m, p=0.001) was significantly reduced; and DI (1.31±0.51 vs. 1.58±0.68, p=0.025) showed significant increase. Despite significant deterioration of LV dyssynchrony during dobutamine infusion, ERO (0.16±0.09 cm2 vs. 0.09±0.08 cm2, p=0.001) significantly improved. On multivariate analysis, ΔcMVTa and ΔEF were found to be the strongest independent determinants of ΔERO (R2=0.443, p=0.001).
Functional mitral regurgitation (FMR) is common in patients with heart failure and is a marker for adverse clinical outcomes.1,2,3 Increased mitral valve tenting area (MVTa) has been identified as a determinant of the severity of FMR in patients with dilated cardiomyopathy (DCM).4,5,6,7 Recent data have revealed the potential role of intra-ventricular dyssynchrony in relation to dynamic changes in mitral regurgitation (MR) in failing hearts.8,9 Although previous studies have evaluated dynamic changes in FMR, the precise mechanism involved therein remains uncertain.
The purpose of this study was to elucidate the changes in FMR in relation to those in left ventricular (LV) dyssynchrony and mitral valve (MV) geometry as well as LV systolic function in DCM patients during dobutamine infusion.
Twenty-nine consecutive DCM patients with an LV ejection fraction (EF) of less than 40% and significant MR (≥grade I) were included in this study. All patients underwent coronary angiography to exclude those with any significant coronary artery disease. Exclusion criteria were 1) structural abnormalities of the mitral apparatus, such as chordae rupture, prolapse, or restricted leaflet due to degenerative calcification 2) atrial fibrillation. 3) Inadequate echocardiographic window of the LV structure and the mitral apparatus to not allow analysis of 3D geometry, and 4) inadequate Doppler color flow image for proximal isovelocity surface area (PISA) analysis.
All other drugs except beta-blockers were continued before the study. Dobutamine was infused intravenously in an incremental doses of 5, 10, 20 and 40 mcg/kg/min for 3 minute at each stages. End points of the infusion included achievement of target heart rate [85%×(220-age in years)], ST segment elevation or depression on electrocardiography (ECG), new wall motion abnormality, significant arrhythmia such as atrial fibrillation, ventricular tachycardia, atrioventricular block, severe hemodynamic change (systolic blood pressure >200 mm Hg or drop in blood pressure from baseline >20 mm Hg), severe breathlessness. If the target heart rate was not reached with maximal dose of dobutamine, intravenous atropine (300 to 1000 mcg) was administered.
Studies were performed using phased-array ultrasound systems (Vivid 7; GE Medical Systems, Milwaukee, WI, USA) equipped with 3S phased-array transducers. Standard 2D echocardiographic study was performed according to the recommendations of the American Society of Echocardiography using conventional views and measurements.10 Dobutamine stress echocardiography was performed on the same day.
LV end systolic volume (LVESV) and LV end diastolic volume (LVEDV) were measured by the biplane Simpson's disk method. LVEDV and LVESV were corrected (c) by the height of each patient (expressed as cLVEDV, cLVESV).6,7 ΔcLVEDV was defined as [baseline cLVEDV-cLVEDV at peak dobutamine infusion] and ΔcLVESV was defined as [baseline cLVESV-cLVESV at peak dobutamine infusion]. EF was calculated by the equation 100×(EDV-ESV)/EDV. ΔEF was defined as [EF at peak dobutamine infusion-baseline EF]. Degree of MR was quantified by effective regurgitant orifice (ERO) by the PISA method.11 ΔERO was defined as [baseline ERO-ERO at peak dobutamine infusion].
Color tissue Doppler images were acquired at rest and the final 90 seconds of each dobutamine stress. Images were adjusted to optimize pulse repetition frequency, color saturation, and depth to allow for high frame rates. The images were stored and analyzed off-line using customized software (Echopac 6.3, GE, Milwaukee, WI, USA). Sample cursors were placed at the midpoint of each of the 8 non-apical segments of the anterior, inferior lateral, and septal walls in the 2, 4 chamber apical views, and myocardial velocity curves were reconstituted. The time interval to-peak systolic velocity (Ts) was measured from the onset of the QRS complex to the peak of the myocardial systolic velocity during ejection period in each of the eight segments at rest and at peak stress. Ts was corrected for heart rate (Tscor) using Bazett's formula (Tscor=Ts/√R-R interval) to allow for comparison between the Ts of any segment at rest and peak stress.12,13 Dyssynchrony index (DI) was measured as the standard deviation of Tscor of all eight segments. All time interval were calculated as the average of at least three consecutive cardiac cycles. All images acquisition and dyssynchrony analyses were performed by a single investigator blinded to the clinical characteristics of the patients.
A real time 3D echocardiography imaging system (Vivid 7, GE, Milwaukee, WI, USA) with a 2.5-MHz handheld transducer was used to image the mitral apparatus. For all patients, transthoracic volumetric images were obtained with an apical view. Care was taken to include the entire MV in the volumetric data set. The volumetric frame rate was 17 to 22 frames/second with an imaging depth of 12 to 16 cm. All volumetric images were transferred to a personal computer for offline analysis.
