Abstract
Purpose
Gastrointestinal integrity and immune surveillance are affected by stress. Stress also adversely affects mucosal barrier function. β-defensins constitute an integral component of the innate immune system as antimicrobial peptides, serving as the first line of defense against microbial pathogens at the epithelial surfaces of the upper digestive mucosa. The primary objective of this study was to determine the effects of stress on the expression profile of mouse β-defensin-3 in the upper digestive mucosa of mice with diabetes.
Materials and Methods
We established a mouse model of restraint stress by using NSY/Hos mice with type 2 diabetes mellitus. We used real-time polymerase chain reaction, in situ hybridization, and immunohistochemistry to investigate the effects of stress and glucocorticoid administration on mouse β-defensin-3 expression in the upper digestive mucosa of the gingiva, esophagus, and stomach.
Results
Mouse β-defensin-3 mRNA expression was higher in the esophagus than in the gingiva or stomach (p<0.05). In the esophagus, mouse β-defensin-3 mRNA expression was lower in stressed mice than in non-stressed mice (p<0.05). Furthermore, immunoreactivity to mouse β-defensin-3 protein was lower in the esophagus of stressed mice than non-stressed mice, consistent with the results of mRNA expression analysis. Systemic glucocorticoid administration also downregulated esophageal mouse β-defensin-3 mRNA expression.
Conclusion
Our novel findings show that stress decreases mouse β-defensin-3 expression in the esophagus of mice with diabetes, possibly due to increased endogenous glucocorticoid production. It appears to be highly likely that stress management may normalize mucosal antimicrobial defenses in patients with diabetes.
Psychological and physical stress can negatively affect gastrointestinal integrity,1,2 immune surveillance,3 and wound healing.4-6 Stress is also associated with gastrointestinal ulceration7 and impaired wound healing in the skin.8 In humans and mice, stress attenuates immune system responses and the function of permeability barriers.9-13 In patients with diabetes mellitus (DM), impaired wound healing in the skin (e.g., foot lacerations) represents a potentially serious condition, as diminished immune responses are major factors in the development of skin ulceration and infection.14,15
Defensins are small cationic antimicrobial peptides that contain disulfide bonds and are an integral component of host innate immune defenses against microbial pathogens. Defensins are classified into α and β subfamilies.16 The α-defensins are found in human neutrophils, while β-defensins are produced by the epithelial cells of many organs, including the digestive mucosa. β-defensins exhibit broad-spectrum of activity against bacteria, viruses, and fungi, particularly at the epithelial interface of mucosal surfaces.17,18 Thus, β-defensins maintain homeostatic levels of commensal bacteria and protect against colonization by pathogenic microbes, constituting the first line of immune defense.19
Human β-defensin (hBD)-1 is expressed at a low level with little regulation in response to infection or other stimuli, whereas under normal conditions, hBD-2 and hBD-3 are generally expressed at low levels in vivo. hBD-2 is expressed in the mucosal epithelium and exhibits potent antimicrobial activity against a variety of pathogens.20 A murine homologue of hBD-2 has been named as mouse β-defensin-3 (mBD-3).21
Psychological stress leads to the downregulation of mBD-3 expression in the epidermis due to increased production of endogenous glucocorticoids (GCs).11 The stress response is orchestrated by the hypothalamic-pituitary-adrenal (HPA) axis via corticosteroids. The negative consequences of stress on epithelial functions such as permeability barrier homeostasis22-24 could be ascribed to a stress-induced increase in circulating levels of GCs;11 however, the relationship between stress-related GC levels and β-defensin expression in the upper digestive mucosa is poorly understood. The primary objective of this study was to determine the effect of stress on mBD-3 expression in the upper digestive mucosa of NSY/Hos mice.
