Abstract
Purpose
UGT1A1, UGT2B7, and UGT2B15 are well-known pharmacogenes that belong to the uridine diphosphate glucuronyltransferase gene family. For personalized drug treatment, it is important to study differences in the frequency of core markers across various ethnic groups. Accordingly, we screened single nucleotide polymorphisms (SNPs) of these three genes and analyzed differences in their frequency among five ethnic groups, as well as attempted to predict the function of novel SNPs.
Materials and Methods
We directly sequenced 288 subjects consisting of 96 Korean, 48 Japanese, 48 Han Chinese, 48 African American, and 48 European American subjects. Subsequently, we analyzed genetic variability, linkage disequilibrium (LD) structures and ethnic differences for each gene. We also conducted in silico analysis to predict the function of novel SNPs.
Results
A total of 87 SNPs were detected, with seven pharmacogenetic core SNPs and 31 novel SNPs. We observed that the frequencies of UGT1A1 *6 (rs4148323), UGT1A1 *60 (rs4124874), UGT1A1 *93 (rs10929302), UGT2B7 *2 (rs7439366), a part of UGT2B7 *3 (rs12233719), and UGT2B15 *2 (rs1902023) were different between Asian and other ethnic groups. Additional in silico analysis results showed that two novel promoter SNPs of UGT1A1 -690G>A and -689A>C were found to potentially change transcription factor binding sites. Moreover, 673G>A (UGT2B7), 2552T>C, and 23269C>T (both SNPs from UGT2B15) changed amino acid properties, which could cause structural deformation.
Uridine diphosphate glucuronyl transferase (UGT) is an enzyme that catalyzes the addition of glucuronic acid to a substrate, known as a glucuronidation reaction, which plays a major role in phase II drug metabolism.1,2 The enzyme is expressed in most parts of the body, including liver, kidney, intestine, lung, skin, prostate, and brain. In this study, we have selected three genes of the UGT gene family, uridine diphosphate glucuronosyl transferase 1 family, polypeptide A1 (UGT1A1); uridine diphosphate glucuronosyl transferase 2 family, polypeptide B7 (UGT2B7); and uridine diphosphate glucuronosyl transferase 2 family, polypeptide B15 (UGT2B15), and then sequenced for single nucleotide polymorphisms (SNPs) in the three UGT genes. We also calculated and compared allele frequencies, linkage disequilibrium (LD), and haplotype structures in five ethnic groups consisting of Korean, Han Chinese, Japanese, African American, and European American subjects.
UGT1A1 is located on chromosome 2q37, and encodes for a part of the UGT enzyme. The gene is also known to have several isoforms due to the splicing of exons at various locations. A preferred substrate for the enzyme is bilirubin, which is hypothesized to be a cellular antioxidant in the human body.3 Previous studies have found that polymorphisms of UGT1A1 are associated with diseases such as head and neck cancers, colorectal cancer, Gilbert syndrome, and coronary artery disease.4-7 Located on chromosome 4q13, UGT2B7 is known to glucosidate hydesoxycholic acid in the liver, as well as glucuronidate steroid hormones and fatty acids.8,9 It also conjugates various classes of major drugs including analgesics, carboxylic non-steroidal anti-inflammatory drugs, and anti-carcinogens.9 In addition, UGT2B7 polymorphisms were found to have associations with an increased risk of bladder cancer, colorectal cancer, and a treatment method for breast cancer.10-12 UGT2B15 is also located on chromosome 4q13, and encodes for an enzyme that has unique conjugation with xenobiotic and endogenous compounds, such as 7-hydroxylated coumarins, flavonoids, anthraquinones, and other drugs.13 Furthermore, UGT2B15 is also known to glucuronidate anti-anxiety drugs such as lorazepam and oxazepam.14,15
In this study, we directly sequenced 288 genomic DNA samples from diverse ethnicities, which yielded 87 SNPs in UGT1A1, UGT2B7, and UGT2B15. Our results might aid research on pharmacogenomics of these important genes.
DNA from a total of 288 subjects was used in the present study and was obtained from 96 Koreans, 48 African Americans, 48 European Americans, 48 Japanese, and 48 Han Chinese subjects. The 96 Korean DNA samples were unrelated population controls. Other ethnic groups were obtained as anonymous, unrelated DNA samples from the Human Variation Panels prepared by the Coriell Institute (Camden, NJ, USA).