We used 3D computer software (4D Cardio-View, Tomtec Co., Munich, Germany) to define measurement planes. First, the mid-systole of the heart cycle was defined. Then, a cross-sectional plane of the MV that clearly visualized both mitral commissures was used to define the commissure-commissure (CC) plane, a plane that passes through both commissures and the LV apex. Finally, anteroposterior (AP) planes perpendicular to the center of the CC axis were defined for imaging of the geometry of the MV. The degree of leaflet tethering was estimated by measuring the angle at which each leaflet met the annular plane (Aα: anterior tethering angle, Pα: posterior tethering angle) in the AP plane. MVTa, the area enclosed by the annular plane, and two leaflets were also measured (Fig. 1). MVTa was c by the height of each patient. ΔcMVTa was defined as [baseline cMVTa-cMVTa at peak dobutamine infusion].
The data were analyzed using SPSS software, version 12, for Windows (SPSS Inc., Chicago, IL, USA). Data are expressed as mean±SD. Continuous variables were described as means and standard deviations and compared using Student's paired t-test. The data at rest and peak stress were compared using Student's paired t-test. Linear regression analysis was applied to study the correlation between change in ERO and various parameters. To determine independent predictors of stress-induced changes in ERO, a stepwise multiple linear regression was performed. p-values <0.05 were considered significant.
Twenty-nine patients with heart failure (M:F=15:14, age: 62±15 yrs) were enrolled. All patients were New York Heart Association functional class I or II. Mean QRS interval [left bundle branch block (LBBB): n=4] was 112±26 ms. The mean EF and ERO were 28% and 0.16±0.09 cm2, respectively. The demographic and clinical characteristics are summarized in Table 1 and 2.
All patients were administered the peak dose of dobutamine (up to 40 mcg/kg/min) with exception of 1 case that reached target heart rate at 20 mcg/kg/min of dobutamine. During dobutamine stress echocardiography, heart rate (78±12 beat/min vs. 109±18 beat/min, p=0.005) and EF (28±8% vs. 39±11%, p=0.001) increased significantly from rest to peak dose. ERO (0.16±0.09 cm2 vs. 0.09±0.08 cm2, p=0.001) significantly reduced after dobutamine infusion in all patients with individually variable changes. There were significant changes in cLVEDV (109.5±38.5 mm3/m vs. 92.1±36.6 mm3/m, p=0.001), cLVESV (80.1±35.2 mm3/m vs. 60.4±31.1 mm3/m, p=0.001), Pα (64±10° vs. 57±11°, p=0.001), Aα (43±8° vs. 25±9°, p=0.001), and cMVTa (1.28±0.48 cm2/m vs. 0.79±0.33 cm2/m, p=0.001) during dobutamine infusion. DI significantly increased from 1.31±0.51 at baseline to 1.58±0.68 at peak dose of dobutamine infusion (p=0.0025) regardless of LBBB. The changes in echocardiographic parameters with dobutamine stress echocardiography are summarized in Table 2. Individual changes in ERO, EF, MVTa, and DI between rest and dobutamine infusion are illustrated in Fig. 2.
Dobutamine-induced ΔERO were significantly correlated with ΔEF and ΔcMVTa (r=0.491, p=0.011 and r=0.499, p=0.009, respectively), but not with ΔDI, ΔcLVEDV, ΔcLVESV, ΔPα, or ΔAα. Correlations between ΔERO and echocardiographic parameters are summarized in Table 3. Based on stepwise multivariate regression analysis, ΔcMVTa and ΔEF (R2=0.443, p=0.001) remained independent determinants of dobutamine-induced changes in ERO (Table 4).
FMR is the result of incomplete closure of normal leaflets without organic mitral lesion. Mitral valvular tenting has been identified as a main determinant of FMR.6 Mitral valvular tenting is characterized by lateral papillary muscle displacement and ventricular dysfunction with reduced transmitral pressure to close the leaflets.
However, FMR varies dynamically with loading conditions that modulate LV volume and mitral valve geometry.
In this study, we attempted to reveal possible factors that contribute to changes in FMR during dobutamine stress. The following are the main findings of our study: during dobutamine infusion, 1) LV systolic function improved and MV tenting area decreased in most of the patients. 2) MR reduced in most of the patients in association with the changes in LV systolic function and MV tenting area. 3) Despite decreases in MR severity, LV dyssynchrony showed no significant improvement in most of the patients.
In our study, ΔMVTa and ΔEF were found to be the strongest independent determinants of MR severity change, while LV dyssynchrony exhibited no significant role therein. These results suggested that MV geometry determined by LV geometry and LV systolic pressure, which represents the MV closing force, may be the primary determinant of MR severity, rather than LV dyssynchrony in DCM.
However, according to observations by Ennezat, et al.8 and D'Andrea, et al.,9 LV dyssynchrony is an independent determinant of changes in FMR during dynamic exercise in patients with congestive heart failure due to LV systolic dysfunction. FMR was aggravated during exercise with deterioration of LV dyssynchrony in DCM.