Eight-week-old male NSY/Hos mice, a model for type 2 diabetes, were purchased from SLC (Shizuoka, Japan) and used in the experiments at 26-27 weeks of age. The mice were housed in groups of 5 animals per cage in a specific pathogen-free room and maintained under a standardized 12-h light/dark cycle (lights on at 0700) and constant temperature (22±3℃). All the mice were fed a high-fat diet25 and water containing 30% sucrose.26 All animal experiments were conducted in accordance with accepted standards of animal care, under protocols developed according to the Guidelines for Animal Experimentation of Kanagawa Dental University, which was based on the Guidelines for the Regulation of Animal Experimentation: International Committee on Laboratory Animals and approved by the committee of Ethics on Animal Experiments of Kanagawa Dental University.
Mice were used for restraint-induced stress experiments at 27 weeks of age. Five mice were subjected to restraint stress and 5 were used as non-stressed controls. Stress was induced by enclosing each mouse in a flexible wire mesh (5×5 mm) shaped to fit its body. During restraint, the mice could move only minimally and had no access to food or water. Because mice show nocturnal behavior, they were restrained from 1800 to 1000 h (16 h) for 3 consecutive days. After release from the restraining mesh, they were returned to their cages and given access to food and water, as were the controls.27,28
Four 26-week-old mice were used to assess the effect of systemic GC on β-defensin expression. Systemic GC was administered by intraperitoneal injection of 9 µg of dexamethasone (450 µg/kg) in saline. Mice were injected 3 times over 3 days.11 The dose was chosen based upon a previous report concerning the function of the epidermal permeability barrier.10,12,29 An equal number of control mice received intraperitoneal administrations of the vehicle alone.
At the end of the experiment on the third day, the mice used in the restraint experiment were deeply anesthetized with sevoflurane and sodium pentobarbital (65 mg/kg, i.p.), and blood samples were collected by axillary puncture between 1000 and 1200 h. For measurement of plasma corticosterone levels, blood samples were collected into Venoject tubes containing ethylene diamine tetraacetic acid (Terumo, Tokyo, Japan). The tubes were immediately placed on ice and then centrifuged (2000 rpm, 15 min, 4℃), after which the plasma was separated and plasma corticosterone levels were determined (SRL Inc., Tokyo, Japan). Blood samples were also collected into BD Vacutainer® Plus plastic tubes containing sodium fluoride and disodium EDTA (Becton Dickinson, Franklin Lakes, NJ, USA) for measurement of blood glucose (Medical Labo Inc., Kanagawa, Japan).
Immediately following blood collection from mice used in restraint-induced stress and GC administration experiments, the anesthetized animals were sacrificed by decapitation. The upper digestive mucosa, including the gingiva, esophagus, and stomach, were removed between 1000 and 1200 h. Esophagus samples were obtained from all mice from both experiments, while samples of gingiva and stomach were obtained only from mice used in the restraint-induced stress experiment.
Total RNA was isolated from each tissue sample by using ISOGEN Reagent (Nippon Gene Co. Ltd., Toyama, Japan) according to the manufacturer's instructions. RNA quality was judged from the ribosomal RNA pattern after electrophoresis on a 1.5% agarose gel containing ethidium bromide and visualization by ultraviolet illumination. RNA concentrations were determined using a Bio Spec-nano spectrophotometer (Shimadzu Access Corp., Kanagawa, Japan). Complementary DNA (cDNA) was synthesized from total RNA by using a First-strand cDNA Synthesis Kit (Roche Diagnostics Ltd., Lewes, UK). Real-time polymerase chain reaction (PCR) was performed using a LightCycler system (Roche).