Promoters, all exons, and exon-intron boundaries (+/- 50 bp) were polymerase chain reaction-amplified and directly sequenced using a ABI PRISM 3730 genetic analyzer (Applied Biosystems, Foster City, CA, USA). Primers for the amplification and sequencing analysis were designed by using Primer3 software (http://frodo.wi.mit.edu) based on the sequence of respective genes (Ref. genome seq.: NG_002601.2, AC_111000.3, and NT_167250.1 for UGT1A1, UGT2B7, and UGT2B15, respectively) (Supplementary Table 1, only online).16 Sequence variants were verified by chromatograms using SeqMan software.
The χ2 tests were used to determine whether individual variants were in Hardy-Weinberg equilibrium at each locus in each ethnic group. Pairwise LD was estimated for the SNPs in each gene using standardized summary statistics D' and r2,17 which were calculated using HaploView software.18 Haplotype blocks were assigned using the D' confidence interval algorithm also using HaploView. For in silico analysis, we used the FastSNP program to predict the potential binding site changes caused by promoter SNPs.19 Fisher's exact test was calculated using the Statistical Analysis System 9.2 (SAS Institute, Cary, NC, USA).
We included a total of 288 subjects (96 Koreans and 48 Han Chinese, 48 Japanese, 48 African American and 48 European American) for direct sequencing of UGT1A1, UGT2B7 and UGT2B15. As a result, we detected 33 SNPs from UGT1A1, 18 SNPs from UGT2B7, and 36 SNPs from UGT2B15 (Table 1). All SNPs were in Hardy-Weinberg equilibrium for five ethnic groups (data not shown). Among the observed SNPs, There were eight novel SNPs from UGT1A1, seven from UGT2B7, and 16 from UGT2B15, amounting to a total of 31 novel SNP discoveries (Supplementary Fig. 1, only online). One novel UGT2B7 SNP (673G>A, E225K) and five novel UGT2B15 SNPs (412A>G, M138V; 16461C>T, A398V; 16263G>T, A398A; 23269C>T, S483L; 23403G>A, V494V) were located in coding regions.
The LD structures of the three genes in the study were inferred by only using SNPs with detectable minor allele frequencies (MAFs) in at least one ethnic group, and the results were shown in Supplementary Fig. 2 (only online). Overall, MAFs of most SNPs were not high enough to form distinct LD structures or haplotype blocks. However, Asian ethnic groups (Korean, Han Chinese, and Japanese) displayed similar LD structures when compared with African American or European American subjects.
Generally, MAFs of Asian ethnic groups were similar, while MAFs of African American and European American groups deviated from Asians in several SNPs (Table 1, Supplementary Fig. 3, only online). Among the numerous SNPs of UGT1A1, which showed MAF differences among ethnic groups, *6 (rs4148323), *60 (rs4124874), and *93 (rs10929302) are known as core markers of UGT1A1. In all three SNPs, MAFs of Asians were similar, while those in European American and African American subjects were either significantly higher or lower than those in Asians. In UGT2B7, the MAF of core marker *2 (rs7439366) was higher in European Americans than in Asians, while it was monomorphic in African Americans. On the other hand, frequency differences in a part of the *3 (rs12233719) allele were not as drastic, but MAFs in Koreans were higher than those in other ethnic groups. In UGT2B15, MAFs of the core marker *2 (rs1902023) in Koreans and Japanese were larger than those in Han Chinese, African Americans, and European Americans. Detailed information of each gene's core markers are summarized in Table 2, with their position, allele change, amino acid change, star allele nomenclature, MAF, and any known roles in pharmacogenetics. Three core markers of UGT1A1,*7 (rs34993780), *28 (rs8175347), and *29 (rs55750087), were monomorphic in all studied ethnic groups, and thus not shown in any results. We conducted Fisher's exact test to find statistically significant differences in allele frequencies of core markers between Koreans and other populations (Supplementary Table 2, only online).