These inconsistent results with our results might be explained as follows. First, when comparing dobutamine with exercise, there is less increase in arterial blood pressure, representing afterload during dobutamine stress because of the vasodilatory effect of dobutamine. This difference in response of the afterload that had a negative impact on FMR could be a factor responsible for the different FMR responses between exercise and dobutamine.14,15,16 Second, dobutamine stress to detect myocardial contractile reserve shows a tendency to improve LV systolic function and reduce LV chamber size more than exercise.17,18 This might result in more improvement in LV geometry and MV closing force.19 Even though contractile reserve in each segment was not measured, contractile function in segments attached to the papillary muscles might be especially important in terms of improvement of MV geometry. With relevance to clinical situations, considering improvement of LV systolic function and reverse LV remodeling after cardiac resynchronization therapy (CRT), the reverse of the geometry of the mitral apparatus, rather than resynchronization itself, may be regarded as the main reason for the improvement of FMR after CRT.20,21,22 Third, LV mechanical dyssynchrony trends toward a deteriorated state as heart rate increases in patients with non-ischemic LV systolic dysfunction.23,24 As both exercise and dobutamine stress increase heart rate, the increased response of LV dyssynchrony during both exercise and dobutamine stress in DCM might be associated with increased heart rate.8,9,25 Nevertheless, the maximal heart rate achieved by dobutamine stress in the present study seemed to be lower than that by exercise in previous studies.9,12,14 These three factors might explain why FMR responded in a different way in the present study from the previous studies. Nevertheless, further investigation is needed to clarify the differences in the effect on LV function and LV dyssynchrony between exercise and dobutamine.
First, FMR of included patient population was a mild to moderate degree, because the dobutamine stress test was performed on stabilized patients (NYHA functional class I or II) after appropriate medical therapy. Further investigations with more diverse severity of FMR are needed to characterize more precise mechanism associated with dynamic changes in FMR. Second, our assessment of dynamic changes in LV synchronicity was based on a four-basal-four-mid segmental model to assess significant LV dyssynchrony. DI from a relatively small number (8) of segments, compared to other studies (12 segments), and segments in which papillary muscles were attached were not included. Third, the study population was relatively small in the present study. Therefore, further investigation with a larger population is required in the future.
Fourth, we assessed MR severity using the PISA method that assumed the geometry of proximal flow convergence to be hemispherical shape. However, with development of 3D color doppler imaging, PISA, particularly in FMR, has been found to be hemiellipsoidal shape, which suggests that MR severity might be underestimated by the conventional PISA method.26,27
In conclusion, our data showed that dobutamine induced stress reduces MVTa in DCM patients with FMR in association with improvement in LV systolic function representative of MV closing force and decrease in LV chamber size. As a result, FMR severity decreased despite the exacerbation of LV dyssynchrony. This finding suggests that changes in LV geometry and MV closing force, which consequently determine the MV geometry, rather than LV dyssynchrony, may be the primary determinants of the dynamic changes in FMR severity in DCM during dobutamine stress.
Figures and Tables
Fig. 1
Illustrations explaining geometric measurements of the mitral tethering angle (A), mitral valve tenting area (B), cross-sectional volumetric image at the mitral valve level (C), and CC plane (D) connecting both commissures. CC, commissure-commissure; Pα, posterior tethering angle; Aα, anterior tethering angle; AP, anteroposterior; MVTa, mitral valve tenting area; AML, anterior mitral leaflet; PML, posterior mitral leaflet; PC, posterior commissure; AC, anterior commissure.
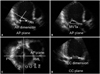
Fig. 2
Individual changes in effective regurgitant orifice, ejection fraction, mitral tenting area, dyssynchrony index between rest, and peak dobutamine infusion (red line: LBBB patients). LBBB, left bundle branch block.
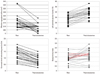
Table 3
Correlations between ΔERO and Echocardiographic Parameters
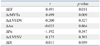
Δ, difference between baseline and peak dose of dobutamine infusion; ERO, effective regurgitant orifice; LVEDV, left ventricle end diastolic volume; LVESV, left ventricle end systolic volume; DI, dyssynchrony index; Pα, posterior tethering angle; Aα, anterior tethering angle; MVTa, mitral valve tenting area; EF, ejection fraction; c, corrected.
References
1. Blondheim DS, Jacobs LE, Kotler MN, Costacurta GA, Parry WR. Dilated cardiomyopathy with mitral regurgitation: decreased survival despite a low frequency of left ventricular thrombus. Am Heart J. 1991; 122(3 Pt 1):763–771.