The primer sequences used to amplify the mBD-3 gene sequence were 5'-GCT TCA GTC ATG AGG ATC CAT TAC CTT C-3' (forward) and 5'-CGG GAT CTT GGT CTT CTC TA-3' (reverse; PCR product: 218 bp), designed and synthesized by Nippon Gene Research Laboratory (Miyagi, Japan). PCR amplification of mBD-3 was performed as follows: 95℃ for 10 min, followed by 40 cycles of 95℃ for 10 s, 60℃ for 15 s, and 72℃ for 15 s. Mouse β-actin was used as a housekeeping control and amplified using LightCycler Primer sets (Search-LC, Heidelberg, Germany; 95℃ for 10 min followed by 40 cycles of 95℃ for 10 s, 60℃ for 10 s, and 72℃ for 10 s). Gene expression is reported as the ratio of the mRNA copy number for the target gene to that for β-actin for each sample.
Esophageal samples were obtained from animals that had been subjected to restraint-induced stress; the samples were fixed in 4% paraformaldehyde and 10%, 15%, and 20% sucrose, and then embedded in Optimal Cutting Temperature compound® (Sakura, Tokyo, Japan) in liquid nitrogen. Tissues were stained with hematoxylin and eosin according to standard techniques to assess morphological changes. Immunohistochemical analyses were performed using a Histofine Staining Kit (Nichirei, Tokyo, Japan).
Frozen tissue sections (3 µm thick) were preincubated in 3% H2O2 for 8 min. After washing in phosphate-buffered saline (PBS), the sections were incubated with anti-mBD-3 polyclonal antibody (1:50; Santa Cruz Biotechnology Inc., Santa Cruz, CA, USA) for 1 h at room temperature, and were then treated with rabbit peroxidase-labeled secondary antibody (Nichirei) for 10 min at room temperature. The chromogen was 3,3'-diaminobenzidine-tetrahydrochloride in 0.003% H2O2 in PBS. The sections were counterstained with hematoxylin. As a negative control, PBS was used in place of primary antibody.
Complementary RNA (cRNA) probes were produced by in vitro transcription of linearized pGEM-T Easy Vector (Promega, Madison, WI, USA). The probes consisted of chemically synthesized 120-mer sense and antisense oligonucleotides specific for mBD-3 (nucleotides 38-157 and 177-296 of the mBD-3 coding sequence; accession number, NM_ 013756.2). Digoxigenin (DIG)-11-UTP-labeled single-stranded cRNA probes for mBD-3 were prepared using a DIG RNA Labeling Kit (SP6/T7; Roche). The in situ hybridization procedures have been described previously.30-32 Paraffin sections (3 µm thick) were digested with proteinase K (1 µg/mL) for 20 min at 37℃. Hybridization was performed at 37℃ for 16 h by using DIG-11-UTP-labeled single-stranded cRNA probes dissolved in hybridization medium (Wako Pure Chemical Industries, Tokyo, Japan). After hybridization, mRNA was detected colorimetrically using a DIG non-radioactive nucleic acid detection kit (Roche).
Statistical analyses were carried out using SPSS software, version 17.0 (SPSS Inc., Chicago, IL, USA). The results are reported as mean±SD values. Differences in plasma corticosterone and blood glucose levels were analyzed using the Mann-Whitney U test. mBD-3 mRNA expression was analyzed by multiple comparisons in 3 groups using the Kruskal-Wallis test; if the differences were significant, they were analyzed using the Mann-Whitney U test in each of the 2 groups. mBD-3 mRNA expression is described in terms of the relative copy number ratio of mBD-3 to β-actin mRNA for each sample. p values <0.05 were considered statistically significant.
Blood glucose levels were higher in stressed mice (296.40±78.85 mg/dL, n=5) than in non-stressed control mice (236.80±40.10 mg/dL, n=5), but the difference was not significant (Fig. 1A). Plasma corticosterone levels were significantly higher (p<0.05) in stressed mice (183.80±35.73 ng/mL, n=5) than in non-stressed mice (126.44±25.89 ng/mL, n=5) (Fig. 1B). These results confirmed that the restraint conditions used induce chronic stress in mice.
Melting curve analysis demonstrated the presence of a single fluorescence peak representing mBD-3 mRNA in all samples (data not shown). Furthermore, a single band was observed on agarose gel electrophoresis (data not shown). These findings confirmed that the PCR product was mBD-3 mRNA.
mBD-3 mRNA expression was significantly higher in the esophagus (0.03645±0.02405, n=4) than in the gingiva (0.01025±0.005909, n=4) or stomach (0.000534±0.0007273, n=4) (p<0.05 for all) (Fig. 2). Furthermore, mBD-3 mRNA expression was significantly higher (p<0.05) in the gingiva than in the stomach.