In order to predict the function of the novel SNPs detected in our study, we conducted in silico analysis using the Fast-SNP program. The program analyzes for a potential transcription factor binding site change caused by a promoter SNP. As a result, in UGT1A1, -690G>A was estimated to induce Ras responsive element binding protein 1 (RREB1) and specificity protein 1 (Sp1) binding sites onto the promoter, while -689A>C could lead to an additional binding site for Sp1 only (data not shown).
In the field of pharmacogenetics, studying the frequency differences of core markers across various ethnic groups is important for personalized drug treatment. Therefore, the present study analyzed the SNPs of UGT family genes UGT1A1, UGT2B7, and UGT2B15 across five ethnic groups. Although the LD structures of the three genes did not exhibit much difference between Asians and other ethnic groups, the MAFs of several SNPs differed depending on ethnicity. Interestingly, most of the SNPs with large MAF discrepancies among ethnic groups were pharmacogenetic core markers of each gene.
From our result, three UGT1A1 core markers, *6 (rs4148323), *60 (rs4124874), and *93 (rs10929302), showed large MAF differences among ethnic groups. Previously, researchers found *6 (rs4148323), *28 (rs8175347), and *60 (rs4124874) to influence the expression levels of UGT1A1, and discrepancies in frequency across ethnic groups led to varying effects of irinotecan application depending on the ethnicity.20-22 In addition, a study of a Japanese population reported that *27 (rs35350960), a genetic variant unique to Japanese subjects, could increase susceptibility to irinotecan side effects.23 Although we failed to detect *27 (rs35350960) or *28 (rs8175347) in the present study, higher MAFs of *6 (rs4148323) and *60 (rs4124874) in Asian ethnic groups suggest that the two alleles could be important pharmacogenetic factors for irinotecan application in Korean and other Asian cancer patients.
As a core marker for UGT2B7, *2 (rs7439366) is known to be related with Vadimezan (5,6-dimethylxanthenone-4-acetic acid, previously called DMXAA), an anti-cancer drug.24 A previous study reported that there was a five-fold inter-individual variation in UGT2B7 expression level in the liver.25 Subsequently, such variation led to differing degrees of reaction to Vadimezan individually, as reduced enzyme expression caused by inhibition of Vadimezan metabolism. In our study's results, the MAF of *2 (rs7439366) was considerably lower in Asians compared to Caucasians, while a part of UGT2B7 *3 (rs12233719) was polymorphic in Asian only. Therefore, we suspect that higher MAF of *2 (rs7439366) in Europeans may lead to increased resistance to Vadimezan clearance in Caucasians, while *3 (rs12233719) may cause increased resistance in Asians.
UGT2B15 is known to glucuronidate drugs such as oxazepam, lorazepam, and rofecoxib.14,26,27 Its core marker *2 (rs1902023) has been linked with varying degrees of reaction toward the drugs. The minor allele of *2 (rs1902023) was found to lower the glucuronidation level of oxazepam in the liver, while also lowering the systemic clearance of lorazepam.14,15 Such studies have shown that *2 (rs1902023) could be used as a pharmacogenetic marker to personalize treatments with oxazepam or lorazepam. In the present study, the MAFs of *2 (rs1902023) varied across the five studied ethnic groups. Interestingly, whereas other investigated SNPs often showed clear MAF differences between Asian and other ethnic groups, *2 (rs1902023) showed differences among Asian ethnic groups as well. While Koreans and Japanese showed higher MAFs than European Americans (MAF=0.484, 0.436, and 0.298, respectively), Han Chinese MAFs were similar to those for European Americans (MAF=0.289). The result suggests that Korean and Japanese ethnic groups tend to possess increased resistance toward lorazepam or oxazepam clearance in comparison to other ethnic groups.