2. Tahta SA, Oury JH, Maxwell JM, Hiro SP, Duran CM. Outcome after mitral valve repair for functional ischemic mitral regurgitation. J Heart Valve Dis. 2002; 11:11–18.
3. Tomita T, Nakatani S, Eishi K, Takemura T, Takasawa A, Koyanagi H, et al. [Effectiveness of surgical repair of mitral regurgitation concomitant with dilated cardiomyopathy]. J Cardiol. 1998; 32:391–396.
4. Otsuji Y, Handschumacher MD, Schwammenthal E, Jiang L, Song JK, Guerrero JL, et al. Insights from three-dimensional echocardiography into the mechanism of functional mitral regurgitation: direct in vivo demonstration of altered leaflet tethering geometry. Circulation. 1997; 96:1999–2008.


5. Otsuji Y, Handschumacher MD, Liel-Cohen N, Tanabe H, Jiang L, Schwammenthal E, et al. Mechanism of ischemic mitral regurgitation with segmental left ventricular dysfunction: three-dimensional echocardiographic studies in models of acute and chronic progressive regurgitation. J Am Coll Cardiol. 2001; 37:641–648.


6. Yiu SF, Enriquez-Sarano M, Tribouilloy C, Seward JB, Tajik AJ. Determinants of the degree of functional mitral regurgitation in patients with systolic left ventricular dysfunction: a quantitative clinical study. Circulation. 2000; 102:1400–1406.


7. Kwan J, Shiota T, Agler DA, Popović ZB, Qin JX, Gillinov MA, et al. Geometric differences of the mitral apparatus between ischemic and dilated cardiomyopathy with significant mitral regurgitation: real-time three-dimensional echocardiography study. Circulation. 2003; 107:1135–1140.


8. Ennezat PV, Maréchaux S, Le Tourneau T, Lamblin N, Bauters C, Van Belle E, et al. Myocardial asynchronism is a determinant of changes in functional mitral regurgitation severity during dynamic exercise in patients with chronic heart failure due to severe left ventricular systolic dysfunction. Eur Heart J. 2006; 27:679–683.


9. D'Andrea A, Caso P, Cuomo S, Scarafile R, Salerno G, Limongelli G, et al. Effect of dynamic myocardial dyssynchrony on mitral regurgitation during supine bicycle exercise stress echocardiography in patients with idiopathic dilated cardiomyopathy and 'narrow' QRS. Eur Heart J. 2007; 28:1004–1011.
10. Lang RM, Bierig M, Devereux RB, Flachskampf FA, Foster E, Pellikka PA, et al. Recommendations for chamber quantification: a report from the American Society of Echocardiography's Guidelines and Standards Committee and the Chamber Quantification Writing Group, developed in conjunction with the European Association of Echocardiography, a branch of the European Society of Cardiology. J Am Soc Echocardiogr. 2005; 18:1440–1463.


11. Enriquez-Sarano M, Seward JB, Bailey KR, Tajik AJ. Effective regurgitant orifice area: a noninvasive Doppler development of an old hemodynamic concept. J Am Coll Cardiol. 1994; 23:443–451.