Restraint-induced stress did not significantly alter mBD-3 mRNA expression in the gingiva (stressed mice, 0.00975±0.007932, n=4; non-stressed mice, 0.01025±0.005909, n=4) (Fig. 3A). However, mBD-3 mRNA expression was significantly lower (p<0.05) in the esophagus of stressed mice (0.00442±0.002369, n=4) than in non-stressed mice (0.03645±0.02405, n=4) (Fig. 3B). Restraint-induced stress did not significantly alter mBD-3 mRNA expression in the stomach (stressed mice, 0.000494±0.0005212, n=4; non-stressed mice, 0.000534±0.0007273, n=4) (Fig. 3C).
Moderate mBD-3 mRNA signals were seen in the cytoplasm of epithelium cells in the esophagus of stressed mice, but nonspecific signals were observed in the cell nuclei of sections reacted with antisense probes (Fig. 5A). In non-stressed mice, positive mBD-3 mRNA signals were noted in the cytoplasm of epithelial cells, whereas nonspecific signals were seen in the nuclei (Fig. 5B). These signals were not localized in the keratinized layers but were localized in the basal and suprabasal layers of the epithelium in the esophagus. The sense probes did not show hybridization signals in the epithelium of the esophagus (Fig. 5C).
To analyze whether decreased mBD-3 expression induced by restraint stress can be attributed to increased GC, we administered dexamethasone intraperitoneally and assessed mBD-3 expression by real-time PCR. mBD-3 mRNA expression was significantly (p<0.05) lower in the esophagus (0.00004±0.0000197) of mice treated with systemic GCs than in the esophagus of control mice (0.000124025±0.0000419) (Fig. 6).
Diabetes is an extremely common lifestyle-related disease. Because it is associated with various complications, patients must pay close attention to their lifestyle. Stress increases blood glucose levels and negatively affects immune function throughout the body and may thus increase the likelihood of infection. In mice, mBD-3 is an important component of the innate immune system and is one of the first lines of defense against infection in the mucosa of the upper digestive tract.21,33 However, it is unclear how stress affects mBD-3 expression in patients with diabetes.
In this study, the mean blood glucose level of non-stressed control NSY/Hos mice was 236 mg/dL. NSY/Hos mice were established as an inbred animal model for spontaneous type 2 DM by selective breeding for glucose intolerance within a closed colony of Jcl:ICR mice.34 Based on previous reports, our NSY/Hos mice were fed a high-fat diet and water containing 30% sucrose.25,26 Previous studies have reported blood glucose levels of around 150 mg/dL in NSY/Hos mice.35 In our study, we established mice that had diabetes with hyperglycemia.
Plasma corticosterone levels were significantly higher in stressed mice than in non-stressed mice; mice were subjected to 16 h of restraint-induced stress each day for 3 d by confining them in a flexible wire mesh, as described in our previous reports.36 Psychological and physical stresses increase plasma corticosterone in rodents, and we found that chronic stress increased plasma corticosterone levels. Our results indicated that we were able to induce hyperglycemia with type 2 diabetes and establish a chronic stress model in mice. Furthermore, the mean blood glucose level in the stressed mice was 296 mg/dL, which was higher than that in non-stressed mice, but not significantly different. In general, an increased stress load results in increased blood glucose levels, although the increase was insignificant in our experiments. Because blood glucose levels were already elevated in stressed mice because of hyperglycemia, the stress might not have increased the levels any further.