In the present study the direct sequencing of UGT1A1, UGT2B7, and UGT2B15 yielded a number of novel SNPs for each gene. We performed additional in silico analyses to predict the function of these thirty two novel SNPs. As a result, a couple of SNPs located in the promoter region of UGT1A1 were simulated to change transcription factor (TF) binding sites. As stated above, -690G>A induced RREB1 and Sp1 binding sites, and -689A>C only introduced Sp1 binding site. RREB1 binds to Ras-responsive elements (RREs) of gene promoters has been shown to increase the expression of the bound gene.28 In a previous study, calcitonin (CT) with RREB1 bound to its promoter showed an increased expression of CT promoter-reporter construct during Ras- or Raf-induced differentiation, which subsequently suggested that RREB1 might play a role in Ras/Raf signal transduction in medullary thyroid cancer.28 Although no study has reported a binding of RREB1 to UGT1A1, our in silico analysis results suggest that the binding of RREB1 might cause expression change of UGT1A1, which could affect the overall function of the gene.
Another predicted TF, Sp1 is known to bind at GC-rich promoter sites and enhance gene transcription. It has been associated with various forms of cancer and drug treatments.29,30 Furthermore, other UGT family members such as UGT1A4, 8, 9, and 10 are known to have a binding site for Sp1 and are regulated by it.31,32 Therefore, the discovery of a potential binding site for Sp1 in UGT1A1 could suggest a new pathway of how Sp1 might increase cancer risk or drug delivery.
Besides the discovery of promoter SNPs, we also found exonic SNPs that induced amino acid change. In UGT2B7, 673G>A caused an amino acid change of Glu225Lys. A previous study showed that such amino acid change, which reverses the amino acid property from acidic to basic, caused a conformational change of the protein structure.33 Among the six novel exonic SNPs of UGT2B15, 2552T>C (Cys283 Arg) and 24269C>T (Ser483Leu) were found to change the amino acid properties. Cys283Arg showed an amino acid property change from polar to basic, while the amino acid property of Ser483Leu was modified from polar to nonpolar. Such amino acid substitutions have been shown to result in significant changes in the protein structures in previous studies.34,35 Therefore, the three exonic SNPs listed above could cause structural modification of proteins, although functional studies would be required to confirm our results.
Although our study sequenced three UGT family genes to examine frequency differences across different ethnic groups and discover novel SNPs, several limitations are present in the study. First, we did not detect core SNPs with low frequencies, such as UGT1A1 *28 (rs8175347), because of the small number of samples. The small number of samples also may skew the results in this study. Also, although samples originated from five different ethnic groups, a majority of samples were of Asian descent, which might have limited the effectiveness of the population study. Lastly, no functional study was conducted to further confirm the roles of the SNPs, although in silico analyses were performed to compensate for the lack of functional analysis to an extent.
In summary, the present study analyzed UGT1A1, UGT2B7, and UGT2B15 by directly sequencing 288 subjects from five ethnic groups. As a result, core markers for each gene showed significant MAF differences from Asians in comparison to other population. Furthermore, we discovered 31 novel SNPs and predicted the function of the newly discovered SNPs by in silico analyses. Our results offer new insight into the pharmacogenetic role of UGT1A1, UGT2B7, and UGT2B15 in various ethnicities as well as potential novel therapeutic targets with the discovery of novel SNPs.
Figures and Tables
Table 1
MAF of 87 SNPs from UGT1A1, UGT2B7, and UGT2B15
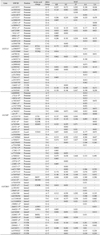
MAF, minor allele frequency; KR, Korean; HC, Han Chinese; JP, Japanese; AA, African American; EA, European American; UTR, untranslated region; SNP, single nucleotide polymorphism.
"-" indicates the SNP was monomorphic in the particular ethnic group.
*SNPs indicate the novel SNPs discovered in our study.
ACKNOWLEDGEMENTS
This research was supported by a grant (11182KFDA658) from Korea Food & Drug Administration in 2011.
References
1. King CD, Rios GR, Green MD, Tephly TR. UDP-glucuronosyltransferases. Curr Drug Metab. 2000; 1:143–161.