12. Stoylen A, Wisløff U, Slørdahl S. Left ventricular mechanics during exercise: a Doppler and tissue Doppler study. Eur J Echocardiogr. 2003; 4:286–291.


13. Thomas VC, Cumbermack KM, Lamphier CK, Phillips CR, Fyfe DA, Fornwalt BK. Measures of dyssynchrony in the left ventricle of healthy children and young patients with dilated cardiomyopathy. J Am Soc Echocardiogr. 2013; 26:142–153.


14. Lapu-Bula R, Robert A, Van Craeynest D, D'Hondt AM, Gerber BL, Pasquet A, et al. Contribution of exercise-induced mitral regurgitation to exercise stroke volume and exercise capacity in patients with left ventricular systolic dysfunction. Circulation. 2002; 106:1342–1348.


15. Keren G, Katz S, Strom J, Sonnenblick EH, LeJemtel TH. Dynamic mitral regurgitation. An important determinant of the hemodynamic response to load alterations and inotropic therapy in severe heart failure. Circulation. 1989; 80:306–313.


16. Heinle SK, Tice FD, Kisslo J. Effect of dobutamine stress echocardiography on mitral regurgitation. J Am Coll Cardiol. 1995; 25:122–127.


17. Keren G, Laniado S, Sonnenblick EH, Lejemtel TH. Dynamics of functional mitral regurgitation during dobutamine therapy in patients with severe congestive heart failure: a Doppler echocardiographic study. Am Heart J. 1989; 118:748–754.


18. Eichhorn EJ, Grayburn PA, Mayer SA, St John Sutoon M, Appleton C, Plehn J, et al. Myocardial contractile reserve by dobutamine stress echocardiography predicts improvement in ejection fraction with beta-blockade in patients with heart failure: the Beta-Blocker Evaluation of Survival Trial (BEST). Circulation. 2003; 108:2336–2341.


19. Tatsumi K, Kawai H, Sugiyama D, Norisada K, Kataoka T, Onishi T, et al. Dobutamine-induced improvement in inferior myocardial contractile function predicts reduction in functional mitral regurgitation: a study using tissue Doppler strain rate imaging. Circ Cardiovasc Imaging. 2010; 3:638–646.


20. Breithardt OA, Sinha AM, Schwammenthal E, Bidaoui N, Markus KU, Franke A, et al. Acute effects of cardiac resynchronization therapy on functional mitral regurgitation in advanced systolic heart failure. J Am Coll Cardiol. 2003; 41:765–770.


21. Kanzaki H, Bazaz R, Schwartzman D, Dohi K, Sade LE, Gorcsan J 3rd. A mechanism for immediate reduction in mitral regurgitation after cardiac resynchronization therapy: insights from mechanical activation strain mapping. J Am Coll Cardiol. 2004; 44:1619–1625.


22. Ypenburg C, Lancellotti P, Tops LF, Boersma E, Bleeker GB, Holman ER, et al. Mechanism of improvement in mitral regurgitation after cardiac resynchronization therapy. Eur Heart J. 2008; 29:757–765.


23. Kurita T, Onishi K, Dohi K, Tanabe M, Fujimoto N, Tanigawa T, et al. Impact of heart rate on mechanical dyssynchrony and left ventricular contractility in patients with heart failure and normal QRS duration. Eur J Heart Fail. 2007; 9:637–643.


24. Plehn G, Vormbrock J, Butz T, Christ M, Trappe HJ, Meissner A. Different effect of exercise on left ventricular diastolic time and interventricular dyssynchrony in heart failure patients with and without left bundle branch block. Int J Med Sci. 2008; 5:333–340.


25. Chattopadhyay S, Alamgir MF, Nikitin NP, Fraser AG, Clark AL, Cleland JG. The effect of pharmacological stress on intraventricular dyssynchrony in left ventricular systolic dysfunction. Eur J Heart Fail. 2008; 10:412–420.


26. Min SY, Song JM, Kim JH, Jang MK, Kim YJ, Song H, et al. Geometric changes after tricuspid annuloplasty and predictors of residual tricuspid regurgitation: a real-time three-dimensional echocardiography study. Eur Heart J. 2010; 31:2871–2880.


27. Matsumura Y, Fukuda S, Tran H, Greenberg NL, Agler DA, Wada N, et al. Geometry of the proximal isovelocity surface area in mitral regurgitation by 3-dimensional color Doppler echocardiography: difference between functional mitral regurgitation and prolapse regurgitation. Am Heart J. 2008; 155:231–238.