The level of mBD-3 mRNA expression was as follows (in this order): esophagus>gingiva>stomach. Furthermore, the mBD-3 mRNA level was higher in the gingiva than in the stomach. These differences may be related to differences in mucosal surface structure of the gingiva, esophagus, and stomach. Minimal mBD-3 mRNA expression was noted in the stomach mucosa, which consists of a simple columnar epithelium. The mechanical barrier of the columnar epithelium is inferior to that of squamous epithelium in the upper digestive mucosa. Expression of the mBD-3 homologue hBD-2 is very low in the human stomach during non-Helicobacter pylori infection, suggesting another important antimicrobial mechanism in the stomach. Furthermore, hBD-2 expression is much higher in the healthy esophagus than the stomach.33 The role of mBD-3 may be minimal in the stomach mucosal epithelium. Furthermore, the stratified squamous epithelium presents a strong barrier against bacterial infection, and keratinization provides a particularly effective defense. Because the degree of keratinization in the esophagus is lower than that in the gingiva, the level of mechanical protection in the esophagus is lower. These observations are consistent with our finding that mBD-3 expression was higher in the esophagus than in the gingiva. hBD-2 plays an important role in defending the oral mucosa against bacterial infection.21 Our novel data regarding differences in β-defensin expression in the upper digestive mucosa of mice with diabetes suggest that the innate protection provided by mBD-3 in the upper digestive mucosa is the most important factor in preventing esophageal infection.
The results of our restraint-induced stress model indicated that mBD-3 expression was downregulated only in the esophagus. It has been clearly established that stress adversely affects the health of the upper digestive tract.1,2,7,9-13 Aberg, et al.11 reported that stress decreases mBD-3 expression in normal mouse epidermis. Interestingly, they also found that the stress-related decline in mBD-3 expression increased the severity of bacterial infection. These reports agree with our present results that stress downregulates mBD-3 mRNA expression in the esophagus, thereby depressing innate immunity. These results together suggest that stress can adversely affect esophageal health in patients with diabetes.
No mBD-3 immunoreactivity was detected in the esophagus of stressed mice, while an mBD-3 positive reaction was detected in non-stressed mice. These results were consistent with mBD-3 mRNA expression analysis by real-time PCR. Thus, in the esophagus, stress results in downregulation of mBD-3 mRNA expression and mBD-3 protein expression. Additionally, mBD-3 was detected in the keratinized layers in non-stressed mice. These results were similar to those of previous reports indicating that the keratinized layers often exhibit the highest expression of β-defensin protein in humans, and may therefore be a site of β-defensin retention.37 These observations suggest that, in non-stressed mice, mBD-3 protein localizes in the keratinized layers of the esophagus to maintain homeostatic levels of commensal bacteria and protect against colonization by pathogenic microbes.
Although mBD-3 mRNA was detected in the suprabasal layers, mBD-3 protein was localized in the keratinized layers of the esophagus in non-stressed mice. Dale, et al.38 reported that the distribution of β-defensin mRNA and protein differs in the human gingival epithelium. More importantly, we found that the mRNA and protein levels of mBD-3 in the esophagus were lower in stressed mice than in non-stressed mice. Thus, both mBD-3 mRNA and protein expression may be downregulated by stress.
Dexamethasone administration led to downregulation of mBD-3 mRNA expression in the esophagus. The effects of dexamethasone include inhibition of the immune system and reduction of inflammation. Endogenous GCs produced by the adrenal gland yield similar effects. Stress results in increased plasma levels of endogenous GCs via activation of the HPA axis.39-41 The adverse effect of stress on mucosal barrier function is reported to be mediated by increased levels of GC;12,13 thus, the results obtained from dexamethasone administration can experimentally explain the negative effects of stress on host defenses. We administered dexamethasone at the same supraphysiologic dose described in previous reports.13,29 Recent reports indicate that mBD-3 protein expression in the mouse epidermis is reduced by exposure to stress or administration of dexamethasone.11 Reports indicating that stress-induced production of endogenous GCs negatively affects β-defensin expression were confirmed by observations that blockading GCs through systemic administration of GC inhibitors can normalize mBD-3 protein expression during stress.11 Our results suggest that increased GC may play an important role in the decrease in mBD-3 expression in the esophagus, and indicate that the effects of stress are mediated by increased levels of endogenous GCs.