2. Liston HL, Markowitz JS, DeVane CL. Drug glucuronidation in clinical psychopharmacology. J Clin Psychopharmacol. 2001; 21:500–515.


3. Baranano DE, Rao M, Ferris CD, Snyder SH. Biliverdin reductase: a major physiologic cytoprotectant. Proc Natl Acad Sci U S A. 2002; 99:16093–16098.


4. Lacko M, Voogd AC, Roelofs HM, te Morsche RH, Oude Ophuis MB, Peters WH, et al. Combined effect of genetic polymorphisms in phase I and II biotransformation enzymes on head and neck cancer risk. Head Neck. 2013; 35:858–867.


5. Bajro MH, Josifovski T, Panovski M, Jankulovski N, Nestorovska AK, Matevska N, et al. Promoter length polymorphism in UGT1A1 and the risk of sporadic colorectal cancer. Cancer Genet. 2012; 205:163–167.


6. Gil J, Sąsiadek MM. Gilbert syndrome: the UGT1A1*28 promoter polymorphism as a biomarker of multifactorial diseases and drug metabolism. Biomark Med. 2012; 6:223–230.


7. Lin R, Wang Y, Wang Y, Fu W, Zhang D, Zheng H, et al. Common variants of four bilirubin metabolism genes and their association with serum bilirubin and coronary artery disease in Chinese Han population. Pharmacogenet Genomics. 2009; 19:310–318.


8. Mackenzie P, Little JM, Radominska-Pandya A. Glucosidation of hyodeoxycholic acid by UDP-glucuronosyltransferase 2B7. Biochem Pharmacol. 2003; 65:417–421.


9. Barre L, Fournel-Gigleux S, Finel M, Netter P, Magdalou J, Ouzzine M. Substrate specificity of the human UDP-glucuronosyltransferase UGT2B4 and UGT2B7. Identification of a critical aromatic amino acid residue at position 33. FEBS J. 2007; 274:1256–1264.


10. Lin GF, Guo WC, Chen JG, Qin YQ, Golka K, Xiang CQ, et al. An association of UDP-glucuronosyltransferase 2B7 C802T (His268Tyr) polymorphism with bladder cancer in benzidine-exposed workers in China. Toxicol Sci. 2005; 85:502–506.


11. van der Logt EM, te Morsche RH, Groenendaal N, Roelofs HM, de Metz M, van der Stappen JW, et al. Genetic polymorphism in UDP-glucuronosyltransferase 2B7 and colorectal cancer risk. Oncol Res. 2009; 17:323–329.


12. Parmar S, Stingl JC, Huber-Wechselberger A, Kainz A, Renner W, Langsenlehner U, et al. Impact of UGT2B7 His268Tyr polymorphism on the outcome of adjuvant epirubicin treatment in breast cancer. Breast Cancer Res. 2011; 13:R57.


13. Green MD, Oturu EM, Tephly TR. Stable expression of a human liver UDP-glucuronosyltransferase (UGT2B15) with activity toward steroid and xenobiotic substrates. Drug Metab Dispos. 1994; 22:799–805.
14. Chung JY, Cho JY, Yu KS, Kim JR, Jung HR, Lim KS, et al. Effect of the UGT2B15 genotype on the pharmacokinetics, pharmacodynamics, and drug interactions of intravenous lorazepam in healthy volunteers. Clin Pharmacol Ther. 2005; 77:486–494.


15. Court MH, Hao Q, Krishnaswamy S, Bekaii-Saab T, Al-Rohaimi A, von Moltke LL, et al. UDP-glucuronosyltransferase (UGT) 2B15 pharmacogenetics: UGT2B15 D85Y genotype and gender are major determinants of oxazepam glucuronidation by human liver. J Pharmacol Exp Ther. 2004; 310:656–665.