We assessed mBD-3 expression in the upper digestive mucosa, and high mBD-3 expression was seen in the esophagus. Additionally, our results suggest that the stress-induced decline in mBD-3 expression was mediated by increased GC levels in mice with hyperglycemia. Validation of our results would suggest that stress management could be an important approach for minimizing the adverse effects of stress on innate immunity in patients with diabetes.
Figures and Tables
Fig. 1
Blood glucose and plasma corticosterone levels in stressed and non-stressed mice. Blood glucose (A) and plasma corticosterone levels (B) in stressed and non-stressed mice (n=5), represented as mean±SD values. Statistical comparisons were made individually (between stressed and non-stressed mice). Statistical significance was observed for plasma corticosterone levels (p<0.05), but not for blood glucose levels.
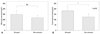
Fig. 2
Expression of mBD-3 in the upper digestive mucosa. Real-time PCR results for mBD-3 in the upper digestive mucosa (n=4). The results are represented as mean±SD values. Statistical comparisons were made among the mBD-3 expression in the gingiva, esophagus, and stomach, and statistical significance was seen for mBD-3 among the 3 groups (p<0.05 for all). mBD-3, mouse β-defensin-3; PCR, polymerase chain reaction.
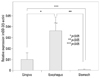
Fig. 3
mBD-3 mRNA expression in the upper digestive mucosa in stressed and non-stressed mice. Real-time PCR results for mBD-3 expression in gingival (n=4) (A), esophageal (n=4) (B), and stomach (n=4) (C) tissue from stressed and non-stressed mice. The results are represented as mean±SD values. Statistical comparisons were made individually (between stressed and non-stressed mice); significant differences were seen between the 2 groups for the mBD-3 mRNA expression in the esophagus but not in the gingiva or stomach (p<0.05). mBD-3, mouse β-defensin-3; PCR, polymerase chain reaction.
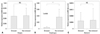
Fig. 4
mBD-3 protein expression in the esophagus of stressed and non-stressed mice by immunohistochemical stain. The localization of mBD-3 in the esophagus in stressed mice (A) and non-stressed mice (B) is shown by immunohistochemical results. Positive mBD-3 immunoreactivity was found in the keratinized layers of non-stressed mice, but not of stressed mice. Scale bar reveals 20 µm. mBD-3, mouse β-defensin-3.
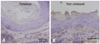
Fig. 5
ISH analysis of mBD-3 mRNA expression in the esophagus of stressed and non-stressed mice. The mBD-3 signals in stressed (A) and non-stressed mice (B) are shown by ISH with antisense probes. Positive mBD-3 signals were found in non-stressed mice, but not in stressed mice. No mBD-3 signals were observed with sense probes (C). Scale bar reveals 20 µm. ISH, in situ hybridization; mBD-3, mouse β-defensin-3.
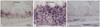
Fig. 6
mBD-3 mRNA expression in the esophagus of GC-treated and untreated mice. Real-time PCR results for mBD-3 expression in the esophagus of GC-treated and untreated mice (n=4). The results are represented as mean±SD values. Statistical comparisons were made individually (between GC-treated and untreated mice); mBD-3 expression significantly differed between GC-treated and untreated mice (p<0.05). GC, glucocorticoid; mBD-3, mouse β-defensin-3; PCR, polymerase chain reaction.
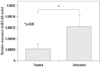
ACKNOWLEDGEMENTS
The authors thank Yukiko Ito for her help with real-time PCR and excellent technical assistance.
References
1. Meddings JB, Swain MG. Environmental stress-induced gastrointestinal permeability is mediated by endogenous glucocorticoids in the rat. Gastroenterology. 2000; 119:1019–1028.


2. Mawdsley JE, Rampton DS. Psychological stress in IBD: new insights into pathogenic and therapeutic implications. Gut. 2005; 54:1481–1491.


3. Glaser R. Stress-associated immune dysregulation and its importance for human health: a personal history of psychoneuroimmunology. Brain Behav Immun. 2005; 19:3–11.


4. Marucha PT, Kiecolt-Glaser JK, Favagehi M. Mucosal wound healing is impaired by examination stress. Psychosom Med. 1998; 60:362–365.


5. Ebrecht M, Hextall J, Kirtley LG, Taylor A, Dyson M, Weinman J. Perceived stress and cortisol levels predict speed of wound healing in healthy male adults. Psychoneuroendocrinology. 2004; 29:798–809.


6. Horan MP, Quan N, Subramanian SV, Strauch AR, Gajendrareddy PK, Marucha PT. Impaired wound contraction and delayed myofibroblast differentiation in restraint-stressed mice. Brain Behav Immun. 2005; 19:207–216.


7. Gudeman SK, Wheeler CB, Miller JD, Halloran LG, Becker DP. Gastric secretory and mucosal injury response to severe head trauma. Neurosurgery. 1983; 12:175–179.


8. Kiecolt-Glaser JK, Marucha PT, Malarkey WB, Mercado AM, Glaser R. Slowing of wound healing by psychological stress. Lancet. 1995; 346:1194–1196.


9. Martin-Ezquerra G, Man MQ, Hupe M, Rodriguez-Martin M, Youm JK, Trullas C, et al. Psychological stress regulates antimicrobial peptide expression by both glucocorticoid and β-adrenergic mechanisms. Eur J Dermatol. 2011; 21:Suppl 2. 48–51.


10. Choi EH, Brown BE, Crumrine D, Chang S, Man MQ, Elias PM, et al. Mechanisms by which psychologic stress alters cutaneous permeability barrier homeostasis and stratum corneum integrity. J Invest Dermatol. 2005; 124:587–595.


11. Aberg KM, Radek KA, Choi EH, Kim DK, Demerjian M, Hupe M, et al. Psychological stress downregulates epidermal antimicrobial peptide expression and increases severity of cutaneous infections in mice. J Clin Invest. 2007; 117:3339–3349.


12. Choi EH, Demerjian M, Crumrine D, Brown BE, Mauro T, Elias PM, et al. Glucocorticoid blockade reverses psychological stress-induced abnormalities in epidermal structure and function. Am J Physiol Regul Integr Comp Physiol. 2006; 291:R1657–R1662.


13. Denda M, Tsuchiya T, Elias PM, Feingold KR. Stress alters cutaneous permeability barrier homeostasis. Am J Physiol Regul Integr Comp Physiol. 2000; 278:R367–R372.


14. Goodson WH 3rd, Hung TK. Studies of wound healing in experimental diabetes mellitus. J Surg Res. 1977; 22:221–227.


15. Bader MS. Diabetic foot infection. Am Fam Physician. 2008; 78:71–79.
16. Diamond G, Zasloff M, Eck H, Brasseur M, Maloy WL, Bevins CL. Tracheal antimicrobial peptide, a cysteine-rich peptide from mammalian tracheal mucosa: peptide isolation and cloning of a cDNA. Proc Natl Acad Sci U S A. 1991; 88:3952–3956.


18. Diamond G, Beckloff N, Ryan LK. Host defense peptides in the oral cavity and the lung: similarities and differences. J Dent Res. 2008; 87:915–927.


19. Diamond G, Ryan L. Beta-defensins: what are they really doing in the oral cavity? Oral Dis. 2011; 17:628–635.


20. Bals R, Wang X, Meegalla RL, Wattler S, Weiner DJ, Nehls MC, et al. Mouse beta-defensin 3 is an inducible antimicrobial peptide expressed in the epithelia of multiple organs. Infect Immun. 1999; 67:3542–3547.


21. Abiko Y, Saitoh M, Nishimura M, Yamazaki M, Sawamura D, Kaku T. Role of beta-defensins in oral epithelial health and disease. Med Mol Morphol. 2007; 40:179–184.


22. Denda M, Tsuchiya T, Hosoi J, Koyama J. Immobilization-induced and crowded environment-induced stress delay barrier recovery in murine skin. Br J Dermatol. 1998; 138:780–785.


23. Altemus M, Rao B, Dhabhar FS, Ding W, Granstein RD. Stress-induced changes in skin barrier function in healthy women. J Invest Dermatol. 2001; 117:309–317.