16. Rozen S, Skaletsky H. Primer3 on the WWW for general users and for biologist programmers. Methods Mol Biol. 2000; 132:365–386.


17. Devlin B, Risch N. A comparison of linkage disequilibrium measures for fine-scale mapping. Genomics. 1995; 29:311–322.


18. Barrett JC, Fry B, Maller J, Daly MJ. Haploview: analysis and visualization of LD and haplotype maps. Bioinformatics. 2005; 21:263–265.


19. Yuan HY, Chiou JJ, Tseng WH, Liu CH, Liu CK, Lin YJ, et al. FASTSNP: an always up-to-date and extendable service for SNP function analysis and prioritization. Nucleic Acids Res. 2006; 34(Web Server issue):W635–W641.


20. Sugatani J, Yamakawa K, Yoshinari K, Machida T, Takagi H, Mori M, et al. Identification of a defect in the UGT1A1 gene promoter and its association with hyperbilirubinemia. Biochem Biophys Res Commun. 2002; 292:492–497.


21. Gagné JF, Montminy V, Belanger P, Journault K, Gaucher G, Guillemette C. Common human UGT1A polymorphisms and the altered metabolism of irinotecan active metabolite 7-ethyl-10-hydroxycamptothecin (SN-38). Mol Pharmacol. 2002; 62:608–617.


22. Jinno H, Tanaka-Kagawa T, Hanioka N, Saeki M, Ishida S, Nishimura T, et al. Glucuronidation of 7-ethyl-10-hydroxycamptothecin (SN-38), an active metabolite of irinotecan (CPT-11), by human UGT1A1 variants, G71R, P229Q, and Y486D. Drug Metab Dispos. 2003; 31:108–113.


23. Ando Y, Saka H, Ando M, Sawa T, Muro K, Ueoka H, et al. Polymorphisms of UDP-glucuronosyltransferase gene and irinotecan toxicity: a pharmacogenetic analysis. Cancer Res. 2000; 60:6921–6926.
24. Miners JO, Valente L, Lillywhite KJ, Mackenzie PI, Burchell B, Baguley BC, et al. Preclinical prediction of factors influencing the elimination of 5,6-dimethylxanthenone-4-acetic acid, a new anticancer drug. Cancer Res. 1997; 57:284–289.
25. Zhou S, Kestell P, Baguley BC, Paxton JW. Preclinical factors affecting the interindividual variability in the clearance of the investigational anti-cancer drug 5,6-dimethylxanthenone-4-acetic acid. Biochem Pharmacol. 2003; 65:1853–1865.


26. Zhang JY, Zhan J, Cook CS, Ings RM, Breau AP. Involvement of human UGT2B7 and 2B15 in rofecoxib metabolism. Drug Metab Dispos. 2003; 31:652–658.


27. Court MH, Duan SX, Guillemette C, Journault K, Krishnaswamy S, Von Moltke LL, et al. Stereoselective conjugation of oxazepam by human UDP-glucuronosyltransferases (UGTs): S-oxazepam is glucuronidated by UGT2B15, while R-oxazepam is glucuronidated by UGT2B7 and UGT1A9. Drug Metab Dispos. 2002; 30:1257–1265.


28. Thiagalingam A, De Bustros A, Borges M, Jasti R, Compton D, Diamond L, et al. RREB-1, a novel zinc finger protein, is involved in the differentiation response to Ras in human medullary thyroid carcinomas. Mol Cell Biol. 1996; 16:5335–5345.


29. Yuan P, Wang L, Wei D, Zhang J, Jia Z, Li Q, et al. Therapeutic inhibition of Sp1 expression in growing tumors by mithramycin a correlates directly with potent antiangiogenic effects on human pancreatic cancer. Cancer. 2007; 110:2682–2690.