24. Garg A, Chren MM, Sands LP, Matsui MS, Marenus KD, Feingold KR, et al. Psychological stress perturbs epidermal permeability barrier homeostasis: implications for the pathogenesis of stress-associated skin disorders. Arch Dermatol. 2001; 137:53–59.
25. Anai M, Funaki M, Ogihara T, Kanda A, Onishi Y, Sakoda H, et al. Enhanced insulin-stimulated activation of phosphatidylinositol 3-kinase in the liver of high-fat-fed rats. Diabetes. 1999; 48:158–169.


26. Nojima K, Ikegami H, Fujisawa T, Ueda H, Babaya N, Itoi-Babaya M, et al. Food hardness as environmental factor in development of type 2 diabetes. Diabetes Res Clin Pract. 2006; 74:1–7.


27. Nakajima K, Hamada N, Takahashi Y, Sasaguri K, Tsukinoki K, Umemoto T, et al. Restraint stress enhances alveolar bone loss in an experimental rat model. J Periodontal Res. 2006; 41:527–534.


28. Saruta J, Iida M, Kondo Y, To M, Hayashi T, Hori M, et al. Chronic stress induces neurotrophin-3 in rat submandibular gland. Yonsei Med J. 2012; 53:1085–1092.


29. Kao JS, Fluhr JW, Man MQ, Fowler AJ, Hachem JP, Crumrine D, et al. Short-term glucocorticoid treatment compromises both permeability barrier homeostasis and stratum corneum integrity: inhibition of epidermal lipid synthesis accounts for functional abnormalities. J Invest Dermatol. 2003; 120:456–464.


30. To M, Kamata Y, Saruta J, Shimizu T, Sato T, Kondo Y, et al. Induction of β-Defensin Expression by Porphyromonas gingivalis-Infected Human Gingival Graft Transplanted in nu/nu Mouse Subdermis. Acta Histochem Cytochem. 2013; 46:25–34.


31. Kondo Y, Saruta J, To M, Shiiki N, Sato C, Tsukinoki K. Expression and Role of the BDNF Receptor-TrkB in Rat Adrenal Gland under Acute Immobilization Stress. Acta Histochem Cytochem. 2010; 43:139–147.


32. Saruta J, Fujino K, To M, Tsukinoki K. Expression and localization of brain-derived neurotrophic factor (BDNF) mRNA and protein in human submandibular gland. Acta Histochem Cytochem. 2012; 45:211–218.


33. Hosaka Y, Koslowski M, Nuding S, Wang G, Schlee M, Schäfer C, et al. Antimicrobial host defense in the upper gastrointestinal tract. Eur J Gastroenterol Hepatol. 2008; 20:1151–1158.


34. Shibata M, Yasuda B. New experimental congenital diabetic mice (NSY mice). Tohoku J Exp Med. 1980; 130:139–142.
35. Takeda S, Sato N, Uchio-Yamada K, Sawada K, Kunieda T, Takeuchi D, et al. Diabetes-accelerated memory dysfunction via cerebrovascular inflammation and Abeta deposition in an Alzheimer mouse model with diabetes. Proc Natl Acad Sci U S A. 2010; 107:7036–7041.


36. Saruta J, Kondo Y, Sato C, Shiiki N, Tsukinoki K, Sato S. Salivary glands as the source of plasma brain-derived neurotrophic factor in stressed rats engaged in biting behavior. Stress. 2010; 13:238–247.


37. Abiko Y, Suraweera AK, Nishimura M, Arakawa T, Takuma T, Mizoguchi I, et al. Differential expression of human beta-defensin 2 in keratinized and non-keratinized oral epithelial lesions; immunohistochemistry and in situ hybridization. Virchows Arch. 2001; 438:248–253.


38. Dale BA, Kimball JR, Krisanaprakornkit S, Roberts F, Robinovitch M, O'Neal R, et al. Localized antimicrobial peptide expression in human gingiva. J Periodontal Res. 2001; 36:285–294.


39. Gomez-Merino D, Drogou C, Chennaoui M, Tiollier E, Mathieu J, Guezennec CY. Effects of combined stress during intense training on cellular immunity, hormones and respiratory infections. Neuroimmunomodulation. 2005; 12:164–172.