30. Kim K, Chadalapaka G, Pathi SS, Jin UH, Lee JS, Park YY, et al. Induction of the transcriptional repressor ZBTB4 in prostate cancer cells by drug-induced targeting of microRNA-17-92/106b-25 clusters. Mol Cancer Ther. 2012; 11:1852–1862.


31. Chen H, Yang K, Choi S, Fischer JH, Jeong H. Up-regulation of UDP-glucuronosyltransferase (UGT) 1A4 by 17beta-estradiol: a potential mechanism of increased lamotrigine elimination in pregnancy. Drug Metab Dispos. 2009; 37:1841–1847.


32. Gregory PA, Lewinsky RH, Gardner-Stephen DA, Mackenzie PI. Regulation of UDP glucuronosyltransferases in the gastrointestinal tract. Toxicol Appl Pharmacol. 2004; 199:354–363.


33. Takahashi N, Takahashi Y, Blumberg BS, Putnam FW. Amino acid substitutions in genetic variants of human serum albumin and in sequences inferred from molecular cloning. Proc Natl Acad Sci U S A. 1987; 84:4413–4417.


34. Hishinuma A, Kasai K, Masawa N, Kanno Y, Arimura M, Shimoda SI, et al. Missense mutation (C1263R) in the thyroglobulin gene causes congenital goiter with mild hypothyroidism by impaired intracellular transport. Endocr J. 1998; 45:315–327.


35. Mendel D, Ellman JA, Chang Z, Veenstra DL, Kollman PA, Schultz PG. Probing protein stability with unnatural amino acids. Science. 1992; 256:1798–1802.


36. Bosma PJ, Chowdhury JR, Bakker C, Gantla S, de Boer A, Oostra BA, et al. The genetic basis of the reduced expression of bilirubin UDP-glucuronosyltransferase 1 in Gilbert's syndrome. N Engl J Med. 1995; 333:1171–1175.


37. Swen JJ, Nijenhuis M, de Boer A, Grandia L, Maitland-van der Zee AH, Mulder H, et al. Pharmacogenetics: from bench to byte--an update of guidelines. Clin Pharmacol Ther. 2011; 89:662–673.


38. Chen G, Ramos E, Adeyemo A, Shriner D, Zhou J, Doumatey AP, et al. UGT1A1 is a major locus influencing bilirubin levels in African Americans. Eur J Hum Genet. 2012; 20:463–468.


39. Aono S, Yamada Y, Keino H, Hanada N, Nakagawa T, Sasaoka Y, et al. Identification of defect in the genes for bilirubin UDP-glucuronosyl-transferase in a patient with Crigler-Najjar syndrome type II. Biochem Biophys Res Commun. 1993; 197:1239–1244.


40. Aono S, Adachi Y, Uyama E, Yamada Y, Keino H, Nanno T, et al. Analysis of genes for bilirubin UDP-glucuronosyltransferase in Gilbert's syndrome. Lancet. 1995; 345:958–959.


41. Saeki M, Saito Y, Jinno H, Tanaka-Kagawa T, Ohno A, Ozawa S, et al. Single nucleotide polymorphisms and haplotype frequencies of UGT2B4 and UGT2B7 in a Japanese population. Drug Metab Dispos. 2004; 32:1048–1054.
Supplementary Material
Supplementary Fig. 1
Chromatograms of novel SNPs for (A) UGT1A1, (B) UGT2B7, and (C) UGT2B15. SNP, single nucleotide polymorphism.
Supplementary Fig. 2
LD blocks of UGT1A1, UGT2B7, and UGT2B15 in Korean, African American, European American, Han Chinese, and Japanese subjects. Haplotype blocks are shown with separate black lines. LD, linkage disequilibrium.
Supplementary Fig. 3
A physical map and MAF of UGT1A1, UGT2B7, and UGT2B15 SNPs among Korean, African American, European American, Han Chinese, and Japanese subjects. Bold SNPs indicate pharmacogenetic core SNPs. Novel SNPs are labeled with their location and allele change. MAF, minor allele frequency; SNP, single nucleotide polymorphism; Ex, Exon.